L7 Respiratory Mechanics Gas Transport Resp Control Blackwell FHP 2024 PDF
Document Details
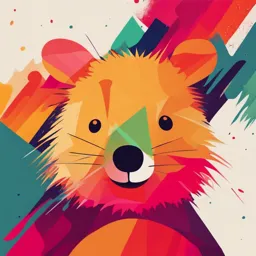
Uploaded by UnquestionableKremlin
Rutgers University
2024
Krista N. Blackwell, Ph.D.
Tags
Summary
These lecture notes cover pulmonary mechanics, gas transport, and respiratory control. The document includes information on factors influencing gas diffusion, solubility of gases, regional differences in perfusion and ventilation, ventilation-perfusion relationships, pulmonary gas exchange, and oxygen/carbon dioxide transport. It also covers the control of respiration, including chemoreceptors and mechanoreceptors.
Full Transcript
Pulmonary Mechanics-Pt 2, Gas Exchange and Transport, Control of Breathing Krista N. Blackwell, Ph.D. Email: [email protected] At the end of this lecture you should be able to: List four factors that influence the diffusion of gases across the alveoli. Explain the difference between the conc...
Pulmonary Mechanics-Pt 2, Gas Exchange and Transport, Control of Breathing Krista N. Blackwell, Ph.D. Email: [email protected] At the end of this lecture you should be able to: List four factors that influence the diffusion of gases across the alveoli. Explain the difference between the concentration of a gas in solution and the partial pressure of that gas in solution and identify the factors that influence the movement of gas into solution. Compare and contrast the solubility of oxygen and carbon dioxide. Describe normal physiological pressures of oxygen and carbon dioxide in the following locations: alveoli, arterial blood, resting cells, and venous blood. Describe the regional differences in perfusion (blood flow) and the differences in driving pressure and alveolar size in zones 1-3 of the lung Describe the regional differences in ventilation and the variations in the intrapleural pressure, transpulmonary pressure and alveolar size vary at the apex and base of the lung Be able to identify the ventilation-perfusion relationship as it relates to dead space and shunt (as described by changes in PO2 and PCO2 levels) Identify the importance of Henry’s Law and Fick’s Law of Diffusion on gas exchange Identify the factors that increase and decrease the pulmonary diffusing capacity Describe oxygen transport in the body, identifying – The amount of oxygen physically dissolved in the blood – The amount oxygen chemically bound in the blood At the end of this lecture you should be able to: Be able to calculate the following: – % Oxygen saturation – Oxygen content Identify the oxyhemoglobin dissociation curve and factors that can alter oxygen-hemoglobin binding Identify the carbon dioxide dissociation curve and how oxygen levels can affect this curve Draw the oxyhemoglobin dissociation curve (for normal conditions) and explain the physiological significance of the shape of this curve. Draw the shifts in the oxygen-hemoglobin dissociation curve that result from changes in pH, temperature, and levels 2, 3-DPG. Diagram the factors affecting total oxygen content of arterial blood. Start with the equation: total arterial oxygen content = amount dissolved in plasma + amount bound to hemoglobin. Identify the three ways in which carbon dioxide is transported in the blood Characterize the roles of the brain regions involved in the neural control of breathing. Identify the specific mechanisms by which central and peripheral chemoreceptors monitor carbon dioxide, oxygen, and pH levels for the purpose of regulating ventilation. Pulmonary Mechanics – Part 2: Airway Resistance Factors that influence alveolar gas exchange The resistance to airflow results from the resistance in the pulmonary tissue and the airway resistance The pulmonary tissue resistance accounts for ~20% of the total resistance, while airway resistance accounts for ~80% of the total resistance Pouiseille’s law describes the effects of radius on airway resistance in the lungs 90% of the airway resistance comes from the upper airways The trachea and bronchi are small rigid structures with a low cross-sectional area Their support by cartilage and bone result in little change in the size of these airways Mucus (with allergies or infection) can