lect5.pdf
Document Details
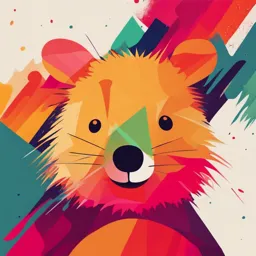
Uploaded by SubsidizedPlatypus4725
Al-Najah University
Tags
Full Transcript
EXTERNAL COMPONENTS there are three main methods of x-ray tube support. i. Ceiling Support System The ceiling support system is probably the most frequently used. It consists of two perpendicular sets of ceiling-mounted rails. This allows for both longitudinal and transverse travel of the...
EXTERNAL COMPONENTS there are three main methods of x-ray tube support. i. Ceiling Support System The ceiling support system is probably the most frequently used. It consists of two perpendicular sets of ceiling-mounted rails. This allows for both longitudinal and transverse travel of the x-ray tube. When the x-ray tube is centered above the examination table at the standard SID, the x-ray tube is in a preferred detent position. ii. Floor-to-Ceiling Support System The floor-to-ceiling support system has a single column with rollers at each end, one attached to a ceilingmounted rail and the other attached to a floor-mounted rail. The x-ray tube slides up and down the column as the column rotates. A variation of this type of support system has the column positioned on a single floor support system with one or two floor- mounted rails. iii. C-Arm Support System Interventional radiology suites often are equipped with C-arm support systems, so called because the system is shaped like a C. These systems are ceiling mounted and provide for very flexible x-ray tube positioning. The image receptor is attached to the other end of the C-arm from the x-ray tube. Variations called L-arm or U-arm support are also common. Protective Housing When x-rays are produced, they are emitted isotropically, that is, with equal intensity in all directions. through the special section of the x-ray tube called the window (Figure 6-3). The x-rays emitted through the window are called the useful beam. X-rays that escape through the protective housing are called leakage radiation; they contribute nothing in the way of diagnostic information and result in unnecessary exposure of the patient and the radiologic technologist. Properly designed protective housing reduces the level of leakage radiation to less than 1 mGya/hr at 1 m when operated at maximum conditions The protective housing incorporates specially designed high-voltage receptacles to protect against accidental electric shock. Death by electrocution was a very real hazard for early radiologic technologists. The protective housing also provides mechanical support for the x-ray tube and protects the tube from damage caused by rough handling. The protective housing around some x-ray tubes contains oil that serves as both an insulator against electric shock and as a thermal cushion to dissipate heat. Some protective housings have a cooling fan to air cool the tube or the oil in which the x-ray tube is immersed. A bellows-like device allows the oil to expand when heated. If the expansion is too great, a microswitch is activated, so the tube cannot be used until it cools. Glass or Metal Enclosure An x-ray tube is an electronic vacuum tube with components contained within a glass or metal enclosure. The x-ray tube, however, is a special type of vacuum tube that contains two electrodes: the cathode and the anode. It is relatively large, perhaps 30 to 50 cm long and 20 cm in diameter. The glass enclosure is made of Pyrex glass to enable it to withstand the tremendous heat generated. The enclosure maintains a vacuum inside the tube. This vacuum allows for more efficient x-ray production and a longer tube life. When just a little gas is in the enclosure, the electron flow from cathode to anode is reduced, fewer x rays are produced, and more heat is generated. X-ray tubes are designed with a glass or a metal enclosure As a glass enclosure tube ages, some tungsten vaporizes and coats the inside of the glass enclosure. This alters the electrical properties of the tube, allowing tube current to stray and interact with the glass enclosure; the result is arcing and tube failure. Metal enclosure tubes maintain a constant electric potential between the electrons of the tube current and the enclosure. Therefore, they have a longer life and are less likely to fail. Virtually all high-capacity x-ray tubes now use metal enclosures. The x-ray tube window is an area of the glass or metal enclosure, approximately 5 cm2, that is thin and through which the useful beam of x-rays is emitted. Such a window allows maximum emission of x- rays with minimum absorption. INTERNAL COMPONENTS Cathode Figure 6-4 shows a photograph of a dual-filament cathode and a schematic drawing of its electric supply. The two filaments supply separate electron beams to produce two focal spots The cathode is the negative side of the x-ray tube; it has two primary parts, a filament and a focusing cup. Filament. The filament is a coil of wire similar to that in a kitchen toaster, but it is much smaller. The filament is approximately 2 mm in diameter and 1 or 2 cm long. An x-ray tube filament emits electrons when it is heated. When the current through the filament is sufficiently high, the outer-shell electrons of the filament atoms are “boiled off” and ejected from the filament. This phenomenon is known as thermionic emission. i. Filaments are usually made of thoriated