Microelectronics: Devices to Circuits Lecture 9 PDF
Document Details
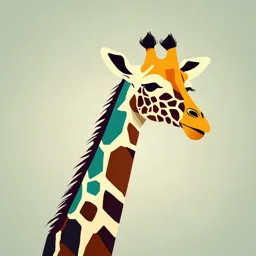
Uploaded by PeerlessBugle
Indian Institute of Technology Roorkee
Sudeb Dasgupta
Tags
Summary
This document is a lecture on Microelectronics: Devices to Circuits, covering BJT small signal circuit models. It discusses small signal models, common emitter configuration, and common base configuration, along with voltage amplification and input/output impedances. Topics are suitable for an undergraduate-level electronics engineering course.
Full Transcript
Microelectronics: Devices to Circuits Professor Sudeb Dasgupta Department of Electronics and Communication Indian Institute of Technology Roorkee Lecture−09...
Microelectronics: Devices to Circuits Professor Sudeb Dasgupta Department of Electronics and Communication Indian Institute of Technology Roorkee Lecture−09 BJT: Small Signal Circuit Model-II Hello and welcome to NPTEL Online Certification course on Microelectronics: Devices to Circuits. In our previous lecture, we had discussed with you the π model and the T model. We have also discussed with you the condition for small signal model and we have seen that the requirement for small signal model has been that, if your input signal is small enough so that your bias point does not change too much and you are in the linear region of operation of the device where amplification is almost independent of the input voltage, we define that to be as smallest signal. We have also understood that if I have a BJT in a common emitter configuration then the amplification is typically very large, of the order of few hundreds in Voltage Amplification. Whereas common collector does not give me voltage amplification directly, right? We also understood about the relationship between α and β, where β is basically the current gain and α is also a current gain in common emitter configuration and β is common current gain in common emitter configuration and α is the current gain in common base configuration. We have seen the functionality and the operation of BJT. What we will do today is, have a look at small signal circuit model we will extend that to CE, CB and CC which means common emitter, common base and common collector. (Refer Slide Time: 2:07) So, just to continue with yesterday’s talk, we will come to this point that if you have a common emitter configuration which you see then, as I discussed with you in the yesterday’s discussion or slide that, you do have a BJT here which is an NPN transistor looking at the direction of the arrow, this is basically an NPN right? So I have a p-type base, an n-type emitter and we have got a collector which is n-type, there is also a resistance ‘Rc’ which primarily is a resistance which is seen at the collector side right? And you have an input resistance also referred to as ‘Rsig’ here and we define ‘Rin’ as the input resistance or input impedance and ‘R0’ as the output resistance or impedance and ‘V0’as the output voltage whereas ‘Vsig’ is my input voltage, input voltage. Now you see that the two aspects which should be very clear at this stage, Vsig is not equal to ‘Vi’ right, so your input voltage to the BJT will be slightly less as compared to the actual signal voltage and the reason being the presence of this Rsignal right? Because these are all voltage sources right, and these voltage sources will have a finite output impedance and as a result, this output impedance will, there will be some drop across that output impedance and therefore the actual potential applied to the base emitter junction of a BJT will be relatively smaller, smaller than what is expected from here, right? Now so we have therefore if you so this BJT is broken up into equivalent small signal model as you can see the blue box here, where you have rπ, rπ is basically nothing but the value of Rin and the reason being rπ is nothing but the resistance between the base emitter junction, right so when the base emitter junction is forward biased this rπ will be of the order of few ohms right to the order of ohms right, because it is a forward bias device characteristics. (Refer Slide Time: 4:08) Now so….so…. the voltage across this rπ is the voltage which is basically Vbe happens to be rπ so Vπ is the voltage across across rπ right? So this is sort of an input voltage which is visible to us and that when multiplied by transconductance (gm) gives me the output current, right? And that is the count, so gm times rπ or Vπ is my output current, that if you multiply with the effective resistance seen from the output sign. What is the effective resistance? RC parallel to R0, right? RC parallel to R0 and therefore I get V0 equals to minus gm times Vi into RC parallel to R0. (Refer Slide Time: 5:05) So if you look very carefully or understand it, then it is basically minus gm times Vπ, right? RC parallel to R0, this is the value of your V0, right? See if you if you break it down Vπ this will be RC R0 upon RC plus R0, right? This will be your so, basically the 1 upon this so minus gm Vπ into R0, R0, fine? So you see therefore if you break it up, I get 1 by R0 plus 1 by RC, right? This is equals to your V0 for our practical purposes. (Refer Slide Time: 6:28) Now you see where R0 at RC is the external potential, RC is the external bias which you have given and R0 is basically the, the output impedance of the BJT, right? So