increase the resistance in these airways Bronchioles are smaller airways branching off the bronchi that have no cartilage support Airway Resistance and Bronchiolar Diameter Bronchioles are collapsible tubes that usually do not contribute to airway resistance (Large CSA) When there is a decrease in the diameter of the bronchioles, they can become a major source of airway resistance The bronchioles are under nervous system (ANS) and hormonal control (paracrine) – Bronchoconstriction and bronchodilation can occur in the presence of various stimuli and alter the airway resistance Asthma results from a narrowing of the airways Asthma – is an acute form of obstructive lung disease and an inflammatory disorder – can be mild or life-threatening – once a trigger is inhaled ( allergens, heat, cold, irritants and exercise) it leads to the activation of the inflammatory response and the development of) and bronchoconstriction – bronchospasm, secondary to the inflammatory response, causes bronchiolar constriction; the contraction of smooth muscle in the bronchiolar walls results from activation of a variety of chemical signals (acetylcholine, histamine, substance P (neuropeptide) and leukotrienes) – causes shortness of breath, wheezing, chest tightness and coughing – results in a decreased FEV1/FVC ratio – can be treated with inhaled and oral medications (β2-adrenergic agonists, anti-inflammatory agents and leukotriene inhibitors) Dynamic airway compression occurs in the bronchioles when lung volumes are low Dynamic airway compression of the airways can occur at low lung volumes, when the airway resistance is very high The low lung volumes that result with a forced expiration can cause dynamic airway compression – With forced expiration, the contraction of the expiratory muscles causes the intrapleural pressures to rise a higher value than the pressure in the airways – As the lung volumes decrease, the transpulmonary pressure decreases – In the regions of the airways that are above the point at which the transpulmonary pressure is 0, the transpulmonary pressure becomes negative which causes the airway collapse Dynamic airway compression occurs when the transpulmonary pressure becomes negative Transpulmonary pressure = Pip- Ppl For a normal expiration, the transpulmonary pressure is positive, when calculating the difference between the intra-alveolar (Pip, intrapulmonary pressure) and the intrapleural pressure Ppl = intrapleural pressure intrapulmonary pressure Ppl Transpulmonary pressure = Pip- Ppl =+1-(-8) = +9 (bronchioles) or = Pip- Ppl =+0.5-(-8) = +8.5 (bronchioles) Ppl Pip Transpulmonary pressure = Pip- Ppl =+2-(-8) = +10 (alveolus) In the bronchioles (area in green), the intrapulmonary pressure is lower than in the alveolus, but the transpulmonary pressure is still positive Pip= Pip Pip Dynamic airway compression occurs when the transpulmonary pressure becomes negative With a forced expiration, transpulmonary pressure becomes negative when the intrapleural pressure becomes positive at low lung volumes Ppl = intrapleural pressure Pip= intrapulmonary pressure Ppl Ppl Transpulmonary pressure = Pip- Ppl =+35-(+25) = +10 (alveolus) In the bronchioles (area in green), the intrapulmonary pressure gets smaller, but the intrapleural pressure is positive when you reach very low lung volumes Transpulmonary pressure (at various points in the bronchioles) = Pip- Ppl =+30-(+25) = +5 (bronchioles) = Pip- Ppl =++25-(+25) = 0(bronchioles) = Pip- Ppl =+20-(+25) = -5(bronchioles) The point at which the transpulmonary pressure is less than zero (in the bronchioles) and/ or then becomes negative is where the airway will collapse (dynamic airway compression) Pip Pip Pip Ventilation-Perfusion Relationship A close relationship between the alveoli and the pulmonary blood is necessary for proper gas exchange Exchange I: Atmosphere to Lung Ventilation and Alveolar Ventilation Exchange II: Lung to Blood Pulmonary Gas Exchange and Oxygen and carbon dioxide transport in the blood Exchange III: Blood to Cells Cellular Respiration (via mitochondria) The ventilation-perfusion relationship is the ratio of the of alveolar ventilation and blood perfusion rates of