Daily Variation of Urban Heat Island Effect (PDF)
Document Details
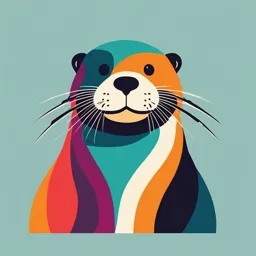
Uploaded by WinningAllegory
2017
Ali Soltania, Ehsan Sharifi
Tags
Summary
This research article investigates the daily variation of the urban heat island effect in Adelaide, examining its relationship with urban greenery. The study maps temperature profiles across the city and identifies peak heat variations. Improved understanding of UHI fluctuations assists in urban policy.
Full Transcript
Frontiers of Architectural Research (2017) 6, 529–538 Available online at www.sciencedirect.com Frontiers of Architectural Research www.keaipublishing.com/foar RESEARCH ARTICLE Daily variation of urban heat island effect and its correlations to urban greenery: A case study of Adelaide Ali Soltania,b...
Frontiers of Architectural Research (2017) 6, 529–538 Available online at www.sciencedirect.com Frontiers of Architectural Research www.keaipublishing.com/foar RESEARCH ARTICLE Daily variation of urban heat island effect and its correlations to urban greenery: A case study of Adelaide Ali Soltania,b,n, Ehsan Sharific a Shiraz University, Shiraz, Iran University of South Australia, Australia c University of Adelaide, Australia b Received 3 November 2016; received in revised form 6 July 2017; accepted 18 August 2017 KEYWORDS Abstract Urban heat island effect; Mobile traverse method; Heat stress; Urban greenery; Adelaide Urban structure and landscape cause an artificial temperature increase in cities, known as the urban heat island effect. The magnitude of such urban–rural temperature difference varies in daily and seasonal basis. Daily patterns of urban heat accumulation in Adelaide is under investigation. In this paper, East–West air temperature profile of Adelaide metropolitan area was mapped in 60 journeys alongside a straight cross route connecting Adelaide Hills to the West Beach under clear sky between 26 July and 15 August 2013. The most intense urban–rural temperature differences of 5.9 1C occurred during midnight in Adelaide. However, maximum urban heat variation occurred during the late afternoon when the near-surface urban heat fluctuates by 2 1C between the CBD East and Western Parklands. During summer heatwaves, the afternoon heat stress limits public life vibrancy in Adelaide. Increased urban greenery can facilitate resilience to heat by providing shadow and evaporative cooling. A better understanding of daily urban heat variations and the cooling effect of urban greenery assists urban policy making and public life management in the context of climate change. Crown Copyright & 2017 Production and hosting by Elsevier B.V. This is an open access article under the CC BY-NC-ND license (http://creativecommons.org/licenses/by-nc-nd/4.0/). 1. n Correspondence to: Art, Architecture & Design, University of South Australia, K3-14, City West Campus, Australia. E-mail address: [email protected] (A. Soltani). Peer review under responsibility of Southeast University. Introduction Australia is likely to experience 3.8 1C increase in its surface temperature by 2090 (CSIRO, 2014). Such regional warming will have a severe impact on local climate regimes, natural ecosystems and human life. In this context, heat stress can become up to 8 1C higher in urban settings compared to http://dx.doi.org/10.1016/j.foar.2017.08.001 2095-2635/ Crown Copyright & 2017 Production and hosting by Elsevier B.V. This is an open access article under the CC BY-NC-ND license (http://creativecommons.org/licenses/by-nc-nd/4.0/). 530 A. Soltani, E. Sharifi their rural counterparts (Gartland, 2008; Kolokotsa et al., 2009). Urban structure, hard surfaces and shortage of vegetation cover in cities are cited as the major contributors to the artificial temperature increase in cities, commonly known as the urban heat island (UHI) effect (Stone, 2012). Due to variations in the UHI effect and its lower magnitude during the day, very limited research is available on daily variations of urban heat in cities when the UHI effect threatens usability of outdoor public spaces (Nikolopoulou, 2011; Santamouris et al., 2015; Sharifi et al., 2016). In response to substantial excess heat in cities, people increasingly move into air-conditioned buildings to benefit from indoor thermal comfort. Meanwhile, resulted anthropogenic heat generated from indoor air-conditioning causes an everincreasing outdoor temperature. In this context, this paper analyses daily variations of urban heat in Adelaide metropolitan area. Results assist urban policy and place making in the context of climate change. 