L1-Intro to kidney -body fluids - clearance Rohacs 2023-dental.pdf
Document Details
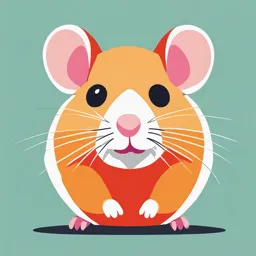
Uploaded by SmartestThermodynamics
2023
Tags
Related
Full Transcript
Kidney Lecture 1: Introduction to the Kidney Body Fluids and Clearance Tibor Rohacs MSB, H 631 2-4464 [email protected] 1 Kidney lectures L1. Introduction to the kidney: body fluids and clearance L2. Glomerular filtration and renal hemodynamics L3. Sodium balance L4. Water balance L5. K+ Ca2+...
Kidney Lecture 1: Introduction to the Kidney Body Fluids and Clearance Tibor Rohacs MSB, H 631 2-4464 [email protected] 1 Kidney lectures L1. Introduction to the kidney: body fluids and clearance L2. Glomerular filtration and renal hemodynamics L3. Sodium balance L4. Water balance L5. K+ Ca2+ and phosphate balance, reabsorption of nutrients L6. Acid base balance, secretion, micturition 2 What do the kidneys do? Homeostasis: any self-regulating process by which biological systems tend to maintain stability while adjusting to conditions that are optimal for survival. If homeostasis is successful, life continues; if unsuccessful, disaster or death ensues. 3 What do the kidneys do? Regulation of body fluids, electrolytes and minerals (H2O, Na+ , K+, Ca2+ , PO4) Removal of waste / toxic substances Acid Base balance Endocrine: – erythropoietin – calcitriol = 1,25-(OH)2 D 3 – renin So the kidneys regulate body fluids and electrolytes and minerals such as water, sodium, potassium, calcium, and phosphate content. The whole idea is if you have too much water, you know exactly what happens after drinking two liters of water. You're going to pee most of it out. So basically what the kidney does, if you have too much of something, it excretes it to the urine. If you have too little, it holds onto it as much as possible. That's kind of the major function of the kidney. Another important function, which I'm sure all of you know, is to eliminate waste and toxic substances. You can look at waste and toxic substances in a way that for the first row, for potassium and sodium and calcium content, you have an optimum. That's what the kidney is aiming for. For waste compounds and toxic substances, your set point is zero. You just want to get rid of them. The kidney does that quite efficiently as long as certain compounds filter well. The third function, we're going to dedicate a whole lecture to it, is acid-base balance. In a way, acid-base balance is also part of this general regulating of body fluids and electrolytes concept. Which is here, the regulated thing is protons. Basically acidbase balance is about free proton concentration in your plasma. If I wanted to, I could put protons here into this list, then this would not be a separate one. But generally, didactically, people discuss acid-base balance separately from other electrolytes. The fourth function of the kidney, again, the first three are all in the context of generating urine. If you have too much acids, you get excrete into the urine. Any waste substances end up in your urine. If you have too much sodium, potassium, calcium, you increase the concentration of those in the urine. All the first three functions are in connection to generating urine. The fourth function, the endocrine function, is sort of different. It has little to do with urine. Erythropoietin, which is a bloodgenerating hormone, just happens to be made in the kidneys. We're not going to discuss this in too much detail, but the reason it's listed here, if somebody has a kidney failure and lose both of their kidneys, they're going to have all sorts of electrolytes problems, but they're also going to have anemia because of the loss of erythropoietin. Calcitriol, the final version of this is active vitamin D3. The final version of the active version is also made in the kidneys. If you have somebody has kidney problems, in addition to electrolyte balance, they’re going to also have problems with active vitamin D3 levels, and which could cause bone problems. This is going to be... I'm going to mention this a little bit at the calcium lecture. And finally, renin, which is the first step in the renin angiotensin to aldosterone system, is going to be discussed in detail in later lectures. 