كتاب علوم متكاملةver 1 PDF
Document Details
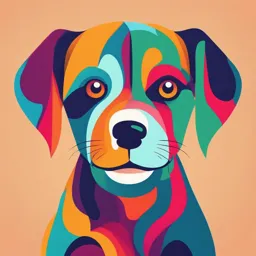
Uploaded by CoolMossAgate3527
Elowa
Tags
Summary
This document is a lesson on aquatic ecosystems, focusing on the chemical and physical properties of water, the water cycle, and the role of oxygen and carbon dioxide in aquatic environments. It includes discussions on water density, solubility of gases, and various biological adaptations of aquatic organisms.
Full Transcript
Aquatic ecosystems Lesson (1) 1-1 Chemical reactions and their impact on water quality (a) Water has a unique property: 1) Can dissolve most of the chemical compounds. 2) Exists in three states [Solid, Liquid, Gas]. 3) Can pass through the...
Aquatic ecosystems Lesson (1) 1-1 Chemical reactions and their impact on water quality (a) Water has a unique property: 1) Can dissolve most of the chemical compounds. 2) Exists in three states [Solid, Liquid, Gas]. 3) Can pass through the cell carrying all the needed nutrients to produce energy. (b) The hydrosphere which indicates the water in its liquid state: 1) Water represents 72% of Earth's surface. 2) 97% of water is salt in oceans and seas. 3) 3% of water is fresh water in lakes - 1% from ground water. 1 Water Cycle 1. Water exists on or near the Earth's surface in a state of continuous change between three states. 2. Water cycle, hydrologic cycle can change the Earth chemically, physically, and biologically. 3. Water cycle consists of: A) Evaporation, which contributes to the formation of clouds. B) Condensation, in which the water vapor cools down. C) Precipitation, in which the rain falls or the snow falls. 2 D) Some water runs on the surface in the seas and oceans. The other enters things small pores in the sedimentary rocks to form underground water. Chemical Structure of Water (a) Water is composed of two elements: hydrogen and oxygen. These elements combine in a ratio of two hydrogen atoms to one oxygen atom. (b) Oxygen constitutes approximately 88.8% of the mass of a water molecule, while hydrogen accounts for the remaining 11.1%. (c) The two hydrogen atoms are bonded to the oxygen atom through covalent bonds. (d) The angle formed between these bonds is approximately 104.5 degrees. 3 Water Polarity: a) Oxygen has a higher electronegativity than hydrogen. b) Electrons are attracted to oxygen atoms. c) A partial negative charge forms on the oxygen atom, and a partial positive charge forms on the hydrogen atoms. d) Water's polarity allows it to form hydrogen bonds, which enable water to dissolve salts into hydrated ions. Example: NaCls + H₂Ol → Na⁺ aq+ Cl⁻ aq 4 Hydrolysis Hydrolysis is a chemical process in which a molecule of water is added to another compound, breaking it down into smaller components. Water consists of [H+] and [OH-] Example: NaCl (hydrolysis): Neutral solution - [H+] = [OH-] NaHCO₃ (hydrolysis): Basic solution - [H+] < [OH-] NH₄Cl (hydrolysis): Acidic solution - [H+] > [OH-] pH Value The pH value is a measure of the acidity or basicity of a solution or liquid. pH < 7: Acidic solution pH = 7: Neutral solution pH > 7: Basic solution pH Values of Common Substances: Seawater: 7.5 to 8.4 Freshwater: 6.5 to 8.5 Distilled water: 7 Groundwater: Varies according to the type of rock 5 Practical Activity of pH 1. Prepare water samples from different sources. 2. Fill beakers with the samples. 3. A pH meter or pH test strips are needed. 4. Cups of water as a reference. 5. Stirring rod. Procedures: 1. Calibration: Calibrate the pH meter. 2. Sample Preparation: Place samples in the beakers. 3. Testing: Immerse the electrode and record the reading. The Importance of Monitoring Salt Hydrolysis in Water Bodies Monitoring Salinity: Regularly monitor salinity levels in natural water bodies to assess the impact of salt hydrolysis. Monitoring Ionic Structure: Track changes in the ionic composition of water to understand how salt breakdown affects water quality. Proper Waste Disposal: Implement effective waste management practices to reduce the introduction of harmful salts into water bodies. Protecting Water Quality: Maintain water quality for both wildlife and human use by minimizing salt-related pollution. 1-2 physical properties of water and their role in the distribution of living Water's unique physical properties, like its lower density at freezing and high specific heat, influence natural phenomena and impact the distribution of living organisms in different environments. 6 Density Density is the mass of a unit volume of matter at a given temperature. It depends on the mass of molecules and their distances. For example, pure water has a density of 1 g/cm3, equivalent to 1000 kg/m3. As water's temperature decreases from 4oC to its freezing point, its density decreases. The relative density of a substance is the ratio between its density and pure water's density at the same temperature. A hydrometer is a sealed glass reservoir used to measure the density of liquids. It contains lead or mercury balls for vertical stabilization and is connected to a stem. The scale indicates the highest density and the lowest density. Practical activity: Measure the density of different samples of water Use a hydrometer to determine the density of water from different sources: (sea, river, canal, pond, lake, underground). Discuss how the hydrometer can be used to predict the presence of soluble pollutants in a sample of water. 7 Water density and water currents in the oceans: Water density in oceans is affected by pressure, salinity, and temperature. Pressure increases with depth, causing water molecules to be closer together, which increases density. Higher salinity results in higher water density, with normal ocean salinity at 35 grams per liter (or the equivalent of two teaspoons per cup of water). Lower water temperature (down to 4°C) causes molecules to come closer, the lower the volume they occupy and the higher the density of water. Differences in water density contribute to ocean currents. Water currents in oceans, caused by differences in water density, transport heat, salt, nutrients, and fresh water from the tropics to the poles and the surface. Polar Regions' Water Density: Water density in polar regions varies with temperature, generally the volume of a liquid increases as the temperature increases and the volume of a liquid decreases as the temperature decreases. 8 As the temperature rises from 0°C to 4°C, water shrinks, increasing its density to 1000 kg/m3. However, as temperatures rise above 4°C, water expands, causing its density to decrease. This is why lakes in polar regions freeze at the surface rather than at the bottom. When air temperatures are between 4°C and 0°C, surface water expands, becoming less dense than water below. The ice remains on the surface, while water remains near the bottom at 4°C, preventing fish and marine life survival. 9 Practical activity: The Effect of Density on Water Movement: Materials: Two identical containers Fresh water Salt water (with salinity equivalent to ocean water) Food coloring Ice cube trays Thermometer Procedure: 1. Prepare the ice cubes: o Fill the ice cube trays with fresh water and add a few drops of food coloring to each cube. o Freeze the trays until the ice cubes are solid. 2. Set up the experiment: o Fill the two containers with equal quantities of fresh water and salt water. o Place a colored ice cube in each container. 3. Observe and record: o Dissolution rate: Measure the time it takes for each ice cube to completely dissolve. o Water movement: Observe and note any visible movement of water around the melting ice cubes. Analysis and Conclusions: 10 Dissolution rate: Compare the dissolution rates of the ice cubes in fresh and salt water. Water movement: Analyze the direction and intensity of water movement in each case. Additional Considerations: Temperature: Ensure that both containers are at room temperature before starting the experiment. Salinity: Verify the salinity of the salt water using a hydrometer or salinity tester. Control variables: Keep all other variables (e.g., container size, ice cube size) constant. Oceanographic Implications: The results of this experiment can be applied to understand the movement of fresh water in the ocean. When icebergs melt, the less dense fresh water spreads out on the surface, forming a layer that acts as an insulator. This can affect ocean circulation patterns and climate. 1-3 Oxygen and carbon dioxide in the aquatic: Rivers and seas naturally contain oxygen and carbon dioxide for aquatic life of plants, fish, and microorganisms such as bacteria and algae. Oxygen is sourced from atmospheric air and photosynthesis by phytoplankton, algae, and aquatic plants. Ocean and seas waves and currents increase gas exchange between the atmosphere and water, providing marine creatures with the oxygen they need for survival. 11 Solubility of the two gases O2 and CO2 in water: The solubility of oxygen (O2) and carbon dioxide (CO2) in water varies depending on the temperature. Oxygen is 500 times more soluble in air than carbon dioxide, but 50 times less solubilized in water. In salty ocean water, their solubility is 20-30% lower than in fresh water. Higher temperatures decrease the percentage of CO2 in water. The graph shows the relationship between the solubility of oxygen and carbon dioxide in fresh water at different temperatures under normal atmospheric composition. The effect of increasing the percentage of dissolved oxygen in water: Enhances respiration: Increased oxygen in water improves aquatic organisms' ability to breathe. Improves metabolism: High oxygen levels support aquatic organisms' metabolism and growth. Increases activity: Adequate oxygen stimulates aquatic organisms' activity in swimming, hunting, and reproduction. Maintains ecosystem balance: A healthy dissolved oxygen level supports diverse aquatic populations. 12 Effects of Increased CO2 on Aquatic Organisms: Increased CO2 in water can have several negative effects on aquatic organisms, including: Acidification: High CO2 levels dissolve more in water, increasing carbonic acid and decreasing water pH. This harms sensitive life stages like egg and larval stages. Weak respiration: High CO2 levels reduce oxygen in water, affecting breathing. Reduced calcification: Increased CO2 converts calcium carbonate into calcium bicarbonate, disrupting skeleton building and maintenance in marine organisms like corals, mollusks, and plankton. The effect of CO2 deficiency in water on aquatic organisms: Reduced photosynthesis: Aquatic plants and algae need carbon dioxide for photosynthesis. Decreasing the availability of CO2 in water may limit their ability to produce energy, affecting the overall productivity of the ecosystem. Effects on food chains: A change in the level of CO2 in the water can affect productive organisms such as phytoplankton and algae, thereby affecting organisms at higher levels of the food chain. Disruption of pH balance: Low concentrations of CO2 may lead to an increase in the pH of water, negatively affecting sensitive species that are adapted to a certain pH range. 