tungsten. Tungsten provides for higher thermionic emission than other metals. ii. Its melting point is 3410°C; therefore, it is not likely to burn out like the filament of a light bulb. iii. tungsten does not vaporize easily. If it did, the tube would become gassy quickly, and its internal parts would be coated with tungsten. iv. The addition of 1% to 2% thorium to the tungsten filament enhances the efficiency of thermionic emission and prolongs tube life Tungsten metal does vaporize and is deposited on internal components. This upsets some of the electric characteristics of the tube and can cause arcing and lead to tube failure. Such malfunction is usually abrupt Tungsten vaporization with deposition on the inside of the glass enclosure is the most common cause of tube failure. tungsten metal does vaporize and is deposited on internal components. This upsets some of the electric characteristics of the tube and can cause arcing and lead to tube failure. Such malfunction is usually abrupt. Focusing Cup. The filament is embedded in a metal shroud called the focusing cup (Figure 6-5). Because all of the electrons accelerated from cathode to anode are electrically negative, the electron beam tends to spread out owing to electrostatic repulsion. The focusing cup is negatively charged so that it electrostatically confines the electron beam to a small area of the anode (Figure 6-6). The effectiveness of the focusing cup is determined by its size and shape, its charge, the filament size and shape, and the position of the filament in the focusing cup. Most rotating anode x-ray tubes have two filaments mounted in the cathode assemble “side by side,” creating large and small focal spot sizes. Filament Current. When the x-ray imaging system is first turned on, a low current passes through the filament to warm it and prepare it for the thermal jolt necessary for x-ray production. At low filament current, there is no tube current because the filament does not get hot enough for thermionic emission. When the filament current is high enough for thermionic emission, a small increase in filament current results in a large increase in x-ray tube current The relationship between filament current and x-ray tube current depends on the tube voltage (Figure 6-7). When emitted from the filament, electrons are in the vicinity of the filament before they are accelerated to the anode. Because these electrons carry negative charges, they repel one another and tend to form a cloud around the filament. This cloud of electrons, called a space charge, makes it difficult for subsequent electrons to be emitted by the filament because of electrostatic repulsion. This phenomenon is called the space charge effect. Thermionic emission at low kVp and high mA can be space charge limited. At any given filament current, the x-ray tube current rises with increasing voltage to a maximum value. A further increase in kVp does not result in a higher mA because all of the available electrons have been used. This is the saturation current. Most diagnostic x-ray tubes have two focal spots one large and the other small. The small focal spot is used when better spatial resolution is required. The large focal spot is used when large body parts are imaged and when other techniques that produce high heat are required Small focal spots range from 0.1 to 1 mm; large focal spots range from 0.3 to 2 mm. Each filament of a dual filament cathode assembly is embedded in the focusing cup (Figure 6-9). The small focal spot size is associated with the small filament and the large focal spot size with the large filament Selection of one or the other focal spot is usually made with the mA station selector on the operating console. Normally, either filament can be used with the lower mA station—approximately 300 mA or less. At approximately 400 mA and up, only the larger focal spot is allowed because the heat capacity of the anode could be exceeded if the small focal spot were used Anode The anode is the positive side of the x-ray tube. it conducts electricity and radiates heat and contains the target There are two types of anodes, stationary and rotating (Figure 6-10). Stationary anode x-ray tubes are used in dental x-ray imaging systems, some portable imaging systems, and other special-purpose units in which high tube current and power are not required. General-purpose x-ray tubes use the rotating anode because they must be capable of producing high-intensity x-ray beams in a short time. The anode serves three functions in an x-ray tube. i. The anode is an electrical conductor. It receives electrons emitted by the cathode and conducts them through the tube to the connecting cables and back to the highvoltage generator. ii. The anode also provides mechanical support for the target. iii. The anode also must be a good thermal dissipater. When the projectile electrons from the cathode interact with the anode, more than 99% of their kinetic energy is converted into heat. This heat must be dissipated quickly. Copper, molybdenum, and graphite are the most common anode materials. Target The target is the area of the anode struck by the electrons from the cathode. In stationary anode tubes, the target consists of a tungsten alloy embedded in the copper anode (Figure 6-11, A). In rotating anode tubes, the entire rotating disc is the target (Figure 