Muscular Tissue Lecture Notes PDF
Document Details
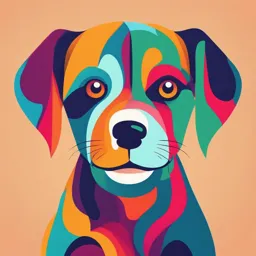
Uploaded by RefreshedAgate8599
Tags
Summary
These lecture notes cover the fascinating topic of muscular tissue, encompassing both macroscopic and microscopic anatomy, the crucial nerve-muscle relationship, and the mechanical process of skeletal muscle contraction. Illustrations and diagrams greatly enhance understanding of this complex biological system.
Full Transcript
Muscular Tissue CHAPTER 11 Clicker time……………… The approximate number of skeletal muscles in the body is a. 350 b. 500 c. 645 d. 715 Three very important concepts 1. Muscle anatomy - Macro and microscopic anatomy 2. The nerve-muscle relationship – The NMJ 3. Skeletal mus...
Muscular Tissue CHAPTER 11 Clicker time……………… The approximate number of skeletal muscles in the body is a. 350 b. 500 c. 645 d. 715 Three very important concepts 1. Muscle anatomy - Macro and microscopic anatomy 2. The nerve-muscle relationship – The NMJ 3. Skeletal muscle contraction – Sliding filament theory Microscopic Anatomy …The Muscle Fiber Sarcolemma—plasma membrane of a muscle fiber Sarcoplasm—cytoplasm of a muscle fiber Myofibrils—long protein bundles that occupy the main portion of the sarcoplasm ◦ Glycogen: stored in abundance to provide energy with heightened exercise ◦ Myoglobin: red pigment; stores oxygen needed for muscle activity The Muscle Fiber Multiple nuclei—flattened nuclei pressed against the inside of the sarcolemma ◦ Myoblasts: stem cells that fuse to form each muscle fiber ◦ Satellite cells: unspecialized myoblasts remaining between the muscle fiber and endomysium ◦ May multiply and produce new muscle fibers to some degree Mitochondria—packed into spaces between myofibrils 11-7 The Muscle Fiber Sarcoplasmic reticulum (SR)—smooth ER that forms a network around each myofibril: calcium reservoir ◦ Calcium activates the muscle contraction process Terminal cisternae—dilated end-sacs of SR which cross the muscle fiber from one side to the other T tubules—tubular infoldings of the sarcolemma which penetrate through the cell and emerge on the other side Triad—a T tubule and two terminal cisterns 11-8 The Muscle Fiber Copyright © The McGraw-Hill Companies, Inc. Permission required for reproduction or display. Muscle fiber Nucleus A band I band Z disc Mitochondria Openings into transverse tubules Sarcoplasmic reticulum Triad: Terminal cisternae Transverse tubule Sarcolemma Myofibrils Sarcoplasm Figure 11.2 Myofilaments 11-9 Myofilaments Copyright © The McGraw-Hill Companies, Inc. Permission required for reproduction or display. Head Tail (a) Myosin molecule Myosin head (b) Thick filament Thick filaments—made of several hundred myosin molecules ◦ Shaped like a golf club ◦ Heads directed outward in a helical array around the bundle Myofilaments Thin filaments ◦ Fibrous (F) actin: two intertwined strands ◦ String of globular (G) actin subunits each with an active site that can bind to head of myosin molecule ◦ Tropomyosin molecules ◦ Each blocking six or seven active sites on G actin subunits ◦ Troponin molecule: small, calcium-binding protein on each tropomyosin molecule Copyright © The McGraw-Hill Companies, Inc. Permission required for reproduction or display. Tropomyosin Troponin complex G actin (c) Thin filament Myofilaments Elastic filaments ◦ Titin (connectin): huge, springy protein ◦ Flank each thick filament and anchor it to the Z disc ◦ Help stabilize the thick filament ◦ Center it between the thin filaments ◦ Prevent overstretching 11-12 Myofilaments Copyright © The McGraw-Hill Companies, Inc. Permission required for reproduction or display. Myosin head (b) Thick filament Tropomyosin Troponin complex G actin (c) Thin filament Contractile proteins—myosin and actin do the work Regulatory proteins—tropomyosin and troponin ◦ Like a switch that determines when the fiber can contract and when it cannot ◦ Contraction activated by release of calcium into sarcoplasm and its binding to troponin ◦ Troponin changes shape and moves tropomyosin off the active sites on actin 11-13 Myofilaments Copyright © The McGraw-Hill Companies, Inc. Permission required for reproduction