13 1-4 Biological adaptations of living organisms in the aquatic environment. Aquatic organisms possess various adaptations that enable them to thrive in their respective environments, whether in the deep ocean or shallow lakes. These adaptations can be physiological, behavioral, or structural, and they help the organisms adjust to different environmental conditions, ensuring their survival. This chapter will explore the diverse strategies aquatic creatures use to live and function in their habitats. Physiological Adaptations in Aquatic Life Aquatic organisms develop specialized physiological adaptations to survive in their unique environments by modifying how they perform essential biological functions. For instance, certain deep- ocean fish regulate respiration in oxygen-deficient conditions and have strong arteries and veins to withstand high water pressure. They can adjust their blood pressure to balance the external forces they encounter. A notable example is the Electric Eel, which thrives in low-oxygen depths by having large gills with fine capillaries that enhance oxygen. extraction, alongside the ability to slow its. metabolism to reduce oxygen demands. Osmotic pressure refers to the difference in solute concentration in a solution, causing water transfer from a dilute solution to a concentrated solution. This process occurs through a semi-permeable membrane, resulting in the diffusion of water from the less concentrated solution to the 14 more concentrated solution, thereby influencing the concentration of the solution. Practical activity: Measure the density of Materials: Sugar solution, thistle funnel, cellophane paper, glass beaker, tap water, rubber band, stand Procedure: Secure cellophane paper over funnel opening with rubber band. Fill funnel with sugar solution. Submerge funnel in water-filled beaker. Mark initial solution level in funnel stem. Observe changes over time. Observation: Solution level in funnel stem increases due to osmosis. Conclusion: Water moves from beaker to funnel to equalize sugar concentration. Freshwater Organisms' Adaptations to Low Osmotic Pressure Physiological adaptations: Freshwater organisms have developed physiological adaptations to manage the low osmotic pressure in their environment, which otherwise could cause excessive water intake, leading to cell rupture. These adaptations include: 15 Contractile Vacuole: A specialized organelle in unicellular organisms (e.g., amoeba, paramecium, and euglena). Water Collection: The vacuole collects excess water from the cell. Water Expulsion: Once filled, the vacuole moves towards the cell membrane to expel the water, preventing cell bursting. Maintaining Balance: This process helps the organisms regulate water intake and maintain internal equilibrium. Multicellular organisms, like fish, have developed mechanisms to eliminate excess water that enters their bodies through the skin, mouth, and gills. Their kidneys play a crucial role in this process by filtering the excess water and expelling it as dilute urine. Key aspects of this adaptation include: Kidney Function: The kidneys remove excess water and produce dilute urine. Water Entry: Water enters the body through the skin, mouth, and gills. Kidney Location: In fish, the kidneys are located in the abdominal cavity on either side of the spine. Maintaining Osmotic Balance: This process allows fish to regulate their internal water levels and maintain osmotic balance in freshwater environments. 16 While fish that live in saltwater (Saltwater fish) need to swallow large amounts of sea water to compensate for the osmotic loss of water from their body, and then they excrete excess salts through their kidneys and specialized cells in their gills. Sharks exhibit physiological adaptation to maintain water and salt balance by controlling urea levels in their blood due to their adaptation to high salinity ocean water. High Urea Concentration: Sharks maintain elevated levels of urea, a nitrogenous compound usually excreted by other animals. Osmotic Pressure Adjustment: The high urea concentration increases their osmotic pressure, making it similar to the surrounding seawater. Water Retention: This reduces water loss from the shark's body in the salty environment, helping to maintain the balance of water and salts. 17 Minimized Water Loss: The adaptation ensures sharks conserve water effectively in high-salinity conditions. Urea, a nitrogenous compound, is excreted in urine by many animals, including sharks. This increases osmotic pressure, bringing it close to the surrounding water, minimizing water loss from the body to the high-salinity environment. The high concentration of urea in their blood aids in this process. Behavioral adaptations: Behavioral Adaptations Overview Behaviors used to avoid extreme conditions. Utilization of resources. Example for behavioral adaption Salmon are born in freshwater, grow up in rivers, and then migrate to the sea where they spend most of their adult life. After reaching sexual maturity, they return to freshwater rivers to reproduce. A key process in their life cycle is "Smoltification," which enables the fish to transition from freshwater to saltwater. Salmon are born in freshwater. They spend their early life in rivers. Salmon migration Young salmon undergo "Smoltification" to adapt to saltwater. Adult salmon live in the sea. They return to freshwater rivers to reproduce. The ability of salmon to move between different environments is due to their ability to make complex physiological adaptations. For example, their circulatory and respiratory systems adapt to changes in salinity and different amounts of oxygen in fresh and salt water. 18 Structural adaptations: Structural Adaptations Overview Changes in organism's physical structure. Aids survival in environments. For example, fish that live in the deep ocean have very large eyes to be able to see in the dark, and their bodies are compressed to withstand the very high pressure in deep water. An example of a compressed deep-sea fish is the icefish, which lives in the cold southern oceans, at depths of about 2000 meters. Fish have several structural adaptations to help them live in water, including: A streamlined body reduces water resistance. Gills extract dissolved oxygen from water. Scales and mucus cover the body for waterproofing and reduced drag. Fins serve as movement organs. Bony fish have a swim bladder to help them float. Gas exchange and cellular respiration: Gas exchange is when an organism obtains oxygen from atmospheric air or the surrounding environment and removes carbon dioxide. Cellular respiration is a vital process in which the organism breaks down the bonds in food molecules, especially glucose, to obtain stored energy. Unicellular organisms, such as amoeba obtain oxygen and eliminate carbon dioxide through the cell membrane by diffusion. 19 1-5 The effect of temperature on the marine environment Heat and temperature: In everyday conversation, "amount of heat" and "temperature" are often confused, but they have distinct meanings in physics. Every object or system consists of numerous particles that are in constant motion. The total energy from the particles’ potential and kinetic energy is termed internal energy. The "amount of heat" specifically refers to the energy transferred due to a temperature difference and is measured in Joules. The international unit for temperature is the Kelvin (K), which is a quantitative description of the average kinetic energy of particles in an object or system. The value of temperature in Kelvin corresponds to its degree Celsius value. (TK = t °C + 273) (which means that 1 Kelvin is273 Celsius) The increase in temperature is attributed to the vibration of molecules, which increases the amplitude of their vibration and kinetic energy, thereby causing the temperature of the system to rise. 20 Specific heat of matter (c): The specific heat of a substance is the amount of heat required to raise the temperature of 1 kg of that substance by 1 K, measured in joules per kilogram per Kelvin (J/kg·K). A substance with a higher specific heat requires more thermal energy to increase its temperature compared to a substance with a lower specific heat. The amount of heat gained or lost by an object (Qth) can be calculated using the formula: Qth=m c Δt Qth where: m c Δt Qth: Amount of heat (in joules) m: Mass of the substance (in kilograms) c: Specific heat capacity of the substance (in J/kg·K) Δt: Change in temperature (in Kelvin) 21 Examples Ex1 Calculate the amount of heat required to raise the temperature of 0.3 kg of copper from 20 degrees Celsius to 70 degrees Celsius given that the specific heat of copper = 385 J/kg. K. sol: Qth = m c Δt = 0.3 × 385 ×(70−20) = 5775 J Ex2 A piece of aluminum with a mass of 200g and a temperature of 80 °C is dropped into a quantity of water at room temperature. If the final temperature of the system is 40 °C, calculate the amount of heat gained by the amount of water. The specific heat of aluminum is 897 J/kg. K sol: Based on the law of conservation of energy, the amount of heat gained by the water is equal to the amount of heat lost by the aluminum piece, assuming no thermal energy leaked or lost from the system. (Use international units.) QAl = mAl⋅cAl⋅ΔTAl QAl = (0.2 kg). 897 J/kg. K) ⋅ (40°C - 80°C) QAl = -7176 J The negative sign here indicates that the aluminum piece has lost heat to the water sample, so the amount of heat transferred to the water is 7176 J 22 Ex3 A block of iron with a mass of 500 g is heated from 25 °C to 100 °C. Calculate the amount of heat required for this temperature change. The specific heat of iron is 450 J/kg·K. sol: Using the formula for heat transfer: Qth=m⋅c⋅Δt we can calculate: QFe= (0.5 kg⋅450 J/kg. K) ⋅ (100−25) QFe=0.5⋅450⋅75 QFe=16875 J Thus, the amount of heat required to raise the temperature of the iron block is 16875 J. The importance of the high specific heat of water: Water's Specific Heat and Climate Water's specific heat is high, approximately 4200 J/kg K. Hydrogen bonds in water molecules contribute to its mild climate. Large bodies of water have lower summer temperatures than beach sand and rocks. Air over land heats up, becoming less dense, and rises upward. Sea breeze, cooler air above water surface, replaces hot air rising upward. (Sea breeze) 23 The effect of temperature changes on marine organisms: Marine organisms, such as coral reefs, require specific temperatures to survive in colder depths, and climate change can impact their distribution, as warm surface waters may not be able to survive in colder waters. The relative stability of water temperature is significantly influenced by the high specific heat of water, which allows it to absorb significant amounts of heat without significant temperature changes. Oceans and lakes serve as thermal reservoirs, absorbing solar energy during the day and releasing it at night, thereby maintaining stable marine temperatures. This thermal balance is very important for the sustainability of marine life. This property shields marine organisms, particularly cold- blooded creatures (Poikilotherms), from rapid temperature fluctuations, enabling them to reside in stable environments like deep seas and oceans. Their body temperature is influenced by the surrounding environment. 