4 How do they do it? How do the kidneys regulate electrolyte balance? I already sort of mentioned that everything that we don't need ends up in the urine, and the way it goes, arterial blood goes into the kidney. The kidney does some kind of magic with it, and the venous blood goes out of the kidney, and whatever didn't end up in the venous blood will end up in the urine. So basically, the kidney generates urine from your blood, and the next slides are going to show in detail how. But you can imagine if you have too much sodium, then the entering blood enters the kidney, and a little bit less sodium is going to leave through the vein, and sodium will be put into the urine. And we can do the same thing with anything else. 5 How do they do it? Nephron 1. Filtration (180 L /day) 20 % of Renal Plasma Flow (RPF) 2. Reabsorption (178/179 L /day) 3. (Secretion) 4. Excretion (1-2 L /day urine) From: Rhoades & Bell: Medical Physiology Glomerulus Proximal tubule Loop of Henle Distal tubule Collecting duct If you just look at the kidney as a black box. At the microscopic, more mechanistic level, what the kidney does, it's best explained on this extremely oversimplified picture of the nephron. The nephron is the microscopic unit of the kidney. I think you did have histology lectures by Dr. Muresan, so you know how the kidney looks in and out microscopically and macroscopically. This is not how a nephron actually looks, but it's easy to explain the basic steps on it. So the first step in kidney physiology is filtration. So you have the glomerular capillaries, and through these glomerular capillaries, the kidney filters a bizarre amount of fluid, 180 liters per day. That's a large volume. It filters. If you filter 180 liters of your plasma, the vast majority of it has to be reabsorbed. Otherwise, you would end up losing enormous amounts of fluid. So the first step is filtration, and the filtered fluid enters a tubular system, and the vast majority of what filters is reabsorbed. That's the second step. You can see 180 liters filtered, 178, 179 liters reabsorbed. And much of what is regulated in kidney physiology is the reabsorption step. So for example, sodium, if you have too much sodium content in your body, then what happens is the kidney will filter more or less the same amount, but it's going to reabsorb a little less, and more sodium will be excreted in your urine. So this is the most important regulated step, reabsorption. That being said, certain substances, certain electrolytes like potassium and protons are actually moved back from the interstitium into the tubular fluid that the opposite of reabsorption is called secretion. So secretion is then from the interstitial fluid, you pump things back to the tubular lumen. So these are the three major steps. And also note that the movement of reabsorption and secretion goes from the interstitial fluid, which is basically the space in between your cells, into the tubular lumen or backwards. And of course, whatever is in the interstitium eventually will equilibrate with your blood. And in the case of the tubular system, that is the peritubule capillaries, which we’re going to talk in more detail about. This is a super oversimplified nephron. In reality, you have the glomerulus and this straight tube here is divided to proximal tubule, the loop of Henle, distal tubule, and the collecting ducts, which I'll show in the next slide. Just before we leave this, the final step, excretion, is whatever is excreted, that’s what ends up in your urine. Excretion and secretion is easy to mix up. It's a popular exam question. That's why I put this little mannequin here to make you remember that these two words are very similar and make 6 sure you pick the right one on any sort of exam situation. 6 The Nephron Berne & Levy Physiology, 7 th ed, 2018 So this is how a nephron looks. You looked at much more detailed pictures of the nephron in your histology lectures, but still I'm going to refresh your memory. So this is the glomerulus where the filtration happens. A tubular system starts with the proximal tubule. The proximal tubule has these really thick cells and lots of mitochondria and this brush border, which increases the surface. All of these, lots of mitochondria and increased surface by the brush border is a sign of large amount of fluid movement. Mitochondria you need to produce the energy and increase surface. You can imagine if you have more surface, you can move more stuff. Interestingly, this proximal tubule then goes down to the deep medulla of the kidney in these thin segments. The descending thin segment and the ascending thin limb goes back to the cortex. This is also called the loop of Henle. And then you're going to have this thick ascending limb, which again contains a lot of mitochondria, lots of active transport. And then it moves back again to the medulla through these collecting ducts. So this is the basic structure of the nephron. I recommend that you go back to the histology lectures if you want to have a more detailed view. But this is more or less what you need to know for the physiology lectures. 