2. The urban heat island (UHI) effect In the early 19th century, Howard compared urban heat in London and reported that the mean annual temperature (20-years average) is 2.5 1C higher in London than its countryside. The peak air temperature variation of 3 1C was recorded during February (mid-winter) (Gartland, 2008). Similar macro-scale urban heat investigations contribute to the understanding of the UHI effect mechanism via comparing city centres and their rural surroundings (Bourbia and Boucheriba, 2010; Mirzaei and Haghighat, 2010). Gartland (2008, p. 2) enumerates five common characteristics for the UHI effect: UHIs are warmer than their rural vicinities. The urban–rural temperature difference is higher in calm and clear weather, at night, and in winter. The UHI effect occurs due to human-made modifications in urban surface covers. More urban development and less greenery correlate to the intensity of UHIs. UHIs create a dome of warmer air above cities. Heat islands are uneven in their spatial distribution and magnitude, especially during daytime (Oke, 2006a) and can vary based on the space configuration and urban features in smaller scales (Erell, 2008). Figure 1 illustrates that the magnitude of urban–rural temperature differences is usually reported to be higher at night time; Thus, (Runnalls and Oke, 2000; Arnfield, 2003). The urban–rural temperature difference starts to develop during the day under clear sky due to solar gain of urban surface materials (Ashie, 2008). Calm weather causes the warm air to be kept in the built environment for an extended time (Morris et al., 2001; Wong and Yu, 2008). 2.1. UHI contributing factors Oke (2006b, p. 184) highlights urban structure, surface cover, fabric and metabolism as the major contributing factors to the UHI effect. Figure 1 The UHI effect peaks at the clear and calm weather at winter nights. It also peaks earlier during winter Schematic representation based on Gartland (2008, p. 4). 2.1.1. Urban structure Buildings’ volume, orientation and the aspect ratio of the spaces between them (measured by the sky view factor) affect the exposure of urban surfaces to the solar radiation. Thus, urban structure affects shadow patterns and heat exchange in the built environment (Johansson, 2006; Lin et al., 2010; Krüger et al., 2011; Andreou, 2013). The complex heat exchange between buildings’ mass and adjacent air changes the intensity and patterns of airflow in urban canyons where wind patterns also affected by the canyon-like structure of streetscapes surrounding by tall buildings. 2.1.2. Urban surface cover Urban surface materials’ thermal characteristics (specific heat, mass, conductivity and diffusivity), color, texture and coverage alter heat exchange in urban settings. Distribution and ratio of land cover classes including paved, vegetated and bare land and surface water contribute to the heat exchange between the urban area and the adjacent air (Karatasou et al., 2006). Due to land cover differences and their respective roughness layers (with temperature differences) local air turbulence occurs in the urban settings which mix the hotter and cooler air and affects the UHI effect intensity. 2.1.3. Urban fabric The overall ratio of residential, commercial and industrial land use, roads and open spaces, parklands and wetlands affects the intensity and distribution of UHIs. Lack of sufficient greenery – as a common characteristic of urban areas – contributes to the accumulation of heat in the built environment thermal mass (Gartland, 2008; Wong and Jusuf, 2010; Coutts et al., 2012). Typology, distribution and intensity of urban greenery also affect local wind patterns. 2.1.4. Urban metabolism Urban life is associated with energy consumption, causing additional waste heat production in cities (Soltani et al., 2012). Such anthropogenic (human-made) heat is mainly related indoor air-conditioning and transportation (Arnfield, 2003; Ichinose et al., 2008; Rizwan et al., 2008; Samuels et al., 2010). Resulted excess heat can increase the need for even more intense indoor air-conditioning. Figure 2 illustrates the interplay between UHI contributing factors. Daily variation of urban heat island effect and its correlations to urban greenery 531 Figure 2 Urban structure, cover, fabric and metabolism contribute to the UHI effect in highly developed areas. (Modified based on: Sharifi and Lehmann (2014, p. 25)). 