24 1-6 The effect of light and solar radiation on aquatic Diversing into the sea, observe light intensity changes, impacting organisms, photosynthesis, and solar radiation's role in ocean ecological balance. Solar radiation is the energy emitted by the sun, which is a major source of energy for Earth's systems. It can be converted into other forms of energy like heat and electricity using different technologies. The effectiveness of these technologies depends on the amount of solar radiation available. Key Features of Solar Radiation Source: Solar radiation originates from the sun. Energy for Earth: It's a primary energy source for Earth's atmosphere, hydrosphere, and biosphere. Conversion: Can be converted into other forms of energy (e.g., heat, electricity). Technology Dependence: The feasibility of solar energy technologies depends on the availability of solar radiation. 25 Visible light is a type of electromagnetic wave that can be seen by humans. It's part of a broader range of electromagnetic waves called the electromagnetic spectrum. Different wavelengths within visible light correspond to different colors, ranging from red to violet. Characteristics of Visible Light: Electromagnetic Wave: Visible light is an electromagnetic wave. Electromagnetic Spectrum: It's part of the broader electromagnetic spectrum. Wavelengths: Different wavelengths within visible light correspond to different colors. Visible Colors: The colors of visible light include red, orange, yellow, green, blue, indigo, and violet. Solar radiation reaching Earth can be classified into two categories: Solar radiation intensity at specific Earth's surface locations or objects is by factors like geography, season, time of day, cloud cover, and altitude. Direct solar radiation: This is the radiation that reaches the Earth's surface without scattering. Indirect solar radiation: This is the light which scattered while passing through the atmosphere. 26 Solar radiation and its effect on water: Solar radiation, Earth's primary energy source, directly impacts water layers, with some absorbed by water, suspended matter, and aquatic plants, while others scatter in depths. light zones in water: As the depth of water increases, the intensity of light gradually decreases. This change in light creates different zones in the ocean: 1. Euphotic Zone (Sunlit Zone): This is the upper layer where enough sunlight penetrates for photosynthesis to occur. Many marine organisms thrive here due to the abundant light. 2. Twilight Zone (Mesopelagic Zone): In this middle layer, light is limited but not completely absent. Some organisms can adapt to the lower light levels. 3. Aphotic Zone (Deep Zone): In this deep layer, no light reaches, making it very dark. Only organisms that have special adaptations can survive here. Marine organisms inhabit these zones based on their ability to adapt to the available light. When sunlight reaches the ocean, some of it is reflected into the air. How much light enters the water depends on the angle of the sun's rays. More light enters when the sun is perpendicular, while less light enters when the sun is at an angle. The ocean's surface absorbs most of the infrared heat from sunlight within the top 10 centimeters. At 10 meters, 50% of visible light energy is absorbed, while only 1% reaches 100 meters in clear tropical waters. The diagram shows that warmer colors absorb more light, while cooler colors scatter. This difference in light penetration reflects the difference in depth 27 Photosynthesis in the aquatic environments : Many autotrophic organisms, like aquatic plants, algae, and phytoplankton, use photosynthesis to convert sunlight into energy for growth. This process relies on light and occurs in the upper layers of water bodies where light is available. Solar radiation and ecological Balance: Solar radiation is crucial for maintaining aquatic ecosystems by affecting photosynthesis, water temperature, and the distribution of marine organisms. The Effect of Solar Radiation on Ecological Balance in Aquatic Environments: 1. The role of solar radiation in the distribution of marine organisms: Marine organisms are distributed unevenly in the water based on their light and energy needs. Here’s a clearer breakdown: Distribution of Marine Organisms Photosynthetic Organisms: o Location: These organisms are abundant in the surface layers of the ocean where sunlight is plentiful. o Examples:. ▪ Coral Reefs: Habitat: Coral reefs thrive in warm, shallow waters near the equator. Light Availability: These areas receive abundant solar radiation throughout the year. Symbiotic Relationship: The sunlight stimulates the growth of symbiotic algae within coral tissues, providing essential nourishment to the coral. 28 2. The effect of solar radiation on water temperatures: Solar radiation has a direct impact on water temperatures, which significantly affects the distribution of marine organisms. Here’s how it works: Impact of Solar Radiation on Marine Life o Water Temperature: o Solar radiation warms the surface waters, especially in tropical regions. o Attraction of Marine Species: o Warm waters attract specific types of fish and marine animals that thrive in these temperatures and need them for survival and reproduction. o Examples of Marine Species: o Tropical Fish: Species like tuna and barracuda are commonly found in warm tropical waters. o Cold-Water Species: In contrast, species such as cod prefer the colder waters located farther from the equator. This relationship between solar radiation, water temperature, and marine organism distribution highlights the importance of environmental conditions in shaping marine ecosystems. 29 3. Changes in solar radiation intensity: Variations in solar radiation intensity, whether due to seasonal changes or climate changes, can disrupt ecological balance in marine environments. Here’s how these disruptions occur: Effects of Solar Radiation Variations o Seasonal Changes: o In polar regions, solar radiation is low or absent during the winter months. o This leads to a significant drop in photosynthesis rates, resulting in decreased food availability for marine organisms that depend on photosynthetic sources. o Impact on Marine Life: o The decline in food availability can cause a decrease in the populations of organisms reliant on photosynthesis, which in turn affects the entire food chain. o Climate Change: o Global warming increases water temperatures, which poses a serious threat to coral reefs. o The death of coral reefs disrupts the habitats and food sources for various marine organisms that depend on these ecosystems for survival. These variations in solar radiation and their consequences highlight the delicate balance of marine ecosystems and the importance of stable environmental conditions for sustaining marine life. 30 4. The effect of solar radiation on ocean currents: Solar radiation plays a significant role in the formation of ocean currents, which are essential for distributing heat and nutrients throughout the oceans. These currents have a profound impact on the distribution of marine life and food resources. Key Points: Influence on Marine Life: Ocean currents help determine where different marine species thrive, making certain areas rich in food resources. Example: Gulf Stream o The Gulf Stream carries warm waters from the equator to the North Atlantic. o This movement of warm water moderates the climate in regions like Western Europe. o It also enhances marine biodiversity in these areas. In summary, ocean currents, driven by solar radiation, are vital for the health of marine ecosystems and the climate of coastal regions. 1-7 The effect of water pressure on living organisms Deep ocean organisms face harsh environments and unique adaptations, including living under immense water pressure. These organisms utilize physiological adaptations to survive under this pressure. Fluids, including liquid and gaseous substances, are characterized by their ability to flow and resist compression, allowing them to maintain constant volume. Pressure at a point inside a liquid: A liquid's pressure at a point equals the weight of the column above it, causing an object to experience a perpendicular force on its surface. 31 The force exerted on an object due to its presence in a liquid is calculated using the relation and its unit is Newton(N). F = P × A. Were o P is the pressure at a point in N/m2 o A is the surface area in m2 exposed to the pressure The pressure of a liquid (Pliquid ) at a specific point inside the liquid, located at a depth h from its surface, can be calculated using the formula: Pliquid=ρ g h Were ρ is the density of the liquid in kg/m3 g is the acceleration due to gravity in m/s2 h is the depth of the liquid surface. If the surface of the liquid is subjected to atmospheric pressure (Pa), the total pressure acting on the point is given by: Ptotal=Pa+Pliquid=Pa+ρgh In this equation, Pa represents the atmospheric pressure at the liquid’s surface. 32 The Factors affecting the magnitude of the liquid pressure at a point inside it: Key Conclusions on Liquid Pressure: Pressure and Depth: The pressure (P) inside a liquid increase with the depth (h) below the liquid’s surface. Pressure and Density: Liquid pressure also increases with the density (ρ) of the liquid. Units of Pressure: o Pressure is measured in N/m2, which is equivalent to the Pascal. o In practical applications, a larger unit, the bar, is often used. o 1 bar = 105Pascal = 105N/m². Properties of liquid pressure: “إلى سائل يتكون منHomogeneous”يشير مصطلح مما،نفس الجسيمات أو المادة الممزوجة بشكل متسا ٍو The pressure at any point in a liquid is كما هو،يضمن االتساق والتجانس في المظهر والسلوك equal in all directions, and in a.الحال في خليط متجانس مثل السكر homogeneous, static liquid, pressure remains constant along the same horizontal plane. This principle explains why water levels across connected seas and oceans remain consistent at the same horizontal plane. 33 Examples Ex1 An aquarium base of an area of 1000 cm2 contains water of the weight 4000 N, what is the magnitude of water pressure acting on the bottom of the aquarium? Sol 𝐏𝐥𝐢𝐪𝐮𝐢𝐝 = 𝛒 𝐠 𝐡 =𝐅𝐠/𝐀=𝟒𝟎𝟎𝟎/𝟏𝟎𝟎𝟎 × 𝟏𝟎−𝟒 = 𝟒 × 𝟏𝟎𝟒 𝐍/𝐦𝟐 Ex2 Calculate the total pressure exerted on a swimmer at a depth of 10 m from the surface of a lake of water if you know that the water density is 1000 kg/m3, the acceleration due to gravity is 10 m/s2, and the atmospheric pressure at the surface of the lake is 1.013 × 105 N/m2 Sol 𝐏 = 𝐏𝐚 + 𝐏liquid= 𝐏𝐚 + 𝛒 𝐠 𝐡 = 𝟏. 