or display. Thick filament Thin filament Bare zone (d) Portion of a sarcomere showing the overlap of thick and thin filaments 11-14 Striations Myosin and actin are proteins that occur in all cells ◦ Function in cellular motility, mitosis, transport of intracellular material Organized in a precise way in skeletal and cardiac muscle Copyright © The McGraw-Hill Companies, Inc. Permission required for reproduction or display. Sarcomere I band A band I band H band Thick filament Thin filament M line Titin Z disc Elastic filament Z disc (b) Striations ◦ A band: dark; A stands for anisotropic ◦ Part of A band where thick and thin filaments overlap is especially dark ◦ H band: middle of A band; thick filaments only ◦ M line: middle of H band ◦ I band: alternating lighter band; I stands for isotropic ◦ The way the bands reflect polarized light ◦ Z disc: provides anchorage for thin filaments and elastic filaments ◦ Bisects I band Copyright © The McGraw-Hill Companies, Inc. Permission required for reproduction or display. Sarcomere I band A band I band H band Thick filament Thin filament M line Titin Z disc Elastic filament Z disc (b) Striations Copyright © The McGraw-Hill Companies, Inc. Permission required for reproduction or display. Nucleus Sarcomere 5 Z disc M line Individual myofibrils 4 H band 3 I band A band I band 1 2 (a) Visuals Unlimited Sarcomere—segment from Z disc to Z disc ◦ Functional contractile unit of muscle fiber Muscle cells shorten because their individual sarcomeres shorten ◦ Z disc (Z lines) are pulled closer together as thick and thin filaments slide past each other Striations Neither thick nor thin filaments change length during shortening ◦ Only the amount of overlap changes During shortening dystrophin and linking proteins also pull on extracellular proteins ◦ Transfers pull to extracellular tissue Myofilaments At least 7 other accessory proteins present Dystrophin—most clinically Copyright © The McGraw-Hill Companies, Inc. Permission required for reproduction or display. important Endomysium Linking proteins ◦ Links actin in outermost myofilaments to transmembrane proteins and Basal lamina eventually to fibrous endomysium surrounding the entire muscle cell Sarcolemma ◦ Transfers forces of muscle contraction to connective tissue around muscle cell Dystrophin ◦ Genetic defects in dystrophin produce Thin filament disabling disease muscular dystrophy Thick filament Figure 11.4 Check Point 1 Review the unique features of macro and microscopic anatomy of skeletal muscle Skeletal muscle never contracts unless stimulated by a nerve The Nerve— Muscle If nerve connections are Relationship severed or poisoned, a muscle is paralyzed Denervation atrophy: shrinkage of paralyzed muscle when connection not restored Motor Neurons and Motor Units Somatic motor neurons—nerve cells whose cell bodies are in the brainstem and spinal cord that serve skeletal muscles Somatic motor fibers—their axons that lead to the skeletal muscle ◦ Each nerve fiber branches out to a number of muscle fibers ◦ Each muscle fiber is supplied by only one motor neuron Motor Neurons and Motor Units Copyright © The McGraw-Hill Companies, Inc. Permission required for reproduction or display. Spinal cord Motor unit—one nerve Muscle fibers of one motor fiber and all the muscle unit Motor neuron 1 fibers innervated by it Motor Dispersed throughout the muscle neuron 2 Contract in unison Produce weak contraction over Neuromuscular wide area junction Provides ability to sustain long-term Skeletal contraction as motor units take muscle turns contracting (postural control) fibers Effective contraction usually requires the contraction of several motor units at once Synapse—point where a nerve fiber meets its target cell The Neuromuscular junction Neuromuscular (NMJ)—when target cell is Junction a muscle fiber One nerve fiber stimulates the muscle fiber at several points within the NMJ The Neuromuscular Junction Synaptic knob—swollen end of nerve fiber Contains synaptic vesicles filled with acetylcholine (ACh) Synaptic cleft—tiny gap between synaptic knob and muscle sarcolemma Schwann cell envelops and isolates all of the NMJ from surrounding