the parallel combination of R0 and RC gives you the net output impedance seen by the device and therefore I have written here that R0 is equal to RC parallel R0, right? And that gives you a fair idea about the type of voltages which you will get. Since gm is relatively high right, so gm is high. Even if this quantity is low quantity right, even if it is low I will get V0 which quiet high, right? (Refer Slide Time: 7:12) So therefore, we can safely assume that that the gain is directly proportional to gm which is an understood phenomena, that it is actually depending on the value of gm, so higher the sensitivity is for a BJT to work, more will be the gain and therefore more will be the output voltage with the same amount of input voltage but please keep in mind that the Vsig which you are using the signal input signal voltage is basically a small signal, right? Please keep this in mind for all practical purposes. This is basically small signal right and therefore these are all small signal models. (Refer Slide Time: 7:43) Now if your signal is large then you entail a non-linearity into your gm, so assuming gm to be constant will be an over assumption, right? So one important point when you do a non-linear analysis or a non-linear profiling which is which we are not doing here, non-linear. Then gm will be actually a function of Vin, in this case Vπ, right? But in reality or at least for the all understanding purposes basic purposes, we assume that gm is sort of independent of independent of Vin, right? In reality gm might be also a function of Vπ and therefore you will entail a non- linearity into the whole system. So…so… therefore we have understood that you do have a problem of nonlinearity also coming into picture, but if we sustain a small value of input voltage there will be no nonlinearity and I would expect to see a gain which is totally independent of Vin and linear gain will be available. (Refer Slide Time: 8:26) Two things we therefore, we take out from this slide is that my input impedance is Rπ and output impedance is basically RC parallel to R0 right? Now so your input impedance is basically Rπ which is nothing but the forward biased EBJ right, Emitter Based Junction. The resistance offered by that is basically my EBJ, right? Second thing is, there is also assumption that this is behaving like a constant current source right, constant current source and we are also assuming it to be ideal. So the voltage, so the impedance offered by this is infinity. But since this is parallel of course therefore this does not play into the picture. I think it is clear to you right? So, what we have therefore assumed is that the current source is basically an ideal current source as a result, the output impedance is infinity and, Since it is a constant current source and the output impedance is infinity, when it is parallel to R0 and RC right, so that vanishes off because it is quite high a quantity, right. So in parallel combination that does not stay to a large extent right, obviously because in parallel you remember it is less than the least in the series and it is larger than the largest. In this case it is less than the least and therefore we just neglect that particular case, right? Okay. (Refer Slide Time: 9:57) We now move into the next slide and see what happens with emitter resistance. Let me see why do you at all needed emitter resistance, very important issue is there when we are having an emitter. Till now if you remember we were actually looking from the point of view or the fact that we had a BJT right? And there was a resistance here, fine and you had a bias here and this bias was varying and you did it like this, like this and then we have a plus VCC and then we are doing like this sorry, we are doing like this, this is RE. Now what we are trying to tell you is, that no let me do small, a change here and the change is that we try to do some change in emitter configuration and what is the change, change is that we try to put a resistance here, why we will see that later on. So let me put a resistance RE here right, emitter resistance, all right. Now see, the reason we want to do it is to ensure what is known as stability, ensure stability of the circuit and I will explain that to you how I get it. See if you remember from your basic class tenth even undergraduate days that or you can understand that whenever you have a negative feedback right? Whenever you have a negative feedback, you automatically have a more stable system, right. The (pay) the price you pay for it is here, gain starts to fall down, right? So if I do a negative feedback in voltage domain which means that the output feedback, the output voltage is fed into the input voltage, input side with a 180 degree phase shifted value then, I can safely assume that my overall gain will reduce because obviously there will be principle of superposition can be applied and the two voltages will be added algebraically as a result the overall voltage bit falls down but then, we also ensure that by doing a negative feedback that the overall gain is more stabilized which means that the gain with respect to change in the input is much smaller. (Refer Slide Time: 12:13) So, negative feedbacks actually gives you a stable system that is what we have learnt and we know all of us possibly know this basic concept. Now if this RE is in series to this external