𝟎𝟏𝟑 × 𝟏𝟎𝟓 + (𝟏𝟎𝟎𝟎 × 𝟏𝟎 × 𝟏𝟎) = 𝟐. 𝟎𝟏𝟑 × 𝟏𝟎𝟓𝐍/𝐦𝟐 Ex3 A dam holds back water in a reservoir. The reservoir is 50 meters deep. What is the total pressure acting on the dam at the bottom of the reservoir? (Assume the water density is 1000 kg/m³ and atmospheric pressure is 1.013 × 10⁵ N/m²) Sol P = Pa + ρgh = 1.013 × 10⁵ + (1000 × 10 × 50) = 6.013 × 10⁵ N/m² 34 Ex4 A submarine is submerged to a depth of 200 meters in the ocean. What is the total pressure acting on the hull of the submarine? (Assume the water density is 1025 kg/m³ and atmospheric pressure is 1.013 × 10⁵ N/m²) Sol P = Pa + ρgh = 1.013 × 10⁵ + (1025 × 10 × 200) = 21.513 × 10⁵ N/m² Water pressure Water pressure is the pressure exerted by water on an object under its surface, increasing with depth due to the weight of water above it. Water pressure increases by approximately 1 atm for every 10 meters below the surface, and the pressure is equal to atmospheric pressure (1 atm = 1.013×105 N/m2). ex At 100m depth, water pressure is 10 times greater than atmospheric pressure, yet many creatures can adapt to this intense pressure. 35 The effects of pressure on the biological adaptations of creatures: 1. The swim bladder (air bladder) a. The surface water organisms: Organisms near the water surface have weaker physical structures due to low water pressure compared to those in deeper waters. b. Organisms in the intermediate depths: Organisms at depths 200-1000m are specialized to handle increased pressure, such as fish with gas-filled swim bladders for buoyancy and balance, or salmon for migration. c. Organisms at the great depths: At depths over 2000 meters, water pressure is intense, requiring organisms with compact body structures, proteins, and internal fluids to withstand it. Also, some of these organisms do not have to swim. Some organisms lack swim bladders or rely on liquid bladders and large, oil-rich livers to increase buoyancy and control depth, such as rays or rays that increase body density. 2. Bony and cartilaginous skeletons: a. Bony fish or Osteichthyes, like tilapia and mullet, have a bone- based skeleton that provides strong support and stabilizes their bodies under pressures like water movement or pressure. b. Cartilaginous fish or Chondrichthyes, including sharks and rays, have a cartilaginous skeleton instead of a bony one, due to their more flexible and lighter cartilage, which sets them apart from bony fish. 36 3. Cellular membranes a. Deep-water organisms' cellular membranes contain lipoproteins that enhance membrane elasticity, prevent collapse, and minimize pressure impact, ensuring cell damage and vital function continuation. 37 1 – 8 The role of solutions and concentrations in the movement of water and the distribution of living organisms. The distribution of organisms in oceans and lakes varies due to the presence of dissolved or suspended substances in water bodies, affecting its density and currents. 1. Aqueous Solutions Solution: is a homogeneous mixture composed of a solvent and a solute. In an aqueous solution, water typically acts as the solvent, while the solute can be a chemical substance like salts or other compounds. Concentration: This refers to the amount of solute dissolved in a specific volume of solvent. 2. Effect of Concentration on Water Density As the concentration of dissolved substances in water increases, the density of the water also rises. These changes in density can cause water movements, such as vertical currents, which can transport living organisms to different depths or bring them to the surface. 38 3. Colligative Properties of Water Colligative properties are characteristics of a solution that depend on the number of solute particles, not the type of solute. These properties include vapor pressure, boiling point, freezing point, and osmotic pressure. 1. the vapor pressure of the liquid: When a liquid and its vapor are in dynamic equilibrium, the vapor formed from evaporation exerts pressure on the surface of the liquid, called vapor pressure. Key points: In pure water: o Water molecules can break free and become vapor. o Water molecules attract each other through hydrogen bonds due to their polarity. In solutions: o Water molecules are also attracted to solute molecules. o The attraction between water and solute molecules is stronger than the attraction between water molecules themselves. o As a result, fewer water molecules evaporate, reducing the vapor pressure. Decrease in vapor pressure: o Vapor pressure reduction is directly proportional to the number of solute molecules or ions in the solution. 39 2. Boiling point: A liquid boils when its vapor pressure equals the atmospheric pressure at its surface. Key points: Boiling point of a pure liquid: o Constant under normal atmospheric pressure. o Used as an indicator of purity. o Varies with changes in air pressure: higher air pressure = higher boiling point. Boiling point of a solution: o Higher than that of pure water due to the stronger bonding forces between solute and solvent molecules. o Requires more energy to vaporize. o The increase in boiling point is directly proportional to the number of dissolved molecules or ions. 3. Freezing point: The freezing point of the solution is always lower than the freezing point of pure water because the attraction forces between water molecules and solute molecules hinder the freezing process and Life application: Salt is sprinkled on roads in cold areas after rainfall so that the rainwater turns into a salt solution, and its freezing point is lower than the freezing point of water. Thus, the amount of ice formed on the road’s decreases, which reduces the chances of accidents on the road. The distribution of organisms in aquatic environments is influenced by several factors: 40 1. Water type (Fresh vs Saltwater): o Organisms are distributed based on water type (e.g., freshwater fish can't survive in saltwater). 2. Osmotic adaptations: o Marine organisms adapt to high salt concentrations. o Freshwater organisms prevent absorbing excess water. 3. Nutrient and pollutant concentration: o Resource-rich environments support more diversity. o Polluted areas reduce diversity. 4. Seasonal changes: o Seasonal shifts, like dry or flood seasons, affect water abundance and organism movement. 5. Water currents: o Currents distribute oxygen and nutrients, impacting feeding and gathering areas of organisms. 41 1-9 Environmental balance and role of human in preserving the sustaining Human activities, such as overhunting and pollution, significantly impact the aquatic ecosystem. This study explores ecological balance, changes in this balance, and strategies to protect and sustain marine ecosystems. Importance of ecological balance in aquatic systems: Ecological balance is a dynamic stability achieved through organism interaction, ensuring life continuity, nutrient balance, diversity, and energy flow through food webs. 1. Nutrient balance: In aquatic systems like lakes and rivers, nutrient balance is crucial. Key points: a) Essential nutrients: o Nitrogen and phosphorus are vital for the growth of plants and algae, which form the base of the food chain. b) Excessive nutrients: o Overabundance, often from fertilizer pollution, can cause abnormal algal blooms, disrupting the ecosystem. 2. Balance between organisms: In aquatic systems, maintaining a balance between organisms is essential. Key points: a) Species interactions: o Organisms interact in various ways, such as prey and predators. b) Role of predatory fish: o Predatory fish help control the populations of prey fish and other organisms. c) Consequences of imbalance: o If predatory fish numbers decline (e.g., due to overfishing), small fish populations may increase excessively. o This can lead to overconsumption of food resources and disrupt the entire ecosystem. 42 3. Energy flow through the food web: In an aquatic ecosystem, energy flow through the food web is vital for maintaining balance. Key points: 1. Producers: o Energy begins with producers like algae and photosynthetic plants. 2. Consumers: o Energy then flows to consumers, including herbivorous and predatory fish. 3. Regulation of populations: o This energy flow regulates the numbers of organisms at each food chain level. 4. Example of imbalance: o If predatory fish consume small fish (which feed on zooplankton) excessively, it can lead to an increase in zooplankton populations. o This shift can negatively impact algae growth, disrupting the overall balance of the ecosystem. Example of the ecological balance in aquatic systems: Coral reefs serve as habitats for marine organisms, with predatory fish controlling small organisms like sea urchins to maintain balance and prevent unnatural population growth. The effect of human activities on the aquatic life: Several factors can negatively impact aquatic ecosystems: 1. Pollution: o Chemicals like pesticides and heavy metals degrade water quality. o These pollutants can harm the health of living organisms. 2. Overfishing: o Leads to a decline in certain species. o Disrupts the ecological balance of the ecosystem. 43 3. Environmental destruction: o Destruction of natural habitats (e.g., coral reefs and wetlands) results in a loss of biodiversity. The role of humans in maintaining the ecological balance: Humans significantly influence environmental changes, requiring them to maintain ecological balance and implement measures to mitigate negative effects. Here are some key strategies for promoting environmental balance: 1. Preserving natural resources: o Handle resources like water, forests, soil, and wildlife with caution. o Use resources sustainably and avoid pollution and overconsumption. 2. Environmental awareness and education: o Learn about the impact of actions on the environment. o Share knowledge through campaigns, workshops, and school programs. 3. Sustainable development: o Adopt development models that meet current needs without compromising future generations. o Develop and utilize clean technologies, promote sustainable agriculture, and enhance sustainability in industrial and urban sectors. 4. Participation in environmental policies: o Engage in environmental decision-making and policy development. o Participate in public dialogues, join environmental organizations, and advocate for strong governmental action. 5. Switching to eco-friendly practices: o Take small steps daily, such as reducing water and energy use, sorting waste, and using public transportation or bicycles. 