tissue fluid Synaptic vesicles undergo exocytosis releasing ACh into synaptic cleft The Neuromuscular Junction 50 million ACh receptors—proteins incorporated into muscle cell plasma membrane ◦ Junctional folds of sarcolemma beneath synaptic knob ◦ Increase surface area holding ACh receptors ◦ Lack of receptors leads to paralysis in disease myasthenia gravis ◦ http://www.ninds.nih.gov/disorders/myasthenia_gravis/de tail_myasthenia_gravis.htm The Neuromuscular Junction Basal lamina—thin layer of collagen and glycoprotein separates Schwann cell and entire muscle cell from surrounding tissues ◦ Contains acetylcholinesterase (AChE) that breaks down ACh after contraction causing relaxation The Neuromuscular Junction Copyright © The McGraw-Hill Companies, Inc. Permission required for reproduction or display. Motor nerve fiber Myelin Schwann cell Synaptic knob Basal lamina Synaptic vesicles (containing ACh) Sarcolemma Synaptic cleft Nucleus ACh receptor Junctional folds Nucleus Mitochondria Sarcoplasm Myofilaments (b) Electrically Excitable Cells Muscle fibers and neurons are electrically excitable cells ◦ Their plasma membrane exhibits voltage changes in response to stimulation Electrophysiology—the study of the electrical activity of cells Voltage (electrical potential)—a difference in electrical charge from one point to another Resting membrane potential—about −90 mV ◦ Maintained by sodium–potassium pump In an unstimulated (resting) cell ◦ There are more anions (negative ions) on the inside of the plasma membrane than on the outside ◦ The plasma membrane is electrically polarized (charged) ◦ There are excess sodium ions (Na+) in the Electrically extracellular fluid (ECF) ◦ There are excess potassium ions (K+) in the Excitable Cells intracellular fluid (ICF) ◦ Also in the ICF, there are anions such as proteins, nucleic acids, and phosphates that cannot penetrate the plasma membrane ◦ These anions make the inside of the plasma membrane negatively charged by comparison to its outer surface What is voltage? a. The electrical difference between 2 points Clicker b. Electrical potential time……………… c. The difference in electrical charge d. All of the above Neuromuscular Toxins and Paralysis Toxins that interfere with synaptic function can paralyze the muscles Some pesticides contain Bind to acetylcholinesterase and prevent it from degrading Ach cholinesterase Spastic paralysis: a state of continual contraction of the inhibitors muscles; possible suffocation Tetanus (lockjaw) is a Glycine in the spinal cord normally stops motor neurons from producing unwanted muscle contractions form of spastic paralysis Tetanus toxin blocks glycine release in the spinal cord and caused by toxin causes overstimulation and spastic paralysis of the Clostridium tetani muscles Flaccid paralysis—a state in which the muscles are limp and cannot contract ◦ Curare: compete with ACh for receptor sites, but do not stimulate the muscles Neuromuscular ◦ Plant poison used by South American natives to poison blowgun darts Toxins and Paralysis Botulism—type of food poisoning caused by a neuromuscular toxin secreted by the bacterium Clostridium botulinum ◦ Blocks release of ACh causing flaccid paralysis ◦ Botox cosmetic injections for wrinkle removal What is the neurotransmitter responsible for relaying the information at the neuromuscular junction? a. Epinephrine Clicker b. Dopamine c. Acetylcholine time……………… d. Norepinephrine e. Serotonin Which of the following events occurs first in the direct transmission of information from neuron to muscle? (at the neuromuscular junction?) A. Release of ACh to synaptic cleft Clicker B. Opening of calcium channels time……………… C. Muscle contraction D. Increase of intracellular calcium in synaptic knob E. Binding of ACh to receptors in sarcolemma Check Point 2 ❑Review the skeletal muscle-nerve relationship ❑How are nerves connected to muscles? ❑What happens at the NMJ that causes a skeletal muscle to contract Behavior of Skeletal Muscle Fibers Four major phases of contraction and relaxation ◦ Excitation ◦ The process in which nerve action potentials lead to muscle action potentials ◦ Excitation–contraction coupling ◦ Events that link the action potentials on the sarcolemma to activation of the myofilaments, thereby preparing them to contract ◦ Contraction ◦ Step in which the muscle fiber develops tension and may shorten ◦ Relaxation ◦ When its work is done, a muscle fiber relaxes and returns to its resting length Excitation Copyright © The McGraw-Hill Companies, Inc. Permission required for reproduction or display. Nerve signal Motor Ca2+ enters nerve synaptic knob fiber Sarcolemma Synaptic Synaptic knob vesicles ACh Synaptic cleft ACh receptors 1 Arrival of nerve signal 2 Acetylcholine (ACh) release Nerve signal opens voltage-gated calcium channels in synaptic knob Calcium stimulates exocytosis of ACh from synaptic vesicles ACh released into synaptic cleft Excitation Copyright © The McGraw-Hill Companies, Inc. Permission required for reproduction or display. Copyright © The McGraw-Hill Companies, Inc. Permission required for reproduction or display. ACh ACh K+ ACh receptor Sarcolemma Na+ 3 Binding of ACh to receptor 4 Opening of ligand-regulated ion gate; creation of end-plate potential Two ACh molecules bind to each receptor protein, opening Na+ and K+ channels Na+ enters; shifting RMP goes from −90 mV to +75 mV, then K+ exits and RMP returns to −90 mV; quick voltage shift is called an end-plate potential (EPP) Excitation Copyright © The McGraw-Hill Companies, Inc. Permission required for reproduction or display. K+ Plasma membrane of synaptic knob Na+ Voltage-regulated ion gates Sarcolemma 5 Opening of voltage-regulated ion gates; creation of action potentials Voltage change (EPP) in end-plate region opens nearby voltage-gated channels producing an action potential that spreads over muscle surface A toxin that contains a cholinesterase inhibitor will a. Inhibit degradation of ACh (acetylcholine) b. Cause a state of continual muscle Clicker contraction time……………… c. Spastic paralysis of muscles d. Block glycine release and cause overstimulation e. Do all of the above Please note that due to differing operating systems, some animations will not appear until the presentation is viewed in Presentation Mode (Slide Show view). You may see blank slides in the “Normal” or “Slide Sorter” views. All animations will appear after viewing in Presentation Mode and playing each animation. Most animations will require the latest version of the Flash Player, which is available at http://get.adobe.com/flashplayer. Excitation–Contraction Coupling Copyright © The McGraw-Hill Companies, Inc. Permission required for reproduction or display. Terminal T tubule cisterna of SR T tubule Sarcoplasmic reticulum Ca2+ Ca2+ 6 Action potentials propagated 7 Calcium released from down T tubules terminal cisternae Action potential spreads down into T tubules Opens voltage-gated ion channels in T tubules and Ca+2 channels in SR Ca+2 enters the cytosol Excitation–Contraction Coupling Copyright © The McGraw-Hill Companies, Inc. Permission required for reproduction or display. Active sites Troponin Ca2+ Tropomyosin Actin Thin filament Myosin Ca2+ 8 Binding of calcium 9 Shifting of tropomyosin; to troponin exposure of active sites on actin Calcium binds to troponin in thin filaments Troponin–tropomyosin complex changes shape and exposes active sites on actin Contraction Copyright © The McGraw-Hill Companies, Inc. Permission required for reproduction or display. Myosin ATPase enzyme in myosin head hydrolyzes Troponin Tropomyosin an ATP molecule ADP Activates the head Pi “cocking” it in an Myosin 10 Hydrolysis of ATP to ADP + Pi; extended position activation and cocking of myosin head ◦ ADP + Pi remain attached Head binds to actin active site forming a myosin– Cross-bridge: Actin Myosin actin cross-bridge 11 Formation of myosin–actin cross-bridge Contraction Copyright © The McGraw-Hill Companies, Inc. Permission required for reproduction or display. Myosin head releases ADP and Pi, flexes pulling thin filament past thick—power stroke ATP 13 Binding of new ATP; breaking of cross-bridge Upon binding more ATP, myosin releases actin and process is repeated ADP ADP ◦ Each head performs five power PPii strokes per second 12 Power stroke; sliding of thin