6-11, B). Alloying the tungsten (usually with rhenium) gives it added mechanical strength to withstand the stresses of high-speed rotation and the effects of repetitive thermal expansion and contraction. High-capacity x-ray tubes have molybdenum or graphite layered under the tungsten target (Figure 6-12). Both molybdenum and graphite have lower mass density than tungsten, making the anode lighter and easier to rotate Specialty x-ray tubes for mammography have molybdenum or rhodium targets principally because of their low atomic number and low K-characteristic x-ray energy Tungsten is the material of choice for the target for general radiography for three main reasons: i. Atomic number—Tungsten’s high atomic number, 74, results in high-efficiency x-ray production and in high-energy x-rays. ii. Thermal conductivity—Tungsten has a thermal conductivity nearly equal to that of copper. It is therefore an efficient metal for dissipating the heat produced. iii. High melting point—Any material, if heated sufficiently, will melt and become liquid. Tungsten has a high melting point (3400°C compared with 1100°C for copper Rotating Anode The rotating anode x-ray tube allows the electron beam to interact with a much larger target area; therefore, the heating of the anode is not confined to one small spot, as in a stationary anode tube. the target areas of typical stationary anode (4 mm2) and rotating anode(1800 mm2 ) x-ray tubes with 1-mm focal spots. Thus, the rotating anode tube provides nearly 500 times more area to interact with the electron beam than is provided by a stationary anode tube Higher tube currents and shorter exposure times are possible with the rotating anode. Most rotating anodes revolve at 3400 rpm (revolutions per minute). The Anode so of high capacity x-ray tubes rotate at 10,000 rpm. The stem of the anode is the shaft between the anode and the rotor. It is narrow so as to reduce its thermal conductivity. The stem usually is made of molybdenum because it is a poor heat conductor. The rotating anode is powered by an electromagnetic induction motor. When the radiologic technologist pushes the exposure button of a radiographic imaging system, there a short delay before an exposure is made. This allows the rotor to accelerate to its designated rpm while the filament is heated. Only then is the kVp applied to the x-ray tube. During this time, filament current is increased to provide the correct x-ray tube current. Line-Focus Principle. The focal spot is the area of the target from which x-rays are emitted. Radiology requires small focal spots because the smaller the focal spot, the better the spatial resolution of the image. Line-focus principle is design to allow a large area for heating while maintaining a small focal spot. By angling the target (Figure 6-17), one makes the effective area of the target much smaller than the actual area of electron interaction. The effective target area, or effective focal spot size, is the area projected onto the patient and the image receptor. When the target angle is made smaller, the effective focal spot size also is made smaller. Diagnostic x-ray tubes have target angles that vary from approximately 5 to 20 degrees. Biangular targets are available that produce two focal spot sizes because of two different target angles on the anode (Figure 6-18). Combining biangular targets with different-length filaments results in a very flexible combination Heel Effect is the consequence of the line-focus principle is that the radiation intensity on the cathode side of the x-ray field is greater than that on the anode side. Electrons interact with target atoms at various depths into the target. The x-rays that constitute the useful beam emitted toward the anode side must traverse a greater thickness of target material than the x-rays emitted toward the cathode direction (Figure 6-20). The intensity of x-rays that are emitted through the “heel” of the target is reduced because they have a longer path through the target and therefore increased absorption. This is the heel effect The smaller the anode angle, the larger the heel effect. The difference in radiation intensity across the useful beam of an x- ray field can vary by as much as 45%. The heel effect is important when one is imaging anatomical structures that differ greatly in thickness or mass density. In general, positioning the cathode side of the x-ray tube over the thicker part of the anatomy provides more uniform radiation exposure of the image receptor. In mammography, the x-ray tube is designed so that the more intense side of the x-ray beam, the cathode side, is positioned toward the chest wall. With angling of the x-ray tube, advantage can be taken of the foreshortening that occurs to the focal spot size, resulting in an even smaller effective focal spot size. The effective focal spot is smaller on the anode side of the x-ray field than on the cathode side (Figure 6-22). Off-Focus Radiation. X-ray tubes are designed so that projectile electrons from the cathode interact with the target only at the focal spot. However, some of the electrons bounce off the focal spot and then land on other areas of the target, causing x-rays to be produced from outside of the focal spot (Figure 6-23). These x-rays are called off-focus radiation. Off-focus radiation is undesirable because it extends the