2.2. UHI metrics UHIs are commonly represented in three major scales, namely urban surface material scale (surface layer), building and public space scale (canopy layer) and city scale (boundary layer) (Arnfield, 2003; Oke, 2006b). The UHI effect is highly dependent on the thermal behaviour of surface materials and their exposure to solar radiation. Emitted heat from urban surfaces is mixed through convection and affects the air temperature above urban surfaces. The urban section limited between the surface cover, and the skyline of buildings is regarded as urban canopy layer. Geometry and orientation of open spaces, aspect ratio (height to width), sky view factor (fraction of sky visible from the ground up) (Svensson, 2004), land cover materials (asphalt, hard-landscapes, grass cover, water, tree, etc.) and wind flow affect heat accumulation in the canopy layer. Emitted heat from urban surface layer mixes via air turbulence in urban canopy layer and above. Resulted air mixture shapes a dome of warm air over the urban areas which is referred to as the urban boundary layer. The formation of warm air dome over built-up areas is because of the blending effect of local air above the canopy layer (Oke, 1987; Tapper, 1990; Thatcher and Hurley, 2012). The height of such a warm air dome can reach up to 1500 m above the urban surfaces at midnight while it is normally around 500 m in rural vicinities in the early morning. The urban boundary layer tends to maximise during the midnight when the hotter air elevates to higher altitudes. Due to the high thermal resistance of the air, the surface layer has only a few centimetres depth. The air temperature in canopy layer reaches its maximum in the late afternoon when the urban canopy is being exposed to the solar radiation for many hours. The urban boundary layer, however, reaches its highest volume during the evening, when the warm air elevated through the lower atmosphere and forms the dome of warm air all over the urban area (Gartland, 2008; Oke, 1987; Sailor, 2015). The rural boundary layer waxes and wanes diurnally, with its maximum at the time of maximum surface heating. Table 1 shows that temperature differences decrease with increased spatial scale. It is likely to detect more temperature differences between North and South sides of a building facade than two different land uses in a precinct (building block versus park (Erell et al., 2011; Priyadarsini, 2009)). 3. Methods The UHI effect exists in all climates; however, its magnitude varies in different cities. Such variation exists partially due to a variety of measurement methods and reported scale (Stewart, 2011). The UHI scholarship uses five methods to measure the UHI effect including fixed stations, mobile traverse, remote sensing, vertical sensing and energy balance (Gartland, 2008). As Table 2 shows surface temperature measurement tends to indicate higher UHI intensity compared with air temperature measurements and simulation. As mentioned earlier, the magnitude of the UHI effect tends to be higher during calm and sunny weather in winter (Gartland, 2008). Therefore, this investigation uses winter data. To investigate the variations in near-surface air temperature in a city with a temperate climate, an urban heat measurement was done in Adelaide in winter 2013. Figure 3 shows that Adelaide metropolitan area provides a unique example for UHI investigation due to its parklands surroundings the CBD area, Adelaide Hills at its Eastern boundary and West Beach at its Western end. Thus, an East–West section of the Adelaide metropolitan includes green hills, outer suburban context, inner suburban context, urban parklands, city centre (CBD) and seashore areas, and provides a range of urban environments to discuss daily UHI effect variations. For summer and winter surface temperature analysis of Adelaide see: Guan et al. (2013), Clay et al. (2016), Sharifi et al. (2017), Sharma (2015), Hien (2016). 3.1. Mobile traverse UHI monitoring The mobile traverse method is an economical and fast way to draw the UHI profile of a city when the availability of fixed stations in the study area is limited and costly 532 Table 1 A. Soltani, E. Sharifi As the scale increases, the UHI effect gets moderated by air turbulence (developed based on: Gartland (2008)). Scale layer Focused temperature Focused elements Dimension range (approximate) Micro Surface Surface temperature 1–10 m Local Canopy Air temperature Macro Boundary Surface and air temperature Open Space skin Building rooftops Building facades Spaces between buildings Public spaces Streetscapes Urban precincts Land use classes Citywide 10–103 m 103–105 m Table 2 The magnitude of the UHI effect varies based on methods of data collection and type. Developed based on Rizwan et al. (2008, p. 123). Method 1 Tokyo (Japan) Atlanta (USA) Seoul (Korea) Method 2 Record method Data type Reported UHI effect Record method Data type Reported UHI effect Mobile traverse Simulated Surface temperature Air temperature 8.0 1C Satellite data 12.0 1C 0.6 1C Simulation Weather station