filament over thick filament ◦ Each stroke utilizes one molecule of ATP Please note that due to differing operating systems, some animations will not appear until the presentation is viewed in Presentation Mode (Slide Show view). You may see blank slides in the “Normal” or “Slide Sorter” views. All animations will appear after viewing in Presentation Mode and playing each animation. Most animations will require the latest version of the Flash Player, which is available at http://get.adobe.com/flashplayer. Relaxation Copyright © The McGraw-Hill Companies, Inc. Permission required for reproduction or display. AChE ACh 14 Cessation of nervous stimulation and ACh release 15 ACh breakdown by acetylcholinesterase (AChE) Nerve stimulation and ACh release stop AChE breaks down ACh and fragments reabsorbed into synaptic knob Stimulation by ACh stops Relaxation Copyright © The McGraw-Hill Companies, Inc. Permission required for reproduction or display. Terminal cisterna of SR Ca2+ Ca2+ 16 Reabsorption of calcium ions by sarcoplasmic reticulum Ca+2 pumped back into SR by active transport Ca+2 binds to calsequestrin while in storage in SR ATP is needed for muscle relaxation as well as muscle contraction Relaxation Ca+2 Copyright © The McGraw-Hill Companies, Inc. Permission required for reproduction or display. removed from troponin is pumped back into SR Ca2+ Tropomyosin reblocks the active sites ADP Pi Ca2+ Muscle fiber ceases to produce or 17 Loss of calcium ions from troponin maintain tension Muscle fiber returns to its resting length Tropomyosin Due to recoil of elastic components and contraction of ATP antagonistic muscles 18 Return of tropomyosin to position blocking active sites of actin Please note that due to differing operating systems, some animations will not appear until the presentation is viewed in Presentation Mode (Slide Show view). You may see blank slides in the “Normal” or “Slide Sorter” views. All animations will appear after viewing in Presentation Mode and playing each animation. Most animations will require the latest version of the Flash Player, which is available at http://get.adobe.com/flashplayer. Rigor mortis—hardening of muscles and stiffening of body beginning 3 to 4 hours after death Deteriorating sarcoplasmic reticulum releases Ca+2 Deteriorating sarcolemma allows Ca+2 to enter cytosol Ca+2 activates myosin-actin cross-bridging Muscle contracts, but cannot relax Rigor Mortis Muscle relaxation requires ATP, and ATP production is no longer produced after death Fibers remain contracted until myofilaments begin to decay Rigor mortis peaks about 12 hours after death, then diminishes over the next 48 to 60 hours Check Point 3 ❑What is the mechanisms of skeletal muscle contraction? ❑What are the key elements responsible for skeletal muscle contraction? ❑What is the role of ATP in skeletal muscle contraction? ❑Sliding filament theory ATP sources All muscle contraction depends on ATP Muscle Oxygen metabolism ATP supply depends on availability of: Organic energy sources such as glucose and fatty acids ATP Sources Two main pathways of ATP synthesis ◦ Anaerobic fermentation ◦ Enables cells to produce ATP in the absence of oxygen ◦ Yields little ATP and toxic lactic acid, a major factor in muscle fatigue ◦ Aerobic respiration ◦ Produces far more ATP ◦ Less toxic end products (CO2 and water) ◦ Requires a continual supply of oxygen ATP Sources Copyright © The McGraw-Hill Companies, Inc. Permission required for reproduction or display. 0 10 seconds 40 seconds Repayment of Duration of exercise oxygen debt Mode of ATP synthesis Aerobic respiration Phosphagen Glycogen– Aerobic using oxygen from system lactic acid respiration myoglobin system supported by (anaerobic cardiopulmonary fermentation) function Short, intense exercise (100 m dash) Oxygen need is briefly supplied by myoglobin for a limited amount of aerobic respiration at onset—rapidly depleted Muscles meet most of ATP demand by borrowing phosphate groups (Pi) from other molecules and Immediate transferring them to ADP Energy Two enzyme systems control these phosphate transfers Myokinase: transfers Pi from one ADP to another, converting the latter to