7 Epithelial Transport (reabsorption) Apical (tubular lumen) Basolateral (interstitium à peritubular capillaries) Boron & Boulpaep Medical Physiology Transporter (or channel) Transcellular Tight junction Paracellular Peritubular capillary So when we talk about moving electrolytes back and forth from the tubule, that is classical epithelial transport. And the way it goes, epithelial cells are connected to each other through tight junctions. And then you have a transcellular movement in an epithelial cell. You have, for example, sodium ion entering the cell through either a transporter or a channel, and then usually it leaves the cell on the other side on some other kind of transporter. This vectorial transport, you're going to have a lot of different examples of this with specific transporters and channels. This is called transcellular because things move through the cells. You also have another kind of mechanism, which is called paracellular, where things move through the tight junctions, and you can also have examples of this in specific lectures for sodium, potassium, and other things. The convention is that the luminal side, the luminal side of the cell which faces the lumen of the tubule is called either tubular or apical side. The apical because these cells have this typical shape and the apex, the thinner part, is facing the lumen. That one is called apical. And the side that faces the interstitium is called the basolateral side, basal or lateral also because the tight junctions tend to be closer to the apical side. So this lateral side is continuous with the basal side. This is how it kind of looks on an abstract cellular level. If you put this back into the context of the simplified nephron, again, if you have reabsorption, you move from the tubule to the interstitium. And as I mentioned earlier, whatever is moved into the interstitium will end up in the peritubule capillaries and goes back to the circulation. 8 Osmosis: Water movement through a semipermeable membrane, driven by concentration difference of impermeable solutes So you already had a full lecture on osmosis with Dr. Shirokov. So this is going to be a very brief overview. So osmosis happens when you have a semipermeable membrane that separates two compartments. And if the concentration of an impermeable solute on one side is higher than on the other, what's going to happen? Water will move. And will move until it reaches equilibrium, until basically the concentration of the two sides is going to be equal. 9 Osmosis: Water movement through a semipermeable membrane, driven by concentration difference of impermeable solutes Osmolarity: number of particles / L solution Osmolality: number of particles / kg solvent Tonicity: hydrostatic pressure osmotically active particles exert I will use them interchangeably…. – milliosmoles/L = mOsm – milliosmoles/kg water = mOsm Reference point in renal lectures: normal plasma osmolarity isotonic = isosmotic osmotic cc = plasma hypertonic = hyperosmotic osmotic cc > plasma hypotonic = hypoosmotic osmotic cc < plasma Now technically this statement was not correct, because it's going to be an equilibrium between the hydrostatic pressure. So the hydrostatic pressure is going to act against this equilibrium. So theoretically, the concentration of two sides are not going to be exactly equal, because the hydrostatic pressure counteracts it. But in reality, at the concentrations we talk about in kidney physiology, the hydrostatic pressure is exerted by osmotic forces so high that you can basically neglect the counter effect of this higher hydrostatic pressure on this side. So you can just basically consider it to be completely equal on the two sides in terms of concentration. A couple of small concepts, osmolarity stands for the number of particles per one liter solution. Osmolality, number of particles per kilogram solvent. In the range again where we talk about osmotic concentration in the human body, these are essentially the same. Tonicity is the hydrostatic pressure osmotic reactive particles exert. These three are just calling osmosis on three different names. I will use these interchangeably. And one important thing in kidney physiology and general physiology with osmosis is that the reference point is the plasma osmolarity. So when we say something is isotonic or isosmotic, that means it's the same concentration as in the plasma. If we say hypertonic or hyperosmotic, it means that the concentration, osmotic concentration is higher than in the plasma. And then we say hypotonic, that means the osmotic concentration is lower than in the plasma. So the reference point is normal plasma osmolarity. 