Air temperature 3.4 1C Satellite data Surface temperature Surface temperature Surface temperature 1.2 1C 8.0 1C Figure 3 Mobile traverse path to measure UHI effect in Adelaide in winter 2013. (Map data: AEROmetrex, 2015; Google Earth, 2015). (Yow, 2007). To record a linear UHI profile of Adelaide with 1 km resolution, 30 synchronised fixed stations are required in a row, whereas it only needs a mobile station and a moving media to map a similar cross-section with 100 m resolution. In mobile traverse method, a portable weather station measures air temperature (plus radiant temperature and humidity if required) through linear or diagonal paths. Mode of transportation can vary based on the scale and accuracy of the investigation. Macro-scale studies use public transport or cars as the mean of transportation (Stewart, 2000), whereas micro-scale investigators use walking or cycling for moving through urban paths (Spronken-Smith and Oke, 1998). In both situations, the duration of each experiment is a critical factor since the weather conditions might change during the experiment. A majority of mobile traverse measurements last for a maximum of an hour and need data calibration to decrease the effect of weather variations (Gartland, 2008). Daily variation of urban heat island effect and its correlations to urban greenery 533 However, even within an hour, urban microclimates might noticeably change. In this experiment, the near-surface air temperature was measured during journeys in the early afternoon (14:00–14:35), mid-afternoon (16:10–16:45), late afternoon (18:00–18:35), early night (21:00–21:35) and midnight (22:45–23:20). Recorded data were moderated against official weather data of Australian Bureau of Meteorology at Roseworthy station (ID: 023122). Roseworthy station is located 56 km North of Adelaide CBD. Therefore, its data were assumed as rural station and are used to analyse the patterns of urban heat in Adelaide metropolitan. The recorded temperatures via mobile traverse monitoring were moderated based on official weather data of Australian Bureau of Meteorology at Kent Town station (ID: 023090) as follows: temperature changes during the journeys measured at Roseworthy station. A limitation of the mobile traverse method is related to the temperature sensors. The mobile weather sensor is commonly fixed on a moving vehicle such as a car or a bicycle. When the sensor is adjusted at the height of 1200–1500 mm above the ground, the measurement can be influenced by rough surfaces such as asphalt or paving or the moving vehicle radiant heat or exhaust gas. Also, to get a trusted measurement, the sensor needs to be held in each data recording locations for a while depending on sensor response rate, which increases the duration of the UHI measurement. Local traffic can also influence the measurement time span in the mobile traverse UHI monitoring method. T moderated ¼ T record −ΔT local station variation 4. 3.2. Recorded near-surface temperature in Adelaide is presented in Figure 5. During winter, urban heat is highly dependent to solar radiation and temperature drops significantly after sunset. Assessment of recorded data against weather data of the Australian Bureau of Meteorology's Roseworthy station indicates that the area around the Adelaide CBD experienced higher UHI effect compared to suburban areas during all measurements (see Figure 6). The near-surface UHI effect peaked at 5.9 1C during midnight in the CBD area. The Adelaide metropolitan area represents an urban heat island, in which stored heat during the afternoon is discharged to the atmosphere with a delay of up to six hours. Near-surface urban heat in Adelaide (compared to Roseworthy station) alters from a minimum of 1.5 1C in the early afternoon to a maximum of 5.9 1C in midnight during a typical sunny winter day (see Figure 6 and Table 3). Heat load accumulates during the afternoon in the built environment and is discharged to the atmosphere with delay during the night. However, such heat accumulation does not match the urban profile during the afternoon, when open spaces are exposed to the solar radiation. This shows the contribution of stored heat release and reduced longwave cooling in Adelaide's midnight UHI effect. Temperature sensor and data logging device The near-surface temperature was measured via an EXTECH RHT10 hygrometer data logger (temperature resolution 0.1 1C; accuracy 7 0.5 1C at 40 1C; range 40 1C to 70 1C; minimum sampling interval 2 s; response rate 10 s; data logging capacity: 16,000 records). The data logger unit was installed 1200 mm above the ground surface on a 200 mm pole on the left side of a passenger car (to prevent radiant heat