L6 NEUR3101 Motor Control Lecture 6 - PDF
Document Details
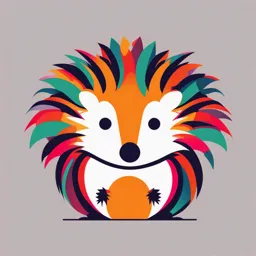
Uploaded by ThoughtfulRetinalite
UNSW Sydney
A/Prof Ingvars Birznieks
Tags
Summary
This document presents a lecture on motor control, focusing on the ascending and descending tracts in the nervous system. It details the characteristics of voluntary movements and the role of the primary motor cortex. The document also discusses pyramidal and extrapyramidal pathways, and includes a summary.
Full Transcript
NEUR3101 Motor control Lecture 6 Brain and movement The ascending and descending tracts A/Prof Ingvars Birznieks Characteristics of voluntary movements Goal-directed Greater flexibility than reflex or rhythmic movements Reflexes and rhythmic movements can be...
NEUR3101 Motor control Lecture 6 Brain and movement The ascending and descending tracts A/Prof Ingvars Birznieks Characteristics of voluntary movements Goal-directed Greater flexibility than reflex or rhythmic movements Reflexes and rhythmic movements can be part of voluntary movements Program Execution Primary motor cortex Supplementary motor area Premotor area Idea plan Octopus: voluntary movements vs complex movements controlled by CPGs https://www.youtube.com/embed/dxQmOR_QLfQ Ika odori don Motor cortex Primary motor cortex and premotor cortex Mediate the planning and initiation of complex temporal sequences of voluntary movements. Cortical areas receive regulatory inputs from basal ganglia and cerebellum via relays in the ventrolateral thalamus. Figure 17.2 Primary motor cortex Theodor Fritsch and Eduard Hitzig demonstrated that electrical stimulation of motor cortex in dogs elicit contraction of muscles on the contralateral side. Based on observations in progression of epileptic seizures John Hughlings Jackson proposed that motor cortex contains an organised spatial representation, or map, of the body’s musculature. Charles Sherrington published maps of the organisation SOMATOSENSORY of motor cortex in great apes. During 1930s Sherrington’s student American neurosurgeon Wilder Penfield demonstrated in great detail that human motor cortex contains a motor map of the body. His data were based on examination of 400 neurosurgical patients. The cortical homunculus is a visual representation of the concept of "the body within the brain“. Homunculus means little man. It was first drawn by an artist hired by Penfield Mrs. H. P. Cantlie. MOTOR Homunculi Primary motor cortex Finer organization assessed by cortical microstimulation and spike triggered EMG averaging revealed that structure of the maps is rather different from what was initially believed. Figure 17.6 Primary motor cortex Even smallest currents capable to illicit response excited several muscles and simultaneously inhibited others suggesting that organized movements rather than individual muscles are represented in the map. This peripheral muscle group is referred to as a muscle field. Note the difference: motor neuron pool refers to all motor neurons innervating a single muscle. Edward Evarts (NIH, USA) found in behaving animals that force generated by contracting muscles correlated with firing rate of upper motor neurons even prior to the execution of movement. Within the same body parts a particular movement could be elicited by stimulation of widely separated sites supporting argument that neurons are connected in networks to create specific movement patterns. Primary motor cortex Michael Graziano from Princeton used microstimulation varying stimulation time so that it would resemble volitional movements (hundreds of milliseconds to several seconds). Resulting movements were sequentially distributed across multiple joints and were strikingly purposeful and target oriented. Output encoded not simply the trajectory of arm motion, but also the final position of the hand: hand to mouth as if to feed (left panel) hand to central space as if to inspect an object (right panel) + starting position final position ▬ movement path Figure 17.7 Primary motor cortex Summary: Activity of upper motor neurons in the cortex controls movements rather than individual muscles. Such organisation represents dynamic and flexible means for encoding higher order movement parameters leading to the coordinated activation of multiple muscle groups across several joints to perform behaviourally useful actions. Primary motor cortex Primary motor cortex layer 5 pyramidal cells are the upper motor neurons. Betz cells are the largest neurons (by soma size) in body, but constitute only 5% of pyramidal cell projections to the spinal cord. Betz pyramidal neurons are primary responsible for skilled movements of the hands and fingers. Corticospinal and corticobulbar (to brainstem) tract axons pass through the internal capsule. 90% of pyramidal tract neurons cross midline (decussate). 10% form anterior corticospinal tract and terminate ipsilaterally or bilaterally. Mostly control axial and proximal limb muscles. Only limited number of axons has privilege to synapse directly with α-motor neurons limited to forearm and hand. Some elements of corticobulbar and corticospinal tracts originate from somatosensory cortex and terminate in dorsal horn which is mostly sensory. They are likely to be involved in modulation of proprioceptive signals. Figure 17.3&4 Pyramidal and extrapyramidal pathways Pyramidal system Corticospinal tract - connects to spinal motor neurons Lateral corticospinal tract – the major corticospinal tract Anterior = ventral = medial corticospinal tract – smaller, gradually diminishes in size as it descends, it ends about the middle of the thoracic region Corticobulbar tract - composed of the upper motor neurons of the cranial nerves Extrapyramidal system Tectospinal tract Rubrospinal tract Reticulospinal tract Vestibulospinal tract Pyramidal tracts Pyramidal tracts Corticobulbar tract connects upper motor neurons in the cortex to lower motor neurons in the motor nuclei of the cranial nerves located in the brainstem. Motor nuclei of the cranial nerves should be viewed functionally analogous to the spinal cord. However, they are located in the brainstem only because they mainly control muscles in the head. Note that lower motor neurons are located only in the nuclei of cranial nerves, other nuclei involved in higher motor control function also located in the brainstem do not have direct access to muscles and thus do not contain any lower motor neurons. The brainstem consists of the midbrain, the pons, and the medulla oblongata. Ventral and lateral corticospinal tracts Very important descending motor system in humans with approx. 