44 Chapter Two : Atmosphere 2-1Atmosphere, its layers and components What Happens If a Planet Has No Atmosphere? Mercury, the smallest planet in the solar system, lacks an atmosphere. Without a gas envelope, its surface absorbs all the solar radiation, causing the temperature to rise significantly. When the sun sets, the radiation quickly escapes into space, and the planet cools rapidly since there's no atmosphere to retain heat. In contrast, Earth's atmosphere is a protective layer of gases that shields the planet from harmful radiation and objects from space. It also regulates surface temperatures and contains gases essential for life. Earth's gravity keeps the atmosphere intact. In this chapter, we will explore the composition and components of Earth's atmosphere and their role in sustaining life. The atmosphere is a mixture of several gases, with the most important being: Nitrogen (N₂): Making up about 78% of the atmosphere, nitrogen is largely inert and does not easily react with other gases or elements. It requires special conditions, like lightning or extremely high temperatures, to form oxides, which are present in very small amounts in the air. Oxygen (O₂): Comprising about 21% of the atmosphere, oxygen is essential for the respiration of all living organisms. It is a chemically active gas, playing a crucial role in combustion, respiration, and numerous natural and industrial chemical reactions. Argon (Ar) is an inert gas that constitutes approximately 0.93% of the atmosphere's volume. Carbon dioxide, constituting 0.04% of the atmosphere's volume, is crucial for plant photosynthesis. Water vapor (H2O) in the near atmosphere plays a crucial role in weather and climate phenomena due to its varying percentage in different locations. Ozone gas, located 10-55 km from Earth's surface, absorbs short- wave ultraviolet radiation, protecting Earth's surface from harmful effects, while the Earth's surface is toxic and harmful. 45 Layers of the atmosphere: 1. Troposphere The troposphere is the Earth's closest layer, with thickness of 18 km at the equator and 8 km at the poles. Its thickness is due to hot convection currents. The air temperature decreases with height in that layer, reduced by one degree Celsius for every 176 m with altitude due to atmospheric pressure decrease, causing air expansion and weather phenomena. 2. Stratosphere: The stratosphere layer, up to 50 km above sea level, contains the ozone layer. Temperature remains constant until 20 km, then rises as altitude increases due to ozone gas presence. Horizontal air movement favors this layer. 46 3. Mesosphere: The Earth's atmosphere is protected by a 30 km thick layer with the lowest temperature (-90o C), where most meteors burn up, preventing harm. 4. Ionosphere: The 640 km-high electrically charged layer, formed by solar radiation ionization of atmospheric atoms, is utilized in long-distance radio communications due to its ability to reflect radio waves. The effect of atmospheric pressure on wind movement: Atmospheric pressure is the pressure exerted by the weight of the air column from a specific point to the end of the atmosphere, affecting the surrounding area. Atmospheric pressure varies across different points in the atmosphere due to the height of the air column above the point. The difference in atmospheric pressure between two areas in the same horizontal plane causes air to move from high to low pressure areas. Weather maps use isobars, lines connecting points with equal atmospheric pressure, with low and high symbols. The millibar is the standard unit for barometric pressure on meteorological maps. 47 Atmospheric Pressure Overview Pressure exerted by air column weight from a point to the end of the atmosphere. Variation across different points due to air column height. Difference in pressure causes air to move from high to low pressure areas. Weather maps use isobars, lines connecting equal atmospheric pressure points. Millibar is the standard unit for barometric pressure on meteorological maps. Mercury barometer measures atmospheric pressure by comparing two mercury levels with a vertical height difference of 760 mm. The standard (normal) atmospheric pressure at sea level is 101300 N/m2, equivalent to 1013 millibar or 760 mm.Hg. The barometer can be used to determine the height of mountains, such as determining the standard atmospheric pressure. 48 2.2 Physical Factors in the Atmosphere This lesson explores the dynamic nature of the atmosphere, highlighting how physical factors like temperature, pressure, humidity, solar radiation, and wind speed influence weather, climate, and the distribution of organisms in different climate zones, providing insights into the underlying causes of weather changes. The physical factors and their effect on the atmosphere : First: Heat Heat is a crucial climatic factor that impacts atmospheric pressure, wind, humidity, condensation, and precipitation. The sun is the primary source of heat and light on Earth, which heats the Earth's land and water surface and transfers it to the surrounding atmosphere, causing temperature to rise. The atmosphere closest to the Earth's surface has higher temperatures. Sun rays heat different areas at different rates, with vertical or nearly vertical areas receiving more heat energy per unit area. - Measuring air temperature Meteorological organizations measure air temperature and compare it with other regions and previous years in the same climatic season. 49 These organizations use one of the following scales: 1- Celsius scale (to C), which is the scale used in Egypt, for example. 2- Fahrenheit scale (to F), which is the scale used in the USA for example. 3- Kelvin scale (TK), the absolute temperature scale used in scientific fields. The relationship between absolute temperature scale TK and Celsius scale 9 𝐭𝐜 is 𝐓𝐊 = 𝐭𝐜 + 𝟐𝟕𝟑, while the Fahrenheit scale tF is 𝐭𝐅 = ( × 𝐭𝐜) + 𝟑𝟐. 5 Mechanisms of heat transfer. Heat is generally transferred in three ways : Conduction transfers heat from higher temperature regions to lower temperature regions in solid objects, with metals having good thermal conductivity and wood having low conductivity. Convection is a process where heat is transferred through fluids, with higher-temperature parts becoming less dense and lower-temperature parts rising. This process is similar to thermal flight, where birds use a technique to stay in the air for extended periods without constantly flapping their wings, saving energy and maintaining their altitude. This technique is not only visually appealing but also conserves energy. 50 Radiation is the transfer of heat through electromagnetic radiation, which can propagate in all directions, without the need for a material medium, in vacuum or through gases. Second - Atmospheric Pressure: Atmospheric pressure impacts weather and climate, with low-pressure areas experiencing windy and rainy weather, and high-pressure areas experiencing stable, non-rainy weather. Atmospheric pressure differences cause wind to blow, with warm tropical air rising at the equator, creating a low-pressure area. The cooler, denser air above Earth's surface moves towards the equator to replace the hot air. The general direction of atmospheric pressure is generally from high to low pressure areas. Polar winds, dry and cold, originate from high atmospheric pressure around the north and south poles and low atmospheric pressure in subpolar regions. Atmospheric pressure impacts oxygen availability for breathing, with low pressure areas like high mountains requiring adaptations like increased red blood cell production. Mountain climbers may experience nose burst due to the increasing pressure difference between their internal blood pressure and the low atmospheric pressure outside. 51 Third - Humidity: Humidity, the amount of water vapor in the air, influences cloud formation and precipitation in tropical regions. It depends on temperature and atmospheric pressure, with saturation occurring when air reaches maximum water vapor content. The effect of humidity on living organisms: Some biological processes in living organisms are affected by the percentage of humidity in atmospheric air. As the relative humidity of the air surrounding the plant increases, the rate of transpiration decreases, which reduces the rate of lifting water and salts from the root to the leaves, and in animals, the rate of evaporation of sweat decreases, which reduces the efficiency of lowering their body 52 Fourth: Wind Speed: Wind, the movement of air from high to low atmospheric pressure, impacts heat and moisture distribution, affecting climate across regions, with strong winds causing significant weather changes. The effect of climate factors on living organisms: Climate significantly impacts organisms' distribution, growth, behavior, and evolution over time, with remarkable adaptation abilities demonstrated by these organisms to extreme environmental changes. 53 1.Adaptation to Freezing: The Wood Frog The wood frog lives in cold northern regions like Alaska and Canada, where temperatures drop below freezing. In winter, the wood frog's body partially freezes—its heart stops beating, and it stops breathing. Surprisingly, the frog does not die in this state but instead enters a deep hibernation until spring arrives and the snow melts, allowing it to thaw and resume normal function. The wood frog produces large amounts of glucose in its vital organs (heart, liver, brain) before freezing. The glucose acts as an antifreeze, preventing the formation of ice crystals in the cells and protecting them from damage. When temperatures rise in the spring, the ice melts, the heart starts beating again, and the frog’s bodily functions resume without harm. Antarctic Icefish: The Antarctic icefish, a rare species, survives in freezing waters by secreting antifreeze proteins in its blood, which prevent ice crystal formation. It also absorbs oxygen directly from the oxygen-rich waters of the extremely cold Antarctic, despite the harsh conditions. 54 Adaptation to High Temperatures: Desert Lizards: Desert lizards thrive in hot environments, where temperatures can reach dangerously high levels that can be lethal to other organisms. Desert lizards have developed distinctive adaptations that enable them to survive in harsh environments. The adaptations involve behavioral strategies like seeking shade or burrowing during hot hours, as well as physiological features like efficient water retention and temperature tolerance. The Australian desert-dwelling thorny devil lizard utilizes small channels on its skin to collect moisture from the air or sand. The channels direct water towards the lizard's mouth, ensuring its hydration in a dry environment. 55 2-3: Chemical Reactions in the Atmosphere The atmosphere, a shield for Earth, undergoes complex chemical reactions affecting air quality, climate, and public health, affecting ozone formation and air pollution. Chemical reactions in the atmosphere : 1. Ozone Formation: The ozone molecule (O₃) consists of three oxygen atoms. Ozone is formed in the stratosphere of the atmosphere through the action of ultraviolet (UV) radiation from the sun on oxygen molecules (O₂) as follows: - Photo dissociation occurs when UV radiation with a wavelength below 240 nm breaks the covalent bond in an oxygen molecule, resulting in the formation of two individual oxygen atoms. - Ozone Formation: An individual oxygen atom then reacts with an oxygen molecule (O₂) to form an ozone molecule (O₃). 