ATP Creatine kinase: obtains Pi from a phosphate- storage molecule creatine phosphate (CP) Fast-acting system that helps maintain the ATP level while other ATP-generating mechanisms are being activated Immediate Energy Copyright © The McGraw-Hill Companies, Inc. Permission required for reproduction or display. ADP ADP Pi Myokinase AMP ATP Creatine ADP phosphate Pi Creatine Creatine kinase ATP Immediate Energy Phosphagen system—ATP and CP collectively ◦ Provides nearly all energy used for short bursts of intense activity ◦ 1 minute of brisk walking ◦ 6 seconds of sprinting or fast swimming ◦ Important in activities requiring brief but maximum effort ◦ Football, baseball, and weightlifting Short-Term Energy As the phosphagen Muscles obtain glucose from blood and their own stored glycogen system is exhausted In the absence of oxygen, glycolysis can generate a net gain of 2 ATP muscles shift to anaerobic for every glucose molecule consumed Converts glucose to lactic acid fermentation Glycogen–lactic acid system—the pathway from glycogen to lactic acid Produces enough ATP for 30 to 40 seconds of maximum activity Long-Term Energy After 40 seconds or so, the respiratory and cardiovascular systems “catch up” and deliver oxygen to the muscles fast enough for aerobic respiration to meet most of the ATP demands Long-Term Energy Aerobic respiration produces 36 ATP per glucose ◦ Efficient means of meeting the ATP demands of prolonged exercise ◦ One’s rate of oxygen consumption rises for 3 to 4 minutes and levels off to a steady state in which aerobic ATP production keeps pace with demand Long-Term Energy Cont. ◦ Little lactic acid accumulates under steady-state conditions ◦ Depletion of glycogen and blood glucose, together with the loss of fluid and electrolytes through sweating, set limits on endurance and performance even when lactic acid does not Muscle fatigue—progressive weakness and loss of contractility from prolonged use of the muscles Repeated squeezing of rubber ball Holding textbook out level to the floor Fatigue and Fatigue is thought to result from: Endurance ATP synthesis declines as glycogen is consumed ATP shortage slows down the Na+–K+ pumps Compromises their ability to maintain the resting membrane potential and excitability of the muscle fibers Lactic acid lowers pH of sarcoplasm Inhibits enzymes involved in contraction, ATP synthesis, and other aspects of muscle function Fatigue and Endurance Fatigue is thought to result from (cont.): ◦ Release of K+ with each action potential causes the accumulation of extracellular K+ ◦ Hyperpolarizes the cell and makes the muscle fiber less excitable ◦ Motor nerve fibers use up their ACh ◦ Less capable of stimulating muscle fibers—junctional fatigue ◦ Central nervous system, where all motor commands originate, fatigues by unknown processes, so there is less signal output to the skeletal muscles Fatigue and Endurance Endurance—the ability to maintain high- intensity exercise for more than 4 to 5 minutes ◦ Determined in large part by one’s maximum oxygen uptake (VO2max) ◦ Maximum oxygen uptake: the point at which the rate of oxygen consumption reaches a plateau and does not increase further with an added workload ◦ Proportional to body size ◦ Peaks at around age 20 ◦ Usually greater in males than females ◦ Can be twice as great in trained endurance athletes as in untrained persons ◦ Results in twice the ATP production Beating Fatigue Taking oral creatine increases level of creatine phosphate in muscle tissue and increases speed of ATP regeneration ◦ Risks are not well known ◦ Muscle cramping, electrolyte imbalances, dehydration, water retention, stroke ◦ Kidney disease from overloading kidney with metabolite creatinine Carbohydrate loading—dietary regimen ◦ Packs extra glycogen into muscle cells ◦ Extra glycogen is hydrophilic and adds 2.7 g water per gram of glycogen ◦ Athletes feel sense of heaviness outweighs benefits of extra available glycogen Heavy breathing continues after strenuous exercise Excess postexercise oxygen consumption (EPOC): the difference between the resting rate of oxygen consumption and the elevated rate following exercise Oxygen Debt Needed