the lung Ideally, each alveolus in the lungs would receive the same amount of ventilation and pulmonary capillary blood flow (perfusion). In reality, ventilation and perfusion differ depending on the region of the lung The balance between alveolar ventilation and perfusion ultimately determine the partial pressures of oxygen and carbon dioxide within the alveolus and in turn the arterial blood http://www.pathwaymedicine.org/alveolar-air-composition The Ventilation Perfusion-Relationship Regional Variations in the Blood Flow in the Lung Blood flow distribution of the lungs is determined by the relationship between pulmonary capillary pressures (at the arterial and venous ends) and the alveolar air pressure In the normal upright lung, blood flow (per unit volume) decreases from the bottom of the lung to the top of the lung because of the differences in the hydrostatic pressure (due to gravity) on the pulmonary vessels Zone 1: No blood flow (higher alveolar air pressures) Zone 2: Intermittent blood flow occurs (only with peak systolic pressures ~25mm Hg) Zone 3: Blood flow occurs continuously Regional Variations in the Blood Flow in the Lung The lung can be divided into three zones, based on alveolar (PA), arterial (Pa), and venous (Pv) pressures – Zone 1 is the upper zone and represents alveolar dead space because alveolar air pressure continually occludes the pulmonary capillaries. – In the middle zone (zone 2), pulmonary capillary flow is intermittent and varies during respiration according to the arterial–alveolar pressure gradient. – Pulmonary capillary flow is continuous in zone 3 and is proportional to the arterial– venous pressure gradient. Zone 1: No blood flow (higher alveolar air pressures) Zone 2: Intermittent blood flow occurs (only with peak systolic pressures ~25mm Hg) Zone 3: Blood flow occurs continuously Intrapleural pressures are more negative at the apex of the lung and less negative at the base The regional differences in lung ventilation are due to the variations in pleural pressures (and transpulmonary pressures) that exist between the apex and base of the lungs Alveoli in upper lung have a higher transpulmonary pressure, are nearmaximally inflated and relatively noncompliant – These alveoli expand less during inspiration Alveoli in lower lung are smaller and have a lower transpulmonary pressure – These alveoli are more compliant and undergo greater expansion during inspiration Summary of Ventilation and Perfusion Regional Variations Ventilation-Perfusion Relationships of the Lung Although blood flow and ventilation are both greater at the base of the lung, there is a greater variation in the perfusion between the upper and the lower lung V/Q normally ranges between 0.3 and 3.0; most of the lung has a V/Q ratio of 0.8 - 1.2 O2-CO2 diagram and the Ventilation-Perfusion Relationship of the Lungs This diagram illustrates changes in.. the V/Q ratio with changes in alveolar PO2 and alveolar PCO2 If the V/Q relationship = 0, the alveoli are not ventilated, only perfused (Shunt) – Alveolar PO2 = Venous PO2.. When the V/Q ratio = 3, the alveoli are ventilated, not perfused (alveolar dead space) – Alveolar PO2 = Inspired PO2 Hypoxic pulmonary vasoconstriction redistributes blood flow to maintain a matched V/Q in the lungs Local factors (i.e. hypoxia) are extremely important in influencing pulmonary vascular tone Alveolar hypoxia (low alveolar PO2), alveolar hypercapnia (increased carbon dioxide) and atelectasis (collapsed alveoli) can cause pulmonary vasoconstriction in the pulmonary circulation Hypoxic pulmonary vasoconstriction is a physiological mechanism that reduces intrapulmonary shunting and prevents hypoxemia (low blood O2 levels) – It is not completely understood, but it is a local response to specific areas of alveolar hypoxia Hypoxic pulmonary vasoconstriction (HPV) occurs via the following steps A. Perfusion of a hypoventilated alveolus causes a decreased PO2 and increased PCO2 for the blood entering the pulmonary vein B. HPV causes an increased resistance to blood flow to the poorly ventilated alveolus C. Blood is diverted from the poorly ventilated alveolus to better ventilated alveoli Hypoxic pulmonary