10 Colloid osmosis (oncotic pressure) Impermeable solute is a colloid (protein) Semipermeable membrane is capillary wall – Impermeable to protein – Permeable to electrolytes So when we talk about osmosis in physiology, we talk about the osmotic effect of small molecules or electrolytes. The most important of them being sodium, because sodium is the highest concentration of iron in your plasma. So when we talk about osmosis, almost always we talk about sodium. Other things have osmotic effects, but normally, sodium is so much higher than anything else than much of the osmotic force is basically sodium. And when we talk about osmosis, we talk about the water movements will happen in between the intracellular and the extracellular fluid, because cell membranes are freely permeable to water. But quantitatively, in the first approximation, they're not really permeable too much to sodium. We'll come back to this. But there's another kind of osmosis, which is called colloid osmosis, which you must have heard from cardiovascular physiology, where the boundary is not the cell membrane, but the capillary wall. And the capillary wall is fully permeable to sodium, glucose, and everything that's small, but it's not permeable to what? Proteins. So colloid osmosis is exerted by proteins, because the capillary members are practically not permeable to proteins, but they're fully permeable to electrolytes. Which force is larger, osmotic forces in the human body or colloid osmotic forces? Which one exerts higher hydrostatic pressure? What do you think is higher, the molar concentration of sodium or the molar concentration of proteins in your blood? Sodium. So if we compare these two in hydrostatic pressures, the hydrostatic pressure that's exerted by osmosis is extremely high, it just basically water freely moves. So the hydrostatic pressure almost never counteracts normal osmotic forces. It's not the case with colloid osmotic pressure, as you learned from cardiovascular physiology. And as we will discuss, normal blood hydrostatic pressures are actually comparable to the osmotic forces that protein exerts. So there's going to be an equilibrium between hydrostatic and colloid osmotic pressure for that reason. 11 Major Body Fluid Compartments Total Body Water (TBW) = ECF + ICF 60:40:20 rule ~60% Body weight = ~ 42 L (70 kg male) Intracellular (ICF) ~40% of Body Weight (28L) Extracellular (ECF) ~20% Body Weight (~14 L) Blood Plasma ~ 25% of ECF (~3.5 L) Interstitial fluid: ~75% of ECF (ECF – Plasma ~10.5 L) Blood = plasma + red blood cells (RBC) Hematocrit (Hct) = VRBC/Vblood (~0.45) Blood volume = Vplasma /(1-Hct) So the major body fluid compartments, the two of them, the extracellular fluid, which is outside your cells, and the intracellular fluid, which is inside of your cells. The intracellular compartment is larger. So the total body water is 60% of your body weight. The intracellular fluid is 2 thirds of that, 40% of your body weight. And the extracellular fluid is 20% of your body weight. So your intracellular fluid is roughly twice as large as your extracellular fluid. The extracellular fluid can be divided into blood plasma, which is the cell-free part of your blood, and to the interstitial fluid, which is basically the fluid compartment in between your cells, but not in your blood vessels. And the interstitial fluid is roughly three times as large as your plasma. The blood, you think of it as extracellular, but it's actually partly intra-partly extracellular because roughly half of it is red blood cells. So whatever is inside your red blood cells is technically intracellular. And you must have heard about hematocrit ratio, which is the volume of red blood cells as a fraction of your blood volume. It's somewhere around 0.45. And you can calculate your blood volume from the plasma volume by dividing it with 1 minus hematocrit. So these numbers are good to remember because it gives you an idea of how much water movement happens, how large are these compartments compared to each other. And this is going to be important in the regulation of certain electrolytes, such as potassium. 