56 The importance of ozone: Ozone acts as a shield that protects the Earth from harmful ultraviolet radiation. Without this layer, life on Earth would be severely damaged by these rays. The negative impact of ozone in the troposphere: 1- Air pollution: can be caused by natural sources like volcanoes and wildfires, or human activities like factory smoke and vehicle emissions. 2- Health issues: Ozone can cause various health issues including irritation of the eyes, nose, throat, breathing difficulties, asthma, and lung damage. 3- Environmental effects: Ozone can harm plants and agricultural crops, affecting growth and quality, and potentially causing corrosion of materials like plastics and rubber. 4- Greenhouse gas effect: Ozone, a greenhouse gas, contributes to the greenhouse effect, causing climate changes like rising temperatures and altered weather patterns in the troposphere. 2. Air Pollution Air pollution can originate from natural sources, such as volcanoes and wildfires, or from human activities, such as factory smoke and vehicle emissions. Air pollution, including CO₂ and other greenhouse gases, contributes to global warming, causing polar ice melting and rising sea levels. 57 Air Pollution and Human Health 1. Respiratory Diseases: Air pollution is associated with respiratory conditions like asthma, bronchitis, and allergies, as well as cardiovascular diseases such as heart disease and stroke. 2. Child Development: Exposure to polluted air can hinder brain development and overall growth in children. 3. Cancer Risk: Pollutants like benzene and arsenic are linked to a higher risk of certain cancers. Air Pollution and Ecosystems Air pollution can result in biodiversity loss in ecosystems. 1. Effect on Plants: Tropospheric ozone damages plant leaves, reducing their ability to perform photosynthesis, which hinders plant growth and productivity. 2. Effect on Animals: Birds and insects are affected by air pollution, impacting their behavior and reproduction. For example, declining bee populations due to air pollution reduce plant pollination rates. Strategies to Reduce Pollution 1. Public Transportation: Encouraging the use of public transport helps lower vehicle emissions. 2. Improving Energy Efficiency: Implementing energy-efficient technologies in homes and industries, such as using LED bulbs and high-efficiency appliances. 3. Increasing Green Spaces: Planting trees and establishing public gardens to improve air quality. 58 2-4 Atmospheric changes and their impacts Global warming and air pollution are contributing to rising summer temperatures, with some scientists predicting living organisms may need protective bubbles to shield themselves from pollution and radiation. This lesson will focus on applying our understanding of the atmosphere to assess its impacts and develop sustainable solutions to address environmental challenges. Changes in the atmosphere and their effects on everyday life: Understanding the atmosphere highlights the need to protect it. Changes in its composition weaken its ability to regulate Earth's surface temperature and shield the planet from harmful solar radiation. 1. Climate Change and Its Effects on Ecosystems: Governments hold global climate conferences to discuss the impacts of climate change and strategies to minimize and prepare for future changes. These discussions address key environmental issues which is : Global warming, caused by the burning of fossil fuels, is a continuous increase in air temperature, causing climate change, melting polar ice, and rising sea levels. Greenhouse gases that cause global warming include carbon dioxide, methane, nitrous oxide, chlorofluorocarbons, and water vapor. The increase in greenhouse gases in the atmosphere, similar to a greenhouse, blocks the passage of short-wavelength solar radiation, causing a gradual rise in Earth's surface temperature. 59 Negative effects of global warming : Global warming leads to melting ice, causing rivers and icebergs to separate, posing a risk of coastal flooding. This results in the extinction of polar creatures, a decrease in biodiversity, and severe climate changes like hurricanes, floods, and droughts. Solutions to air pollution and climate change: First: expand the use of renewable energy: Changing to clean energy sources such as solar, wind, and hydropower can reduce greenhouse gas emissions. Second: Planting:The Egyptian state is keen on green spaces in new cities to increase photosynthesis, a crucial process in absorbing carbon dioxide gas, a major contributor to global warming, making planting a vital strategy. How does the Earth hold on to atmospheric gases? 1. Gas molecules move at high speeds, influenced by their mass and temperature, with less massive molecules moving faster at higher temperatures. 2. Escape velocity Ve is a constant amount for every planet, requiring an object to gain a certain speed to escape its gravitational field. 3. The escape velocity from Earth's gravity is about 11.2 km/s. 4. The presence of a planet's surface gas depends on the relationship between the effective velocity of gas particles (vrms) and the escape velocity (ve). 5. When the effective velocity of gas molecules (vrms < ve) is less than the escape velocity, the planet's gravity prevents the gas from escaping into space. 6. Gas molecules can escape a planet's gravity if their effective velocity is equal to or greater than its escape velocity, making the gas rare or non-existent on the planet's surface. 60 The effect of atmospheric change on living organisms: The atmosphere's changes, including temperature, atmospheric pressure, humidity, and air pollution, significantly impact the lives of organisms. Understanding how these changes affect daily life enables us to take effective steps to adapt to these changes. Temperature changes: Humidity changes affect plant growth, while air pollution negatively impacts human health and wildlife, potentially leading to species extinction. 61 Chapter Three : Soil 3-1: Soil composition and its importance in the ecosystem Soil study is a field in environmental and agricultural sciences that examines soil properties, composition, and its impact on plants, animals, and the environment. It is crucial for nutrient supply and food security, and proper management is essential to prevent depletion. Soil Soil is a complex system that serves as the upper, loose layer covering the Earth's crust. Key points about soil include: Composition: Soil is formed from the weathering and disintegration of various types of rocks, including igneous, sedimentary, and metamorphic. Formation Process: The formation of soil results from the effects of different surface physical factors and their interactions with various environmental elements. Physical weathering is the fragmentation of rocks due to temperature changes, repeated freezing and thawing of water in rock cracks, mechanical processes like rock rubs during transportation, wind and water currents, and gravity. Chemical weathering alters the chemical composition of minerals by reacting with air or other chemicals, leading to the decomposition of rocks in the presence of water. Biological weathering involves the movement of plant roots, water, and air within rocks, causing them to crack and break apart. 62 Soil constituents: Soil is made up of several key components : Minerals: Soil's largest component is minerals, primarily silicates. Primary minerals are irregular, coarse, and irregularly shaped, while secondary minerals are stable, formed from chemical and biological weathering processes of primary minerals. Organic matter: 1. Humus, consisting of living and dead organisms like insects, earthworms, fungi, bacteria, and other living and dead plants and animals, mixes with the soil. 2. Organic matter significantly impacts the chemical, physical, and biological properties of the soil. 3. Organic matter, including humus, significantly impacts soil's chemical, physical, and biological properties, with fertilizers and plant crop residues being the primary sources. 4. Organic materials encompass not only organic materials but also living components like insects, earthworms, fungi, parasites, and other organisms. Water: Water, found in soil pores, is crucial for transporting nutrients and facilitating biological and chemical decomposition. Soil's water retention depends on soil grain size and temperature, with clay soils being better at retaining water than sandy soils. 63 Gases: Atmospheric gases exchange within soil pores, including oxygen for plant root respiration and soil microorganisms, and carbon dioxide and nitrogen for nitrogen-fixing bacteria, crucial for plant growth. Soil profile: Soil profile is a vertical section in soil that reveals major horizons or zones with varying thickness based on soil type, bed rock formation, organism and climate influence, and weathering exposure. Most different types of soil consist of three main horizon: -The soil surface or horizon (A):Plant roots, earthworms, insects, and microorganisms are active in the humus, rich in nutrients, organic matter, and biological activity, making Horizon A darker in color due to organic matter. -The subsoil surface or horizon (B): The biological activity of horizon (B) soil, a clay-rich soil, is generally less fertile, retains more moisture, is lighter in color, and has a coarser texture. -The parent rock or horizon (C): This layer is composed of cohesive or loosened rocky material, from which soils of horizon (A) and (B) were formed, and plant roots do not penetrate it. 64 Soil types and their physical properties : Soil properties vary based on composition and environment, including clay, loamy, silt, sandy, humid, calcareous, red, and others. The main types of soils include: 1. Clay soil : Clay soils are dense, poorly ventilated, and have high water retention capacity, leading to poor drainage due to difficulty in water seepage. They retain moisture for extended periods, and their expansion and shrinking, causing clay cracks, can result from their small spaces. 2. Sandy soil : Sandy soils are large, coarse deposits with large pores, making them well-ventilated but low water retaining. They quickly dry, making them unsuitable for agriculture without regular irrigation, and are often exposed to deduction factors. 3. Alluvial soils (silt soils) : Alluvial soils are a blend of clay, sand, and silt, moderately aerated, and have high water retention capacity, but may weaken when waterlogged. The soil's average drainage retains water but may become saturated in wet conditions, but its high fertility makes it ideal for cultivation. 