vasoconstriction (a-c) redistributes blood flow to maintain a matched V/Q in the lungs Pulmonary gas exchange and transport of oxygen and carbon dioxide Exchange I: Atmosphere to Lung Ventilation and Alveolar Ventilation Exchange II: Lung to Blood Pulmonary Gas Exchange and Oxygen and carbon dioxide transport in the blood Exchange III: Blood to Cells Cellular Respiration (via mitochondria) Exchange IIa: Pulmonary Gas Exchange Gas exchange in the respiratory units occur at the alveolar-capillary membrane, which forms the blood gas-interface Pulmonary Gas Exchange Henry’s Law: states that the quantity of a slightly soluble gas that dissolves in a given mass of liquid at a particular temperature is proportional to the partial pressure of that gas Fick’s First Law of Diffusion: states that the diffusion rate increases when the surface area, the ionic concentration gradient and the permeability increases Pulmonary Diffusing capacity: measures the ability of the alveolar-capillary membranes to conduct gases CO2 diffusing capacity ~400-450 ml/min/mm Hg( 20X greater than the O2 diffusing capacity) O2 diffusing capacity ~21 ml/min/mm Hg CO diffusing capacity ~17 ml/min/mm Hg (O2 diffusing capacity ÷ 1.23) Pathological changes that can affect pulmonary gas exchange Pathological changes that can adversely affect gas exchange – – – – Reductions in the surface area Increased thickness of the alveolar capillary membrane Increased diffusion distance (between the alveolus and the blood) A decreased airflow into the lungs Exchange IIb: Oxygen and carbon dioxide transport in the blood Oxygen Transport in the Body O2 in the blood is transported in two ways; what is physically dissolved in the blood and what is chemically bound to hemoglobin (Hb) The physically dissolved O2 is very low, O2 is relatively insoluble in the blood – At a normal body temperature, the solubility coefficient for oxygen is 0.003mL/100 mL of blood – A PO2 of 100 mm Hg: dissolved O2 = 0.003 100 mm Hg = 0.3 mL/100 mL blood ∙ Oxygen Transport in the Body Hemoglobin (Hb) is a complex molecule with a tetrameric structure consisting of 4 polypeptide chains (globin), each of which contains a protoporphyrin (heme) group – The heme group has a ferrous atom in the center and can bind one molecule of oxygen – Each Hb molecule can bind 4 molecules of O2 Normal adult Hb (HbA) consists of two α-chains and two β-chains More than 120 abnormal variants have been demonstrated in patients – HbS: causes sickle cell disease is well known – HbS polymerizes and crystallizes in the cytosol of the red blood cell(erythrocyte) when it is not combined with oxygen and its shape changes to a crescent or ”sickle” shape – Sickled shaped cells have a tendency to stick together, increasing the blood viscosity and also increases the risk for blood vessel blockage (thrombus formation) Blood O2 content is the physically dissolved O2 and the blood O2 capacity Blood O2 content is the amount of oxygen in vol % (mL O2 per 100 mL blood) present in the blood at any partial pressure of O2 Blood O2 content = Physically dissolved O2 + Blood O2 capacity Blood O2 carrying capacity is the amount of O2 that will combine with Hb in the blood at a given PO2 The oxygen-carrying capacity: – 15g Hb/100 mL blood X 1.34 mL O2/ g Hb = 20.1 mL O2/100 mL of blood Sample Calculation: Calculate the oxygen content at a PO2 of 40 mmHg. A. Blood O2 capacity: the oxygen bound to Hb at a PO2 of 40 mmHg (~around 75% saturated): 20.1 mL O2/100 mL of blood X 75% = 15.08 mL O2/100 mL of blood – B. – Physically dissolved O2 at a PO2 of 40 mmHg: 0.003 mL/100mL blood PO2 (in mmHg ) X 40 mm Hg = 0.12 mL O2/100 mL of blood C. – Blood O2 content at a PO2 of 40 mmHg: 15.08 mL O2/100 mL of blood + 0.12 mL O2/100 mL of blood = 15.2 mL O2/100 mL of blood ∙ Oxygen Loading (Content) in the Lungs Oxygen Transport in the Body The oxyhemoglobin dissociation curve or the oxygen dissociation curve relates the percent of O2 saturation to the partial pressure of oxygen (PO2) – – – The curve has a sigmoidal shape (it plots all 4 reactions of oxygen binding to the Hb molecule) The reactions the Hb subunits (binding O2) do not occurring simultaneously, they