12 Electrolyte Composition of Body Fluids Extracellular Fluid ECF (mM) Plasma Interstitial Fluid (ISF) Intracellular Fluid ICF (mM) [Na] = 140 [Na] = ~10 [K] = 4.2 [K] = 120 [Cl] = 100 [Cl] = ~20 [Ca] = 1.1 [Ca] = 10-4 290 mOsm 290 mOsm Boundaries: Capillaries: impermeable to proteins, permeable to water and electrolytes (colloid osmosis) Cell membrane: impermeable to electrolytes, permeable to water (aquaporins) (osmosis) So what is the composition of these fluid compartments? So this picture shows the plasma, the interstitial fluid, which is together the extracellular fluid. It's the boundary between them is the capillaries. The composition is actually quite similar. The only difference between them is that you have more protein in the plasma than the interstitial fluid. And both fluids are largely containing sodium and chloride and some bicarbonate and much less potassium than sodium. On the other hand, the intracellular fluid, which is separated from the interstitial fluid by the cell membranes, is largely potassium based. You have a lot more potassium than sodium. Of course, you have phosphates, you have proteins, and all sorts of things. So what is the mechanism? Why do we have much more potassium inside than outside and sodium vice versa? What is the mechanism by which this happens? The sodium-potassium ATP is a sodium-potassium pump for some evolutionary reason. It's essentially sitting there in the membrane of essentially every living cell. And so your inside is potassium based, your outside is sodium based. And that's the reason why the major osmotically active ion outside is sodium. And it will have an importance in regulating potassium. Your potassium levels are generally very low. Chloride levels usually go together with sodium except in some acid-base differences. Now calcium is another one which is interesting. You have 1.1 millimole free on average outside. And you have orders of magnitude less inside your cells. And the relevance of this is that calcium can be easily used as a signaling ion because if you have 10,000 times more outside, if you just open one or two calcium permeable channels, calcium will shoot up. And that's how it heartbeats, that's how muscles contract, etc, etc.. So nature made use of the fact that you have so much less calcium inside your cells that is used as a signaling ion. So again, the boundaries are the capillaries in between your plasma and your interstitial fluid. And this is where water movement is determined by colloid osmosis. And your cell membrane is the boundary between intra and exoskeleton fluid. And there, osmosis determines the movement. And you will see in the water lecture the reason why, the major reason why cells are so permeable to water is the presence of aquaporins, which are these water permeable ion channels. 13 Darrow-Yannet Diagram osmolarity 300 150 ECF Visual representation of changes in volume and osmolarity of ECF and ICF ICF 0 14 L 28 L 300 150 ECF ICF 0 16 L Initial 28 L osmolarity osmolarity A: Drinking 2L of water 300 150 ECF ICF 0 14.67 L 29.33 L After equilibrium -Primary change always happens at the ECF, osmotic concentration will equilibrate by water movement between ECF and ICF Now this slide shows the Darrow-Yenet diagram, which is a nice visual representation of the changes in the volume and osmolarity of your exoskeleton and intracellular fluid. The way it looks, your vertical axis, so to speak, it's not that easy to draw with this. This is showing osmolarity, and the horizontal shows the volume. So the cyan, the light blue part, is your exoskeleton fluid, and the yellow one is your intracellular fluid. As I mentioned earlier, your intracellular fluid is around 28 liters. Your exoskeleton is around 14 liters. So the next couple of slides is going to show you changes, what happens to one and the other compartment, if you do certain kinds of interventions. The first one is a very, very common one. Let's say you drink two liters of water. So when you drink two liters of water, it's going to be absorbed from the intestines, and it's going to reach your plasma, your blood. So the idea is that the primary change always happens in the exoskeleton fluid. You can't put some things magically inside your cells. It has to reach your blood first. So what happens is anything that changes first changes in the exoskeleton fluid, and then what is going to happen is there's going to be a secondary water movement in between your extra and intracellular fluid that will equilibrate the two sides to have the same osmotic concentration. So this one, this example of drinking two liters of water kind of artificially divides this into two steps, which of course doesn't happen because as soon as your water is reabsorbed, it's going to also move into your cells. But just for the theoretic reasoning, I divided this into two parts. So after you drink two liters of water, your excess fluid's volume is going to be higher, 16 liters. But since you dilute your sodium and everything that's osmotically active, the concentration will be lower. That's what's shown on this side. So your excess fluid will be higher in volume but lower in osmotic concentration. And after actually bracing the two sides, letting water freely flow from between the two sides, what is going to happen is two thirds of the water that you drank is going to go inside your intracellular fluid. And from the origin of 14 liters, you're going to have 14.67 liters despite the fact that you drank two liters of water because two thirds of it went intracellularly. 