1 million fibres Originates in the primary motor cortex, premotor areas, and somatosensory cortex! Consists from Lateral corticospinal tract Controls distal limb muscles Ventral / anterior corticospinal tract Controls axial (neck & back) Fig. 11.2 p 197 muscles Noback et al. (2005) The Human Nervous System. 6th Ed. Humana Press, Totowa, NJ, USA Kandel Schwartz & Jessel (2000). Principles of Neural Science 4/e McGraw-Hill. Lateral corticospinal tract ~ 90% of corticospinal fibres Crosses the midline at the pyramidal decussation. Project to local circuit neurons, and in some cases directly on the alpha and gamma motor neurons of distal muscles of upper and lower extremities - hands and feet. Humans have more monosynaptic direct connections to motor neurons than any other animals. This allows greater flexibility and fine control of digits. Small proportion of CS tract fibres (~2%) descend without decussating as the uncrossed lateral corticospinal tract (i.e. control distal muscles on the ipsilateral side) Kandel Schwartz & Jessel (2000). Principles of Neural Science 4/e McGraw-Hill. Ventral / Anterior corticospinal tract ~ 10% of corticospinal fibres Does not cross the midline at the pyramidal decussation. Fibres project bilaterally to ventro- medial spinal cord (axial muscles – neck, shoulder, trunk), meaning that they cross at the level of the target spinal segment. Extrapyramidal system Pyramidal system Tectospinal tract Pyramidal tract - coordinating head and eye - control most of our fine movements movements as part of the visual and auditory reflexes Rubrospinal tract - fractionation of fine movements activation of flexor muscles Vestibulospinal tract - controls postural muscles Reticulospinal tract - projects from the reticular formation - inhibition or facilitation of movement From : The Central Nervous System, P. Brodal - feedforward control Extrapyramidal pathways Rubrospinal Receives afferent fibres from the motor cortex and cerebellum. Regulates tone: excitatory effect on limb flexor muscles, inhibitory effect on extensor muscles. Neural activity in the red nucleus is related to force, velocity and direction of fine movements. Responsible for fractionation of movement. Fractionation is the ability to apply movement to one joint in isolation without affecting other joints. Reticulospinal Descends from reticular formation in two tracts to act on the motor neurons supplying the trunk and proximal limb muscles. Involved mainly in locomotion and predictive (feed-forward) postural control. Tectospinal Coordinates head and eye movements. Connects midbrain tectum superior colliculus with motor nuclei of cranial nerves and cervical region of the spinal cord. Mediates reflex postural movements of head in response to visual (tectum/superior colliculus) and auditory (inferior colliculus) stimuli. Vestibulospinal The nuclei relay motor commands through the vestibulospinal tract in response to vestibular input. Function of these motors commands is to alter muscle tone, extend, and change the position of the limbs and head with the goal of supporting posture and maintaining balance of the body and head. Direct projections from vestibular nuclei to the spinal cord ensure a rapid compensatory feedback response to any postural instability detected by vestibular apparatus. Summary of pyramidal and extrapyramidal pathways The pyramidal pathways accomplish skilled, discrete muscle actions. The extrapyramidal pathways provide the framework (muscle tone, posture, regulation of reflex activity). The lower motor neurons are referred to as the final common pathway. Both pathways control lower motor neurons. These lower motor neurons may receive both excitatory and inhibitory stimulation from both pathways. The sum of this input determines the final response of an individual neuron in the pathway. In case of spinal cord injury the overall dominating effect is loss of excitatory input on alpha motor neuron, loss of inhibitory input on gamma motor neuron. Spinal cord transection and spinal shock When the spinal cord is suddenly transected, essentially all its functions, including spinal cord reflexes, immediately become depressed. This is called “spinal shock”. There is complete loss of all reflexes, no muscle tone, paralysis, complete anaesthesia. The cause is sudden cessation of tonic input upon spinal cord interneuron pool which is normally present and transmitted via pyramidal and extrapyramidal tracts. Period of spinal shock is couple of days in humans. It may vary depending on the level spinal cord injury. The more complex is the animal the greater is the spinal shock period. After the spinal shock During recovery from spinal shock, the excitability of spinal cord reflexes increase due to the lack of descending inhibition and possible denervation hypersensitivity. Reflexes will reappear, but mostly exaggerated. Strong afferent stimuli from one of paralysed body parts may irradiate to other reflex centres including autonomic. Descending pathways - Tectospinal tract