occur sequentially, with each reaction assists the next reaction As each heme group is oxygenated the affinity for oxygen for the remaining heme groups increases The dissociation for oxygen from the heme groups occurs in the same manner – The dissociation or loss of oxygen from one heme group speeds up the successive reactions At a normal arterial P02 of 100mM Hg, the Hb saturation is 98% Oxygen Transport in the Body Percent saturation = content of oxygen in the blood * 100% oxygen carrying capacity of Hb 1 gram of Hb when saturated binds 1.34 mL O2 and the normal Hb concentration is 15g/ 100 mL of blood Therefore the maximum amount of O2 that can be carried in the blood is – 15g Hb/100 mL blood X 1.34 mL O2/ g Hb = 20.1 mL O2/100 mL of blood Shifts in the oxyhemoglobin dissociation curve can be best described by the P50 – The P50 is the PO2 at which 50% of the Hb present in the blood is deoxyhemoglobin and the other 50% is oxyhemoglobin Alterations in the Oxyhemoglobin Dissociation Curve Alterations in temperature, pH, PCO and 2, 3-DPG can cause shifts in the oxyhemoglobin dissociation curves 2 – When the curve shifts to the right, there is a greater ability to release oxygen and when it shifts to the left oxygen is less likely to be released – Bohr Effect: explains why cells oxygen release or red blood cells unload oxygen in tissues Carbon dioxide (CO2) is the key player A normal process in healthy people More oxygen is released in tissues that have higher CO2 values Shifts in the oxyhemoglobin dissociation curve can be best described by the P50 – The P50 is the PO2 at which 50% of the Hb present in the blood is deoxyhemoglobin and the other 50% is oxyhemoglobin Other factors than can affect the oxyhemoglobin dissociation curve include anemia, nitric oxide levels, carbon monoxide levels , the presence of other forms of Hb (Methemoglobin (Mb) and Fetal hemoglobin (Hb F) Factors which affect the Hemoglobin Dissociation Curve Carbon Dioxide Transport in the Body Carbon dioxide is transported (3 ways)in the blood: dissolved carbon dioxide (amount carried in the physical solution - 7%), carbamino compounds (carbon dioxide that chemically combines with amine groups of blood proteins (25%) and bicarbonate ions (70%) Respiratory Stress: Hypoxia and Hypoxemia Hypoxia and Hypoxemia are types of Respiratory Stress Hypoxemia: insufficient oxygen in the blood Hypoxia: insufficient oxygen in the tissues When discussing the causes of respiratory stress, the terms hypoxia and hypoxemia are often used interchangeably The causes of hypoxia (hypoxemia) can be classified into four groups: – – – – Hypoxic hypoxia Anemic Hypoxia Hypoperfusion Hypoxia Histotoxic Hypoxia Clinically, the treatment of hypoxia or hypoxemia depends on the type of hypoxia Arterial Blood Gases are used to assess lung function by measuring the levels of oxygen, carbon dioxide and the acidity (pH) of the arterial blood – PaO2, PaCO2, pH, HCO3, O2 content, O2 saturation Causes of Hypoxia/Hypoxemia The causes of hypoxia can be classified into four groups: Control of Respiration Control of Respiration Breathing is involuntary and controlled by specific regions within the brainstem Understanding the control of breathing is complex, due to the anatomical location and the complex organization of neural networks – Some animal experiments and patients with brain damage or identifiable brain lesions have led to the current knowledge for the control of breathing A Model for the control of stimulation states: 1. Respiratory neurons in the medulla control the inspiratory and expiratory muscles 2. Neurons in the pons integrate sensory information which also influences stimulation 3. A network of spontaneously firing neurons (pacemakers) generate the rhythmic pattern of breathing 4. Specific chemoreceptors and mechanoreceptors can also modulate ventilation The control of respiration will be split into the neural control of respiration, chemical control of respiration and mechanoreceptor control of respiration The Brainstem and the Neural Control of Respiration Normal breathing does not occur without neural control (involuntary control) There are 2 main areas in the brain that are responsible