for the following purposes: Replace oxygen reserves depleted in the first minute of exercise Replenishing the phosphagen system Oxidizing lactic acid Serving the elevated metabolic rate Physiological Classes of Muscle Fibers Slow oxidative (SO), slow-twitch, red, or type I fibers ◦ Abundant mitochondria, myoglobin, capillaries: deep red color ◦ Adapted for aerobic respiration and fatigue resistance ◦ Relative long twitch lasting about 100 ms ◦ Soleus of calf and postural muscles of the back Fast glycolytic (FG), fast-twitch, white, or type II fibers ◦ Fibers are well adapted for quick responses, but not for fatigue resistance Physiological ◦ Rich in enzymes of phosphagen and glycogen– Classes of lactic acid systems generate lactic acid, causing fatigue Muscle ◦ Poor in mitochondria, myoglobin, and blood Fibers capillaries which gives pale appearance ◦ SR releases and reabsorbs Ca2+ quickly so contractions are quicker (7.5 ms/twitch) ◦ Extrinsic eye muscles, gastrocnemius, and biceps brachii Cardiac Muscle Limited to the heart - it pumps blood Properties of cardiac muscle ◦ Contraction with regular rhythm ◦ Muscle cells of each chamber must contract in unison ◦ Contractions must last long enough to expel blood ◦ Must work in sleep or wakefulness, without fail, and without conscious attention ◦ Must be highly resistant to fatigue Characteristics of cardiac muscle cells ◦ Striated like skeletal muscle, but myocytes (cardiocytes) are shorter and thicker ◦ Each myocyte is joined to several others at the uneven, notched Cardiac Muscle linkages—intercalated discs ◦ Appear as thick, dark lines in stained tissue sections ◦ Electrical gap junctions allow each myocyte to directly stimulate its neighbors ◦ Mechanical junctions that keep the myocytes from pulling apart Sarcoplasmic reticulum less developed, but T tubules are larger and admit supplemental Ca2+ from the extracellular fluid Cardiac Muscle Damaged cardiac muscle cells repair by fibrosis A little mitosis observed following heart attacks Not in significant amounts to regenerate functional muscle Cardiac Muscle Can contract without need for nervous stimulation ◦ Contains a built-in pacemaker that rhythmically sets off a wave of electrical excitation ◦ Wave travels through the muscle and triggers contraction of heart chambers ◦ Autorhythmic: able to contract rhythmically and independently Autonomic nervous system does send nerve fibers to the heart Can increase or decrease heart rate and contraction strength Very slow twitches; does not exhibit quick twitches like skeletal muscle Cardiac Maintains tension for about 200 to 250 ms Muscle Gives the heart time to expel blood Uses aerobic respiration almost exclusively Rich in myoglobin and glycogen Has especially large mitochondria 25% of volume of cardiac muscle cell 2% of skeletal muscle cell with smaller mitochondria Smooth Muscle Sarcoplasmic reticulum is scanty and there are no T tubules Ca2+ needed for muscle contraction comes from the ECF by way of Ca2+ channels in the sarcolemma Some smooth muscles lack nerve supply, while others receive autonomic fibers, not somatic motor fibers as in skeletal muscle Injured smooth muscle regenerates well Types of Smooth Muscle Copyright © The McGraw-Hill Companies, Inc. Permission required for reproduction or display. Multiunit smooth muscle Autonomic nerve fibers ◦ Occurs in some of the largest arteries and pulmonary air passages, in piloerector muscles of hair follicle, and in the iris of the eye ◦ Autonomic innervation similar to skeletal Synapses muscle ◦ Terminal branches of a nerve fiber synapse with individual myocytes and form a motor unit ◦ Each motor unit contracts independently of the others (a) Multiunit smooth muscle Types of Smooth Muscle Single-unit smooth muscle Autonomic nerve fibers ◦ More widespread ◦ Occurs in most blood vessels, in the digestive, respiratory, urinary, and Varicosities reproductive tracts ◦ Also called visceral muscle ◦ Often in two layers: inner circular and outer longitudinal ◦ Myocytes of this cell type are electrically coupled to each other by Gap junctions gap junctions ◦ They directly stimulate each other and a large number