65 The role of soil in the ecosystem : Soil plays a vital and essential role in the ecosystem, contributing to many environmental processes that support life on Earth. Among the most important roles that soil plays in the ecosystem: Supporting plant growth : Nutrient Source: Provides essential nutrients like nitrogen, phosphorus, and potassium necessary for plant growth. Root Stabilization: Acts as a support structure for roots, preventing plants from drifting or falling over. Water Retention: Retains moisture and makes it available to plants as needed for growth. Regulating the water cycle: Rainwater Absorption: Absorbs and stores rainwater, helping to provide water to plants during dry periods. Natural Filtration: Acts as a natural filter, removing impurities and pollutants from water as it seeps through soil layers before reaching groundwater. Nutrient cycling: Soil is home to microorganisms like bacteria and fungi that convert dead organic matter into nutrients that plants can utilize. Supporting biodiversity and ecological balance: Habitat for Organisms: Provides a habitat for a wide range of organisms (worms, insects, bacteria, fungi) that decompose organic matter, fix nitrogen, and aerate the soil. Support for Plant Biodiversity: Supports various plant species by providing a suitable environment, which in turn supports wildlife. 66 Climate regulation: Climate Change Mitigation: Healthy soils sequester carbon (absorb carbon dioxide) and help reduce greenhouse gases in the atmosphere. Greenhouse Gas Emission Reduction: Reclaiming degraded soils and adopting soil conservation practices can help lower greenhouse gas emissions. Temperature Regulation: Absorbs and stores heat during the day and slowly releases it at night, helping to moderate rapid temperature changes. Maintaining the structure of the Earth and preventing erosion: Plants that grow in the soil help stabilize it and prevent it from being eroded by wind or water. Healthy soil planted with plants helps prevent desertification and land degradation. 67 Activity 1: Analyzing Soil Samples Objectives To collect and analyze soil samples from various environments. To assess and compare the soil samples based on their physical characteristics and potential impact on plant growth. Procedure 1. Sample Collection: o Collect soil samples from three different locations: ▪ Garden: Expect a rich mixture of organic matter and nutrients. ▪ Farmland: Anticipate variations due to fertilizers and cultivation practices. ▪ Roadside: Expect soil potentially impacted by pollution and less organic content. 2. Sample Analysis: 68 o Analyze each soil sample based on the following parameters: A. Texture Observation: o Procedure: ▪ Touch each soil sample. ▪ Evaluate and categorize the texture: ▪ Is it soft, coarse, or sticky? ▪ Notes: Document the tactile observations for each sample. B. Water Retention Test: o Procedure: ▪ Place a small amount of each soil sample in a strainer or over a cotton cloth in a funnel. ▪ Slowly pour a measured amount of water onto the soil. ▪ Observation: ▪ Note how quickly the water seeps through the soil. ▪ Determine if it soaks quickly (indicating sandy soil) or if it holds water for a long time (indicating clay soil). ▪ Notes: Record the findings for each sample. C. Drainage Test: o Procedure: ▪ Place equal amounts of each soil sample in clear cups with identical holes at the bottom. ▪ Add an equal amount of water to each cup. ▪ Observation: 69 ▪ Measure how quickly the water drains from each soil type. ▪ Notes: Document the drainage rate for each sample. 3. Comparison of Samples: o Analyze the data collected from the texture observation, water retention test, and drainage test. o Discuss the potential impact of each soil type on plant growth: ▪ Texture: Affects root penetration and water retention. ▪ Water Retention: Important for understanding how well each soil supports plant hydration. ▪ Drainage: Influences aeration and the risk of root rot in plants. Conclusion Summarize the findings from the analysis, highlighting the differences between the soil samples. Discuss how the physical properties observed affect plant growth, such as nutrient availability, water access, and root development. Make recommendations for soil management based on the observed characteristics. Activity 2: Influence of soil on plant growth Sow seeds of the same type of plant in different types of soil (sand, clay, loam). Observe the growth of the plants over a period of two weeks, and record observations about plant height, leaf color, and germination percentage. Conclude which soil type was more favorable for plant growth and why. 70 3 – 2 :The effect of human practices on soil Soil is crucial for ecosystem support and nutrient cycle, but human activities like agriculture and industry can degrade its quality. This lesson explores how these activities affect soil and strategies to protect this vital resource. effect of agricultural activities on soil quality: 1.Soil compaction: Heavy agricultural machinery, including tractors and large equipment, can excessively compact the soil, leading to several negative consequences: 1. Reduced Water and Air Absorption: o Soil compaction significantly decreases the soil's capacity to absorb water and air, which are vital for plant health. 2. Formation of Hard Layers: o Compaction creates hard, petrified layers beneath the soil surface, obstructing plant root growth. 3. Challenges for Plant Growth: o Plants in compacted soil struggle to access water and nutrients, resulting in unhealthy growth. o This is particularly evident in wheat fields, where compacted soil leads to shorter and weaker plants. 4. Impact on Agricultural Yields: o Overall, soil compaction contributes to poor crop yields, affecting agricultural productivity. Addressing soil compaction is essential for promoting healthy plant growth and ensuring sustainable agricultural practices. 71 2 - Salination : Soil salinity refers to the increase of salt levels in the soil. It occurs when excess salts accumulate on the soil surface, often visible to the naked eye. Here’s how soil salinity develops: 1. Transportation of Salts: o Salts present in groundwater are transported to the soil surface through capillary action. o When the water evaporates, it leaves behind the salts, leading to increased salinity. 2. Areas Affected: o This phenomenon is particularly prevalent in regions like Egypt's Nile Valley. 3. Mechanism of Accumulation: o Water contains dissolved salts that seep into the soil. o Plants absorb water and a small amount of dissolved mineral salts. o Over time, this process results in the accumulation of large amounts of salts in the soil. 4. Consequences of Increased Salinity: o The elevated salinity hinders the ability of plants to absorb water. o This leads to soil degradation and plant degeneration. Understanding these factors is crucial for managing soil health and ensuring sustainable agricultural practices. 72 3- Lack of nutrients in the soil : One of the biggest mistakes in agriculture is the practice of growing a single crop on the same soil year after year. While this method may yield some short-term economic benefits, these advantages are often temporary. The long-term consequences include: 1. Soil Exhaustion: o Continuous cultivation of the same crop depletes the soil, leading to exhaustion over time. 2. Nutrient Depletion: o This practice results in a lack of essential nutrients necessary for plant growth, ultimately affecting crop health and yields. To ensure sustainable agricultural practices and maintain soil health, diversifying crops is essential. The effect of industrial activities on soil quality Industrial activities contaminate the soil with chemicals and heavy metals, causing serious environmental Problems. 1.Soil contamination with heavy metals : Globally, the drainage of industrial waste has resulted in the accumulation of high levels of heavy metals, such as lead and mercury, in numerous industrial areas. This contamination poses serious risks: 1. Toxicity to Plants and Animals: o Heavy metals like lead and mercury can be highly toxic, adversely affecting both plant and animal health. 2. Consumption Risks: o When contaminated soil is consumed by plants, it can lead to toxic effects in animals and humans who eat these plants. 73 Addressing industrial waste drainage is crucial for protecting soil health and safeguarding ecosystems. 2 - Soil contamination with toxic chemicals : Toxic chemicals from industrial areas, particularly around oil refineries and petrochemical factories, can seep into the soil and lead to significant pollution. Key issues include: 1. Contamination of Agricultural Soil: o Areas surrounding oil refineries often experience contamination with hazardous substances, such as gasoline. 2. Health Risks: o Gasoline contains carcinogenic compounds, and soil contamination with these substances may increase the risk of cancer for individuals living in these areas. Addressing soil pollution from industrial chemicals is essential for protecting public health and ensuring the safety of agricultural practices. 3 - Soil contamination with nitrate compounds : n agricultural areas that heavily rely on manufactured nitrogen fertilizers to boost crop yields, such as some regions in the Egyptian Delta, excessive nitrate contamination of the soil is a significant concern. The key issues include: 1. Nitrate Contamination: o Excessive use of nitrogen fertilizers leads to increased nitrate levels in the soil, which hinders plants' ability to absorb other essential nutrients, resulting in poor growth. 74 2. Groundwater Pollution: o Nitrate compounds can seep into groundwater due to excessive irrigation or rainfall. This process makes the groundwater a serious pollutant. 3. Health Risks: o Contaminated groundwater can pose serious health issues, such as Blue Baby Syndrome, which affects infants who consume nitrate-contaminated water. This condition can cause a reduction in the oxygen-carrying capacity of blood, leading to health complications. Addressing nitrate contamination is crucial for ensuring soil health, protecting water quality, and safeguarding public health. 75 Methods of soil conservation: Strategies for Soil Preservation and Protection Against Degradation To preserve soil quality and protect it from degradation, consider adopting the following practices: 1. Sustainable Agricultural Practices: o Organic Farming: Rely on natural fertilizers and biopesticides. Convert agricultural waste and organic materials into organic fertilizer to enrich the soil without harmful chemicals. 2. Crop Rotation Techniques: o Implement a system of agricultural cycles by rotating crops and planting diverse vegetation. This helps prevent soil erosion and promotes a healthier ecosystem. 3. No-Till Farming: o Utilize the “no-till farming” technique to maintain soil structure and reduce erosion. This method helps retain moisture in the soil and improves long-term fertility. Adopting these practices can significantly contribute to soil health and sustainability, ensuring productive agricultural systems for the future. 