emitter resistance, emitter resistance is applied to, to the side of emitter then when a current IE flows through it then there is a voltage drop which is IE times RE in which this is positive and this is negative, right? So the voltage across this which is let us suppose we assume it to be as VE appears because of IE, RE. This V will start to reverse bias my this base emitter junction right, as it starts to reverse bias it is because you are applying positive side to n-type and maybe a negative side to p-type right, or even a positive bias to the n-side and as a result what is happening is that the emitter is getting more and more positive which means it is trying to reverse bias the emitter base junction and therefore majority current carrier is reducing and you have a large amount of minority carrier which appears. Therefore, when you apply RE your gain starts to fall down, right? And it starts to go down and down. So when you put an emitter resistance RE in series to the BJT we end up having a reduced gain right, a reduced gain but a more stabilized, stabilized circuit. So I have a reduced gain but more stabilized is with me. So that is the reason why we do, we bias with emitter resistance. (Refer Slide Time: 13:49) Now if you look very carefully therefore, in this case we have an RE here which is basically this one and therefore Rin is given as β plus 1, RE plus re , plus RE right, where capital RE is basically the resistance, external resistance and re is the internal resistance. So this so if you go from this point to this point, it is basically RE plus RE which you see in front of you right, that you multiplied with β plus 1 you get total Rin value right, because that is the basic definition of a β now. β will be very much larger than 1 and therefore I can safely write down Rin to be equals to β times re plus RE which appears as β re plus β times RE, right? So if your β is typically very high let us say, it is about 150-200 then the resistances offered by the BJT right and the circuitry gets amplified drastically, right? And the input (temp) and the input resistance becomes very-very large because you can see here, you are multiplying β to both re, small re and capital re and as a result you will have a large amount of voltage across this terminal right, not only that you will also have to reduce the gain of the MOS device which you are using, other FET device of the MOSFET, sorry BJT which you are using. (Refer Slide Time: 15:27) Now therefore I get Av0 to be equals to minus gm times RC divided by 1 plus gm RE, right? So it is basically gain is equals to minus gm RC divided by 1 plus gm times R0, fine. And this your voltage gain Av0. We also assume for all practical purposes that RE is so large as compared to RC that RE is RC is approximately equal to RE and as a result R0 equals to RC, right? So the output impedance or output resistance seen by the device is approximately equal to the collector resistance offered by the BJT, right. These two things we will be assuming for all practical purposes. If this is true we can look at the left hand side figure, the down figure here, then we see that I have got Vsig, I have got Rsig which is the signal specifications which terminates at this particular point and you have α times IE as the collector current source right, assuming that the emitter current is fully biased and only depends upon the input bias and not on anything else. I can safely say that the collector current here is equals to α times IE, right? The IE also flows to the below side so IE times RE is basically the voltage drop which you see on the below side of the channel, right. And that is what you get from all of these regions, right. Okay. We now come to the common base amplifier and the common base amplifier you will see that my base is grounded right, so the previous was basically your base emitter grounded, now we are entered into base grounded. So when the base is grounded I get my, I am applying signal to the input side on the emitter side and let us see how it works out. The problem with this is technically is when you apply a signal on the emitter side right, when you apply a signal on the emitter side, you end up having a large parasitic capacitances being extracted even at relatively small voltages, right and as a result what will happen is that the speed of the operation will get reduced, right. So one has to be very cautious when we design a common base amplifier in a small confined domain. Now with this knowledge we can safely right down or we can actually give an instruction that Rin, is equal to RE, no doubt because of the previous discussion we can just remove it. (Refer Slide Time: 18:00) So if you see Rin is equal to RE, which is given here, so I get Rin equals to RE. That is what you get Rin equals to re why, because input resistance right, input resistance which you feed here is exactly equals to RE. RE is the output impedance of a BJT at this stage. So I get Av0 equals to gm times RC and R0 equals to RC, right? So you can understand that, your R0 is typically very large quantity and therefore gm times RC is very large and that is the reason you do have a large amplification in their case, in their this thing that is compared to other experiments or other this thing because the same reason is that your gm is relatively independent of the applied currents, right. (Refer Slide