from car engine in front and exhaust from back), and was covered by a white pipe cover to prevent the rapid effect of solar radiation (see Figure 4). Dry-bulb temperature and relative humidity were recorded every 10 s. In a pilot study prior to the main data collection with the same temperature data logger, it is identified that in real-world conditions it will take 30 s for EXTECH RHT10 to detect temperature differences of 0.1 1C while moving with the speed of 50 km/h). Each journey of 16.3 km took 30–35 min to complete depending on the local traffic. Duplicated data were cleaned afterwards to remove inevitable traffic light stoppages. Recorded temperatures were moderated based on Results Figure 4 Mobile temperature data logging device (EXTECH RHT10) installed 1200 mm above the ground surface attached to a utility vehicle. 534 A. Soltani, E. Sharifi Figure 5 Averaged near-surface temperature profile of Adelaide measured between 26 July and 15 August 2013. Figure 6 5. The UHI profile of Adelaide metropolitan (East–West) between 26 July and 15 August 2013. Discussion The UHI profile during the late afternoon has more thermal fluctuation along the travel path, especially around the boundaries of the CBD. Such thermal fluctuations resulted in up to 2 1C near-surface temperature differences in very short distances of less than 1 km from the city centre. Also, a considerable fluctuation is recorded in early afternoon and early night urban heat profiles when up to 1.2 1C temperature differences are recorded in CBD vicinities. The UHI effect had less variation during mid-afternoon and midnight (mid-afternoons in Adelaide usually become partly cloudy in sunny winter days; average cloud cover was 30% during this study). 5.1. Adelaide's UHI daily profile Having less temperature variation in Adelaide UHI effect during mid-afternoon and midnight supports the assumption that: Urban surface materials store heat in their thermal mass during the first hours of exposure to solar radiation. Urban surface covers start to emit the stored heat to the built environment in early afternoon. If solar radiation intensity decreases, UHI fluctuations decrease too, but the overall UHI intensity continues to increase during the next few hours. The UHI fluctuation peaks at late afternoon and early night when surface materials are exposed to solar radiation for the whole day. The UHI fluctuation decrease and the UHI intensity peaks when proceeding to midnight. Although the nocturnal UHI effect is concentrated in highly urbanised areas such as Adelaide CBD, the daytime urban heat can have different distribution patterns, and it may have very high magnitudes in some urban settings, lower intensity in other areas, and in some cases negative values in park cool islands. The midnight UHI effect had the most intense temperature differences (urbanrural) in Adelaide (refer to the higher values of mean, minimum, and maximum in Table 3). However, the afternoon urban heat has more temperature variance (point-to-point variation), especially during the late afternoon (refer to higher values of sample variance, standard deviation, and skewness in Table 3). The averaged near-surface urban heat was the highest in Adelaide during the midnight monitoring. However, the 6 1C higher temperature during winter nights, when the suburban temperature may be as low as 9 1C, is not a particular Daily variation of urban heat island effect and its correlations to urban greenery 535 Table 3 Descriptive analysis of averaged urban heat patterns in Adelaide metropolitan area, measured between 26 July and 15 August 2013. Mean Standard Error Median Standard Deviation Sample Variance Skewness Minimum UHI Maximum UHI Early afternoon (1C) Mid-afternoon (1C) Late afternoon (1C) Early night (1C) Midnight (1C) 1.93 0.02 1.88 0.31 0.09 0.38 1.47 2.73 3.15 0.03 3.34 0.40 0.16 0.62 2.30 3.75 4.62 0.05 4.58 0.66 0.44 0.08 3.40 5.81 4.78 0.03 4.83 0.36 0.13 0.60 3.93 5.37 5.30 0.03 5.41 0.40 0.16 0.33 4.56 5.90 Table 4 Correlation between ratio of tree canopy within 500 m of the mobile traverse path and average near-surface urban heat in Adelaide during winter 2013. West Beach Tree canopy Average UHI Average UHI Average UHI ratio afternoon (1C) night (1C) all recorded (1C) 20% 2.5 4.6 3.5 Outer suburbs East West 24% 2.9 4.8 3.9 18% 3 5.2 4.1 threat (in fact, it could be an advantage). Nevertheless, the midnight urban heat could be a threat during summer heatwaves with midnight minimum temperatures of more than 30 1C. The afternoon urban heat may be even more harmful during summer when solar radiation is more intense, days are longer, and the temperature profile is considerably higher than winter (Williams