76 3-3 The effect of Acid Rain on Soil Acid rain, caused by atmospheric reactions with water vapor, significantly impacts ecosystems, particularly soil and plants, by causing the formation of acids. First: Acid Rain and Its effect on Soil Acid rain is an environmental phenomenon that occurs when air pollutants, such as sulfur oxides (SOₓ) and nitrogen oxides (NOₓ), react with water vapor in the atmosphere to form acids, specifically sulfuric acid and nitric acid. Key points include: 1. Formation of Acid Rain: o These acids mix with rainwater, lowering its pH to below 5.6, making it more acidic. 77 2. Effects on Soil: o When acid rain falls on the soil, it interacts with various components, including carbonate salts and minerals. This reaction can lead to: ▪ Soil Erosion: The acidic rain can cause the soil to erode more easily, degrading its structure. ▪ Chemical Composition Changes: The introduction of acids alters the soil's chemical makeup, affecting nutrient availability and overall soil health. Understanding the effects of acid rain is crucial for implementing measures to protect soil and maintain its quality. Effects of Acid Rain on Soil: 1-Soil Degradation Due to Acid Rain Acid rain can have detrimental effects on soil health, leading to various forms of degradation: 1. Erosion of Essential Minerals: o Acid rain can erode essential minerals in the soil, such as calcium and magnesium, which are crucial for maintaining soil fertility. The loss of these minerals can significantly reduce the soil's ability to support healthy plant growth. 78 2. Release of Toxic Metals: o Acid rain stimulates the deposition of toxic metals, such as aluminum, in the soil. o Trees and plants that absorb these metals may suffer from poisoning, which hinders their growth and can ultimately lead to their death. Understanding these impacts is essential for developing strategies to mitigate the effects of acid rain and protect soil quality. 2-Effects of Acid Rain on Plants Acid rain can have several negative effects on plant health and agricultural productivity: 1. Toxic Effects: o Acid rain can erode and poison plant roots with toxic metals, adversely affecting their health. 2. Reduced Growth: o Lower nutrient levels in the soil due to acid rain can hinder plant growth and weaken overall plant health. 3. Decreased Crop Yield: o The deterioration of soil quality from acid rain can adversely affect crops, leading to lower agricultural productivity. 4. Crop Damage: o Acid rain can directly damage crops by impairing their ability to absorb essential nutrients. Additionally, acid rain disrupts the ecological balance. Changes in soil and plant health can negatively impact other plants and animals that rely on healthy soil, further compromising ecosystem stability. 79 Strategies for Reducing the Effects of Acid Rain on Plants and Soil To mitigate the impact of acid rain on plants and soil, the following strategies can be implemented: 1. Reducing Emissions: o Enhance fuel combustion technologies and incorporate purification devices to decrease sulfur and nitrogen oxide emissions from industrial processes and vehicles. 2. Using Alkaline Fertilizers: o Apply alkaline fertilizers, such as lime, to neutralize acids in the soil and restore pH balance, promoting healthier soil conditions. 3. Implementing Environmental Policies: o Enforce laws and regulations aimed at controlling pollutant emissions to protect air and soil quality. 4. Raising Awareness: o Promote environmental awareness regarding the effects of acid rain and the importance of preventive measures among communities and stakeholders. By adopting these strategies, we can help protect plants and soil from the harmful effects of acid rain and contribute to a healthier environment. 80 3-4 Soil measurements and strategies of its preservation: This lesson focuses on understanding soil properties like humidity, acidity, and mineral structure, and discussing strategies for preserving and improving soil quality for sustainability, ensuring the planet's life support. First: techniques of measuring the humidity, acidity, and mineral structure of the soil Understanding of soil properties requires using fine measuring techniques. 1.Soil humidity: Understanding Soil Humidity Soil humidity refers to the total amount of water present in the soil's fine pores or on its surface. Several environmental factors influence soil humidity, including: Rainfall: The amount of precipitation directly affects the water content in the soil. Soil Type: Different soil types have varying capacities to retain moisture. Plant Type: The presence of certain plants can influence water retention and consumption in the soil. Soil humidity is a crucial indicator for cultivation. If the water content in the soil increases or decreases beyond a certain level, it can negatively affect plant health. Climatic changes are primarily responsible for fluctuations in soil humidity throughout the year. These changes can impact water availability and influence agricultural practices. 81 Measuring humidity: The moisture percentage in soil can be measured using a Moisture Meter or by weighing before or after dryness. Factors effect on the soil humidity: 1.Salinity: As soil salinity increases, the percentage of water in the soil decreases. High salt concentrations can hinder water absorption by plants. 2.Particle Size: Larger soil particles lead to increased porosity (the percentage of pore space in the soil sample) and greater permeability (the ease of water movement between soil particles). However, this can result in a decreased water percentage in the soil. 3.Consistency of Particle Size: When soil particles vary significantly in size (such as a mixture of sand, clay, and silt), porosity decreases, permeability lowers, and the water percentage in the soil increases. 4.Temperature: As soil temperature rises, soil humidity tends to decrease. Higher temperatures can lead to increased evaporation of moisture from the soil surface. 5.Depth: As depth increases, soil humidity typically increases as well. Deeper soil layers often retain more moisture due to less exposure to evaporation. 82 Consequences of increased humidity of the soil: Increased humidity in the soil can lead to root rot and ultimately the death of plant roots. While plant roots require water for growth, they also need air for cellular respiration. Here’s how excessive soil humidity affects root health: 1. Reduced Air Availability: o As soil humidity increases, the amount of air that reaches the plant roots decreases. This lack of oxygen can lead to the death of root cells due to their inability to respire effectively. 2. Growth of Harmful Organisms: o Excess water creates a favorable environment for saprophytic bacteria and fungi. These organisms thrive in overly moist conditions and can grow on dead roots, contributing to their decay. Addressing issues of soil humidity is crucial for maintaining healthy root systems and overall plant health. Activity Aim: Measuring the percentage of humidity Tools: Pot containing a plant, Moisture Meter Steps: 1- be sure that the metal rod of the device is clean and completely dry, and its reading at zero. 2- Insert the metal rod in soil near the roots of the plant,and be sure that it is not in contact with the bottom of the pot. 3- Determine the reading directly. 4- Compare the reading with the suitable rate in irrigation of the 83 Activity Aim: Measuring the percentage of humidity of soil by using technique of weight. Tools: Pot containing a sample of soil, digital balance, Bunsen flame, heating dish. Steps: 1- By using the digital balance determine the mass of empty dish. 2- Put a sample of soil on the heating dish on the flame till the water evaporates from it completely. 3- By using the digital balance determine the mass of the dish and the soil together. 4- Mass of soil = Mass of dish and soil – mass of the empty dish 5- Irrigate the pot by amount of water. 6- By using the digital balance determine the mass of soil after adding water to it. Use the relation: Mass of wet soil − mass of dry soil Percentage of humidity = X 100 𝒎𝒂𝒔𝒔 𝒐𝒇 𝒅𝒓𝒚 𝒔𝒐𝒊𝒍 Measuring Soil Acidity (pH) The acidity of soil can be measured using a pH meter, which determines the soil's acidity or basicity (alkalinity). Key points include: 1. Importance of pH: 84 o Soil pH is a crucial indicator for selecting the appropriate type of soil for cultivating specific crops. Different crops have varying pH requirements for optimal growth. 2. Neutralizing Acidity: o When soil acidity increases, it can be neutralized by applying alkaline substances, such as lime. This helps restore a more suitable pH level for crop cultivation. Understanding soil pH is essential for effective agricultural practices and ensuring healthy plant growth. Aim: Measuring the acidity of soil. Tools: Digital pH meter. Steps: 1- Place the metal part in distilled water as in the figure, then dry it with a tissue to be sure it is clean and free from any previous residues that affect the reading of pH meter. 2- put the metal rod of the device in the soil that is needed to determine its pH without reaching the bottom. 3- Record the reading of pH device directly. Observation and explanation: pH 7 means neutral soil pH higher than 7 means alkaline soil pH less than 7 means acidic soil 85 Measuring the Percentage of Minerals in Soil Soil analysis can be conducted using chemical methods to determine the components of essential elements, such as Nitrogen, Phosphorus, and Potassium (NPK). Understanding the percentage of these essential elements in the soil plays a crucial role in plant cultivation: 1. Potassium (K): o Potassium is essential for the healthy growth of plants and is vital for flower formation. 2. Phosphorus (P): o Phosphorus helps strengthen plant roots, promoting stability and nutrient uptake. 3. Nitrogen (N): o Nitrogen is crucial for the greening of plant leaves, supporting overall growth and vigor. Regular soil analysis for NPK levels can help optimize fertilization practices and improve crop yields. Second: Strategies of Preservation the soil and enhancing its quality: Preserving Soil and Enhancing Its Quality through Sustainable Agricultural Practices To preserve soil and improve its quality, sustainable agricultural practices should be implemented. One effective practice is maintaining plant cover, which offers several benefits: 1. Prevention of Soil Erosion: 86 o Cultivating plants that cover the soil helps prevent erosion and soil dredging. The roots of these plants bind soil particles together, making the soil more resistant to the effects of wind and rain. 2. Preserving Soil Humidity: o Plant cover helps retain soil moisture, preventing excessive evaporation and maintaining a balanced water level. 3. Protection from Desertification: o Trees and other plants act as natural windbreaks, protecting the soil from being carried away by strong winds. This helps prevent desertification in vulnerable areas. By applying sustainable practices like plant cover, we can significant