14 Darrow-Yannet Diagram osmolarity 300 150 ECF Visual representation of changes in volume and osmolarity of ECF and ICF ICF 0 14 L 28 L osmolarity B: Isotonic volume expansion by 2 L – no changes in ICF because no osmotic gradients, therefore no fluid movements between ECF and ICF 300 ECF 150 ICF 0 16 L 28 L So next example is another one. You go to the hospital and for whatever reason, they put you in an IV line and infuse you with two liters of isotonic saline. If that happens, then because the saline is isotonic or isosmotic, there's not going to be any net water movement because you introduced something that was isosmotic. And therefore, if you get an IV injection of two liters of isotonic saline, your volume is going to change from 14 to 16 liters, no water movement because there's no osmotic force developed because you entered the same osmotic concentration. This is probably the simplest of the three. 15 Darrow-Yannet Diagram osmolarity 300 150 ECF Visual representation of changes in volume and osmolarity of ECF and ICF ICF 0 14 L 28 L 300 150 ECF ICF 0 14 L 28 L Initial osmolarity osmolarity C: Eating 18g of table salt (NaCl) 300 150 ECF ICF 0 15.27 L 26.73 L After equilibrium Isotonic saline is ~9 g/L NaCl If you just magically put salt into your blood, your osmotic concentration is going to be very high. And then after equilibration, that sodium is going to attract a lot of water out of your cells. So the end result, if you eat 18 grams of salt, the end result will be even if you don't drink anything, your original 14 liters will increase to 15.27 liters. We can do the calculations, but I saved you that. If you're interested, you can email me how to calculate this or figure it out by yourself with 300 mOsm per liter as a standard concentration. But the bottom line is that how much sodium you entered will correlate very well with your volume. 16 Darrow-Yannet Diagram osmolarity 300 ECF 150 Visual representation of changes in volume and osmolarity of ECF and ICF ICF 0 14 L 28 L EC volume Drink 2 L water 14 L à 14.67 Eat 18 g salt 14 L à 15.27 2 L isotonic saline 14 L à 16 L Conclusion: the Na+ content of the ECF is an important determinant of its volume, due to osmotic water movement If you just drink two liters of water, the increase in your exo fluid is going to be only 670 milliliters, which is less than if you eat salt without any water. And of course, the highest change will happen when you input both salt and water. The 18 grams in two liters is the same as same sodium content as two liters of isotonic saline. So these examples serve to illustrate that sodium has a very important role in regulating your plasma volume. It's very important, the sodium content is a very important determinant of how much volume you have in your plasma due to osmotic water movements. 17 Conclusion: Na+ regulation ~ ECF Volume regulation Na+ content of ECF ↑à hypervolemia Na+ content of ECF ↓à hypovolemia Sensor of Na+ regulation are primarily volume (pressure) sensors, Major regulator: renin-angiotensin-aldosterone system Conclusion: Water regulation ~ Osmoregulation H2O content of ECF ↑à hyposmosis H2O content of ECF ↓à hyperosmosis Sensors of water reabsorption are primarily osmosensors Major regulator: vasopressin (ADH) And the reason why I'm trying to emphasize this is because if you have disturbances in the sodium content of your ECF, for example, if the sodium content of your ECF goes up, for example, you eat a lot of salt and your kidneys can't keep up with getting rid of it, the primary symptom is going to be, it's not going to be high sodium concentration in your blood because sodium brings out water from your cells and you're going to be thirsty and you're going to be drinking. So if you eat a lot of salt, the problem is usually hypertension. Normally, the kidneys can handle it for a while, but if you just eat a ton of salt sooner or later, you're going to end up with hypervolemia, which also cause hypertension at the end. If you, for some pathologic reason, lose a lot of sodium, the end result usually will be