Time: 19:01) Common Collector Amplifier as the name suggests is also referred to as Common emitter or emitter follower amplifier and is given by this formula. In this case please understand the voltage gain is approximately equals to zero and that is what we all understand that common collector is not a very good amplifier or it cannot amplify small signals to large values, right and that is quite important that they cannot do it and since they cannot do it, they are used for some other purposes but not for amplification purposes, right. And that is what we come to know right, because Av0 equals to 1 so, it is basically phase change which is looking into and nothing else and is given by α times IE which is basically the actual current source which is available to us. RL is already there, Rsignal and Vsignal is also available. Output impedance of current and resistances are also available with me and gives me quite interesting results as far as common collector or a common this is given. (Refer Slide Time: 19:55) So let me recapitulate what we did. Common emitter configuration is the most easiest and the most high gain, can be obtained from common emitter configuration. The common base amplifier user high frequency amplified it with low input signal, right? Input signal is quite low and therefore you use a high amplifier design. The emitter follower finds application as a voltage buffer right, for connecting high source and passes it onto right source. So if you do a common collector, you are actually, though you amplification will be low voltage to voltage but your transits will be very-very fast which means that if you want to compare the output impedance of a particular system with your system. On the collector side if you put the resistance is small and therefore you can easily match the low input impedance and their design. So the emitter follower finds application as voltage buffer for connecting a higher source to a load resistance source, right? So emitter follower generally used, typically used for the purpose of buffering or the purpose of connecting between two points through a network right and that is what is emitter follower is all about. It is basically we require it the power it to be low and it is excessively low right and that that is what is very important. With this we have almost finished this section but before we move forward let me give you a brief questionnaire or a question maybe, right? (Refer Slide Time: 21:27) So I have a question, so I have a transistor whose beta is equals to 100 right and applied voltage is 10 volts so I get IC, so I need to find out first of all beta, this is 3 volts, so we need to find out IB first. So to do that in this loop, let me find out that this is 0.7, right? And you are given 3 volts, so what you get from here is that IB will be equals to VBB minus Vs right? This just drop divided by r which is 100 k and if you solve it comes out to 0.023 mA, right? (Refer Slide Time: 23:12) So the amount of current which flows is typically very small. So therefore IC will be equals to 100 times 0.023 mAs so IC will be there for approximately 23 mAs, fine. So typically this much amount of current will be required for all practical purposes. Now, if you again go back and explain the relative slides here we can (show), we can safely show that VC is equals to VCC minus ICRC, right? In this case we find out this to be as plus 3.1 volt. Now if you find out the value of RE it is VT by IE. VT is 25 mV/decade divided by VT, VT is divided by IE actually. IE will be VT divided by 2.3 divided by 0.99 and this comes out to be approximately close to 10.8 Ω, which means that 10.8 Ω is the is the emitter resistance offered by the emitter to the input system , right? Transconductance (gm) can be written as IC by VT and therefore this can be written as 92 mA per voltage minus, right? Rπ β by gm, we get 100 by 92, fine. So this is how we do a small signal analysis as far as this course is concerned and gives you an idea about how a small signal analysis works even for a single transistor or for a BJT. A transistor wherein BJT starts to find out new methodologies to destabilize itself, right? So therefore these are the few areas in which people are working and quite interesting work is going on. Now, so, what we did was, we used a common emitter configuration for the best configuration and we and then what we saw was that for if your gain is typically very large, bulk gain is very-very large and your actual gain or the device gain is relatively small we can still have that as the best suited amplifier. The common base is used for high frequency due to low input resistance is used for high frequency amplifier, right? The emitter follower finds applications of voltage buffer for connecting a high source to a low resistance path, right. And this we have done for emitter follower, we have done and we do for common base as well, right? (Refer Slide Time: 25:42) We will therefore in this stage, recapitulate what we did, we did collector common collector emitter base configuration, we also looked into the fact that given a result or given this thing which one is the best form of configuration and for that common emitter is the best form of configuration as far as the results are confirmed. Thank you very much for your patient hearing. We will come back to you next time with more information.