for rhythmic respiratory patterns The Medullary Respiratory Center (contains neurons in the Nucleus Tractus Solitarius-Dorsal Respiratory Group and the Ventral Respiratory Group Neurons) The Pons (Pontine Respiratory Group – once called the The Pneumotaxic and Apneustic Centers) Input is received from the mechanoreceptors, sensory input (higher brain centers – hypothalamus/limbic system) and chemoreceptors to modulate the rate of breathing Output from the brain is sent to the muscles of breathing and the muscles that support components of the upper and lower respiratory tract (pharynx, larynx and tongue) The DRG and VRG neurons control inspiration and some of the VRG neurons control expiration The dorsal respiratory group (DRG) contains primary inspiratory neurons and controls the basic rhythm of respiration – – DRG – Stimulates the inspiratory muscles (mainly involved in normal quiet breathing) Also integrates all other input (mechanoreceptors, chemoreceptors and most sensory input) Phrenic Nerve Diaphragm Normal (quiet) expiration is controlled by the shutoff of the inspiratory neurons The ventral respiratory group (VRG) is thought to contain the spontaneously firing cells (pacemakers) for respiratory rhythm (Pre-Botzinger Complex) In addition the VRG stimulates the muscles involved in an active (forced) inspiration and expiration The Pontine Respiratory Groups (Apneustic and Pnuemotaxic centers) “fine-tune” or provide a smooth respiratory rhythm Normal Inspiratory and Expiratory Neural Patterns Central and Peripheral Chemoreceptors are stimulated by changes in PCO2, PO2 and pH Peripheral Chemoreceptors sense changes in arterial PCO2, PaO2 and pH Peripheral Chemoreceptors are located at the carotid and aortic bodies (near the baroreceptors that control BP) and sense changes in PaO2, PCO2 and hydrogen ion concentrations (pH) Hypoxia stimulates ventilation via the stimulation of these chemoreceptors – When PO2 falls below 70(60) mm Hg, ventilation will increase – With COPD and when ascending to high altitude, changes in PaO2 can occur and activate these receptors Glomus cells in the carotid and aortic bodies stimulate action potentials which activate inspiratory signals in the medulla Central Chemoreceptors sense changes in arterial and cerebrospinal fluid (CSF) PCO2 and CSF pH Central chemoreceptors are located on the ventrolateral surface of the medulla – sense changes in PCO2 (arterial and CSF) and H+ concentration (pH) The changes in the CO2 and H+ ion concentrations are sensed in the cerebrospinal fluid (CSF) – PaCO2 is sensed because the CO2 crosses the blood brain barrier (BBB) – CO2 in the brain (in the presence of water) moves into the CSF where it is converted to carbonic acid by carbonic anhydrase which dissociates into H+ and HCO3- Arterial pH is not sensed because and H+ ions cross the BBB very slowly Signaling Pathway for Central and Peripheral Chemoreceptors Pulmonary Mechanoreceptors are activated by noxious stimuli and work to maintain normal ventilation in the lungs Pulmonary Mechanoreceptors and Higher Brain Centers in the Control of Breathing Irritant receptors are located in the airway mucosa of the large diameter airways and are activated in response to noxious gases or irritants – Activation of these mechanoreceptors stimulates bronchoconstriction (via parasympathetic activation of the bronchiolar smooth muscle) – These receptors can also stimulate coughing and/or sneezing in response to these stimuli Pulmonary Stretch Receptors (Hering-Breuer Reflex) are located in the muscle walls of the bronchi and the bronchioles and are activated by distention (stretch) of the airways in response to large tidal volume inspirations – Activation of these receptors act as an “inspiratory off switch”, resulting in an inhibition of respiration – This mechanism protects against excessive filling of the lungs and is more important in infants that in adults Emotional activities (limbic system) and autonomic activities (fight, flight, fear and excitement) controlled by the hypothalamus can increase the rate of breathing – Some of these responses can bypass the medullary neurons but cannot bypass chemoreceptor activation