hypovolemia because sooner or later, your plasma cannot hold up to enough water and you're going to have hypovolemia. And we're going to have a whole lecture dedicated to this. The sensors for sodium regulation are primarily osmosensors or pressures, sorry, primarily volume and pressure sensors. And the key regulator is going to be the renin angiotensin aldosterone system. And the other one, the other important take home message from this is that if you regulate the water content, that's going to be more important in osmo regulation than in volume regulation because water itself is not a very efficient way to increase your volume because you need sodium to keep it in the excess of fluid. And so if you have, if your water content in the ECF goes up, the primary symptom is going to be hyposmosis and vice versa. If your water content goes down, the problem will be hyperosmosis. And the primary sensors for water reabsorption are going to be osmosensors and the key hormone that regulates it is going to be antidiuretic hormone or vasopressin. Again, we're going to dedicate a full lecture to this in the water balance lecture. 18 Clearance Volume of plasma cleared from a given substance in a given time C U P V Clearance Concentration in urine Concentration in Plasma Urine flow / time The last part of this lecture is the clearance principle. The clearance, let's start with the definition. You can have some examples later to see what a clearance principle is good for, but let's define it first. So clearance, abbreviated as C, is the volume of plasma cleared from a given substance in a given time. So it's basically a volume of plasma cleared from a given substance at a given time. It's kind of a virtual volume of plasma and one of the reasons the clearance principle is introduced because if you say I got rid of two grams of glucose or got rid of two grams of sodium, you can't compare them because you have a lot more sodium in your blood than glucose. If you convert this to how much plasma volume they took, then everything is going to be in the same unit. So clearance makes it easy to compare how much of something you excreted because you convert all of them to the volume of plasma that they are contained in. So that's one of the reasons the clearance principle is introduced. So let's look at the formula and the formula hopefully will make it clear for most of you what clearance is. If not, the next slide is going to give an alternative explanation. So let's say so U is the concentration of something in your urine, P is the concentration of the same thing in your plasma. U is urinary concentration, please, plasma concentration, and V is the urine flow. So let's say just for the example that you have substance X and the concentration of substance X in your urine and your plasma is exactly the same. What will be this equation? C will equal, so if this is the same as this, we'll get rid of it, so it's going to be V. And it kind of makes sense. If you have the same concentration of something in your urine than in your plasma, you cleared exactly that volume of plasma from that substance, right? Let's look at example two. You have 10 times higher concentration in your urine. How much plasma did you clear? If U is 10 times P, what's going to be the value of C? 10V, exactly. So if you have 10 times more higher concentration of urine, you cleared 10 times your urine flow, right? If you have 100 times more, you cleared 100 times more. If it's 5 times more, you cleared 5 times more. Does it make sense? So the higher the concentration of any substance in your urine compared to your plasma, that means you cleared a higher volume from that substance 19 Derivation of clearance Arterial input Px,a * RPFa Venous output Px,v * RPFv Arterial input = Venous output + Urine output Px,a * RPFa = Px,v * RPFv + Ux * V Urine output Virtual arterial input Px,a * Cx virtual venous output = 0 actual urine output + Ux * V Replace RPF with virtual RPF, i.e. the volume that is totally cleared of the substance RPF = Renal Plasma flow From Boron Boulpaep: Medical Physiology So this is an alternative explanation. This is a good slide. You don't have to remember any of it. As long as you remember the clearance principle, the clearance formula, you're fine. But some people find this explanation a little more easier to digest than just playing this proportion game with the formula. So you're going to derive the principle of clearance here. This derivation is based on the simple idea that whatever goes into the kidney should come out of the kidney. So this is only true for non-metabolized substances. So that being