of cells contract as a single unit (b) Single-unit smooth muscle Types of Smooth Muscle Copyright © The McGraw-Hill Companies, Inc. Permission required for reproduction or display. Autonomic nerve fiber Varicosities Mitochondrion Synaptic vesicle Single-unit smooth muscle Types of Smooth Muscle Copyright © The McGraw-Hill Companies, Inc. Permission required for reproduction or display. Figure 11.22 Mucosa: Epithelium Lamina propria Muscularis mucosae Muscularis externa: Circular layer Longitudinal layer 11-83 Excitation of Smooth Muscle Smooth muscle is involuntary and can contract without nervous stimulation ◦ Can contract in response to chemical stimuli ◦ Hormones, carbon dioxide, low pH, and oxygen deficiency ◦ In response to stretch ◦ Single-unit smooth muscle in stomach and intestines has pacemaker cells that set off waves of contraction throughout the entire layer of muscle Most smooth muscle is innervated by autonomic nerve fibers ◦ Can trigger and modify contractions Excitation of ◦ Stimulate smooth muscle with either acetylcholine or norepinephrine Smooth ◦ Can have contrasting effects Muscle ◦ Relax the smooth muscle of arteries ◦ Contract smooth muscles of the bronchioles Contraction is triggered by Ca2+, energized by ATP, and achieved by sliding thin past thick filaments Contraction and Relaxation Contraction begins in response to Ca2+ that enters the cell from ECF, a little internally from sarcoplasmic reticulum Voltage, ligand, Ca2+ channels and mechanically open to allow Ca2+ gated (stretching) to enter cell Contraction and Relaxation Calcium binds to calmodulin on thick filaments ◦ Activates myosin light-chain kinase; adds phosphate to regulatory protein on myosin head ◦ Myosin ATPase, hydrolyzing ATP ◦ Enables myosin similar power and recovery strokes like skeletal muscle Cont. Thick filaments pull on thin ones, thin ones pull on dense bodies Contraction and membrane plaques and Relaxation Force is transferred to plasma membrane and entire cell shortens Puckers and twists like someone wringing out a wet towel Contraction and Relaxation Contraction and relaxation very slow in comparison to skeletal muscle ◦ Latent period in skeletal 2 ms, smooth muscle 50 to 100 ms ◦ Tension peaks at about 500 ms (0.5 sec) ◦ Declines over a period of 1 to 2 seconds ◦ Slows myosin ATPase enzyme and pumps that remove Ca2+ ◦ Ca2+ binds to calmodulin instead of troponin ◦ Activates kinases and ATPases that hydrolyze ATP Contraction and Relaxation Latch-bridge mechanism is resistant to fatigue ◦ Heads of myosin molecules do not detach from actin immediately ◦ Do not consume any more ATP ◦ Maintains tetanus tonic contraction (smooth muscle tone) ◦ Arteries—vasomotor tone; intestinal tone ◦ Makes most of its ATP aerobically Smooth Muscle Contraction Copyright © The McGraw-Hill Companies, Inc. Permission required for reproduction or display. Plaque Intermediate filaments of cytoskeleton Actin filaments Dense body Myosin (b) Contracted smooth muscle cells (a) Relaxed smooth muscle cells If the myosin molecules are the “golf clubs” then what are the golf balls? Botulinum (the poison in botulism—a deadly form of food poisoning) prevents the release of acetylcholine. What might be the immediate cause of death in a person who has been poisoned in this way? Critical If you made an intramuscular injection of a chemical which Thinking combined irreversibly with calcium ions, what action would the skeletal muscle be able to perform? What reason do you Questions give for your answer? In what parts of the cell and when must ATP be consumed in order for muscle contraction to occur? If you knew that chickens and domesticated turkeys primarily used their breast muscles (i.e., flight muscles of the pectoral region) to escape from danger, how could this explain the light color of breast meat? Clinical Application Question Henry was in the hospital, having had a mild heart attack. He is feeling very well now and is about to be released. He turns to you and says, since he feels as good as new, that he will be returning to his high-stress, physically demanding job as a professional football line- backer. What do you tell him and why?