size of the focal spot. The additional x-ray beam area increases skin dose modestly but unnecessarily. Off-focus radiation can significantly reduce image contrast. X-RAY TUBE FAILURE The length of x-ray tube life is primarily under the control of radiologic technologists. Basically, x-ray tube life is extended by using the minimum radiographic factors of mA, kVp, and exposure time that are appropriate for each examination. The use of faster image receptors results in longer tube life. X-ray tube failure has several causes, most of which are related to the thermal characteristics of the x-ray tube. Enormous heat is generated in the anode of the x-ray tube during x-ray exposure. This heat can be dissipated in one of three ways: i. Radiation ii. Conduction iii. convection (Figure 6-25). A second type of x-ray tube failure results from maintaining the anode at elevated temperatures for prolonged periods. During exposures lasting 1 to 3 s, the temperature of the anode may be sufficient to cause it to glow like an incandescent light bulb. During exposure, heat is dissipated by radiation. Between exposures, heat is dissipated, primarily through conduction, to the oil bath in which the tube is immersed. Some heat is conducted through the narrow molybdenum neck to the rotor assembly; this can cause subsequent heating of the rotor bearings. Excessive heating of the bearings results in increased rotational friction and an imbalance of the rotor anode assembly. Bearing damage is an other cause of tube failure. Final cause of tube failure involves the filament. With excessive heating of the filament caused by high mA operation for prolonged periods, more tungsten is vaporized. The filament wire becomes thinner and eventually breaks, producing an open filament. This same type of failure occurs when an incandescent light bulb burns out Most CT tubes are now guaranteed for 50,000 exposures Question: A7-MHUhelicalCTx-raytube is guaranteed for 50,000 scans, each scan limited to 5 s. What is the x-ray tube life in hours? RATING CHARTS Radiologic technologists are guided in the use of x-ray tubes by x-ray tube rating charts. It is essential that technologists be able to read and understand these charts even though many of these charts are now digitally stored in the operating console. Three types of x-ray tube rating charts are particularly important: i. The radiographic rating chart ii. The anode cooling chart iii. The housing cooling chart For a given mA, any combination of kVp and time that lies below the mA curve is safe. Any combination of kVp and time that lies above the curve representing the desired mA is unsafe. If an unsafe exposure was made, the tube might fail abruptly. Most x-ray imaging systems have a microprocessor control that does not allow an unsafe exposure Question: With reference to Figure 6-26, which of the following conditions of exposure are safe, and which are unsafe? Question: Radiographic examination of the abdomen with a tube that has a 0.6-mm focal spot and anode rotation of 10,000 rpm requires technique factors of 95 kVp, 150 mAs. What is the shortest possible exposure time for this examination? Anode Cooling Chart The anode has a limited capacity for storing heat. Although heat is dissipated to the oil bath and x-ray tube housing, it is possible through prolonged use or multiple exposures to exceed the heat storage capacity of the anode. In x-ray applications, thermal energy is measured in heat units (HUs) or Joules (J). One heat unit is equal to the product of 1 kVp, 1 mA, and 1 s. One heat unit is also equal to 1.4 J. Calories and British thermal units (BTUs) are other familiar thermal energy units Single Phase HU = kVp × mA × s = 0.7 J Question: Radiographic examination of the lateral lumbar spine with a single-phase imaging system requires 98 kVp, 120 mAs. How many heat units are generated by this exposure? Question: A fluoroscopic examination is performed with a single-phase imaging system at 76 kVp and 1.5 mA for 3.5 min. How many heat units are generated? Three Phase/High Frequency HU = 1.4 × kVp × mA × s = 1 J Question: Six sequential skull films are exposed with a three-phase generator operated at 82 kVp, 120 mAs. What is the total heat generated? The thermal capacity of an anode, and its heat dissipation characteristics are contained in a rating chart called an anode cooling chart (Figure 6-27). Different from the radiographic rating chart, the anode cooling chart does not depend on the filament size or the speed of rotation. The tube represented in Figure 6-27 has a maximum anode heat capacity of 350,000 HU. The chart shows that if the maximum heat load were attained, it would take 15 minutes for the anode to cool completely. Question: A particular examination results in delivery of 50,000 HU to the anode in a matter of seconds. How long will it take the anode to cool completely? Question: How much heat energy (in joules) is produced during a single high-frequency mammographic exposure of 25 kVp, 200 mAs? Housing Cooling Chart The cooling chart for the housing of the x-ray tube has a shape similar to that of the anode cooling chart an is used in precisely the same way. Radiographic x-ray tube housings usually have maximum heat capacities in the range of several million heat units. Complete cooling after maximum heat capacity requires from 1 to 2 hours.