said, your arterial input should equal your venous output and your urine output. And if you quantify this, your arterial input should equal your renal plasma flow, RPF is renal plasma flow, times the concentration of substance X in your arteries. So whatever amount of plasma flows your kidney, if you multiply this with the concentration of the substance, that's going to be equal of how much of that substance went into the kidney. The venous output will be the renal plasma flow on the venous side times the concentration in the venous blood here. And the amount that goes into the ureter is going to be the urine, is going to be the urinary concentration times urine volume or urine flow. Now to derive the principle of clearance, you need to make a sort of a conceptual leap. And instead of having a real kidney that you perfuse with the renal plasma flow, you replace this real renal plasma flow with a virtual renal plasma flow for a volume that is completely cleared from that substance. So instead of real plasma flow, you replace the plasma flow with the volume that is completely cleared from that substance. And the volume of plasma that is completely cleared from a substance is actually clearance. That's how defined it in the previous slide. But if you completely cleared from that particular substance, that means there shouldn't be any in the venous blood. So this middle part will be zero. And then you basically got the clearance formula because p times c equals u times v. Then you just solve it to c, divide the two sides at p, and it's u times v times p. I don't know if you've made it clear or not. The bottom line, you need to remember the clearance principle and you need to understand what clearance means. 20 Clearance substances filtered, not reabsorbed, not secreted Inulin, a polymer of fructose (MW~5500) is freely filterable but neither reabsorbed nor secreted The clearance of inulin is equal to the volume of plasma filtered per unit of time, i.e. the Glomerular Filtration Rate (GFR) U *V CIn = In = GFR PIn Filtration (GFR = 125 ml / min = 180 L / day) Urine flow (V) = ~ 1 ml / min ~ 1.5 L / day So what is clearance good for? Again, just because we can compare glucose to sodium of how much we cleared from it doesn't seem like a super useful thing. The most important use of the clearance principle is actually to calculate or measure the glomerular filtration rate. The glomerular filtration rate is a very important clinical parameter of kidney physiology because usually when the kidneys fail, the glomeruli stop filtering. They got sclerotic or whatever, but they stop filtering. So when clinicians want to quantify kidney function, they want to know what the GFR is. They want to know what the glomerular filtration rate is. And that can be measured. This was measured first probably more than 100 years ago in experimental animals. And then people figured out how to do this in humans. So the way to use the clearance principle to calculate the glomerular filtration rate is to take a substance that is water soluble, so it's well filtered, and neither reabsorbed nor secreted. So if you take a substance, it just filters perfectly, enters the tubular fluid, and the kidney doesn't do anything with it after it's filtered. Neither reabsorbs nor secretes, right? So if you find such substance, then it means the kidney will clear all the plasma that was filtered from that substance. So if you calculate the clearance of this substance, the clearance of this substance will be equal to the glomerular filtration rate, because whatever was filtered is cleared because it neither secreted nor reabsorbed. So all that entered the tubular fluid will leave with the urine. And such substance is the first one that was used in animal experiments is called inulin. It's a polymer of fructose, a relatively high molecular weight, so there's no transporter that can take it back, but it's small enough to be freely filtered. So people many, many, many years ago just infused inulin into experimental animals, measured what was in the urine, measured what was in the plasma, and calculated. And they were probably surprised to see how incredibly high the glomerular filtration volumes were. So this we will come back on the next lecture in a little bit more detail. 21 Clearance – filtered and reabsorbed substances 1. Many substances are removed from the tubular fluid and returned to the blood – reabsorption C < GFR 2. Glucose is completely reabsorbed by the kidney tubules - its concentration in the urine is zero and therefore 3. Na+ Cglucose = 0 is freely filtered, then reabsorbed, but not completely 0< CNa