et al., 2012; Hatvani-Kovacs et al., 2016; Sharifi et al., 2017). The 2.4 1C temperature variations in the late afternoon could make a comfortable outdoor environment in summer uncomfortable to attend or pushes thermal discomfort towards stronger heat stress (the pilot study had been done in winter due to the higher reported magnitude the UHI effect in the literature). The UHI measurement in Adelaide indicates that: Some urban spaces store more heat compared to others. Such heat storage variations may occur over walking distances of 500–1000 m. The UHI effect is lower during the afternoon compared to midnight. However, near-surface temperature fluctuation is higher during the afternoon. The afternoon urban heat could be more harmful to humans during summer, although it provides better thermal comfort conditions during the winter. 5.2. Correlations between tree canopy ratio and UHI effect Adelaide has a unique urban structure with a CBD surrounded by parklands (see Figure 3). Recent research on the urban heat in Adelaide suggests that Adelaide CBD parklands Inner suburbs Parklands CBD Adelaide Hills r 15% 3.5 5.4 4.4 35% 3.1 4.3 3.7 13% 3.9 5.5 4.7 50% 2.7 4.3 3.5 0.52 0.85 0.72 effectively mitigate the urban heat of the CBD (Clay et al., 2016; Guan et al., 2013). In the late afternoon, the nearsurface air temperature in parklands was up to 2 1C lower than the CBD 1 1C cooler than most of the suburban areas. Parklands were cooler than inner and outer suburbs almost all day. As discussed in the last section urban air mixture during the night decreases the relative cooling effect of parkland by midnight. Previous studies indicate the strong negative correlations between urban surface temperature and tree canopy ratio (Guan et al., 2013; Sharifi et al., 2017). Table 4 presents the ratio of tree canopy within 500 m of the mobile traverse path of this case study (measured via iTree Canopy tool available at https://canopy.itreetools.org/). Adelaide CBD parklands have considerably higher tree canopy (35%) compared to both the CBD (13%) and inner suburban areas (15%). It also has two fold tree canopy than most outer suburbs. Figure 6 illustrates that the cooling effect of urban greenery is more significant during the late afternoon (immediately after sunset). The magnitude of UHI effect is averaged for afternoon and night time (see Table 4). The afternoon UHI effect in Adelaide has the correlation coefficient (r) value1 of 0.52 to the ratio of tree canopy which means a moderate downhill relationship exist between the 1 Correlation coefficient value varies between 0 and 7 1. It indicates the strength and direction of the relationship between variables (Bryman, 2008; Neuman, 2011). Correlation coefficient (r) values closer to +1 represent stronger positive relationships between the two variables, while closer r-values to 1 indicate strong negative dependency (i.e., variable A decreases with increase in variable B) and r-value = 0 indicate no connection. 536 A. Soltani, E. Sharifi two variables. Averaged nighttime UHI effect has the rvalue of 0.85 to the ratio of tree canopy and indicates a strong downhill relationship. In another word, a high ratio of tree canopy correlates strongly with cooler nocturnal temperatures in the built environment. 6. Policy implication UHI measurements are commonly reported during the night – when the urban–rural temperature differences are at their maximum; thus, they fall short in addressing the issue of heat stress on human participants. This UHI study of Adelaide indicates that daily heat stress may vary in the built environment within short distances of less than 1 km. Having thermally comfortable urban microclimates is a fundamental characteristic of healthy and vibrant public spaces (Gehl, 2010). Therefore, urban planning professionals and decision makers are required to consider diurnal heat stress alongside nocturnal UHI effect in planning liveable cities. Results of this study suggest that the maximum urban heat stress occurs during the afternoon when both overall temperature and daily urban heat are at their peak. Such daily heat stress in the built environment decreases in urban parklands, near forested areas and water bodies in cities with temperate climate such as Adelaide. Heat stress peaks in more developed urban settings associated with impermeable urban surface covers (Coutts et al., 2012). Thus, urban greenery and surface water can assist achieving, cooler and healthier urban environments in the context of climate change. With an emphasis on urban consolidation policies, natural landscapes in and around Australian cities are likely to be replaced by buildings and road surfaces over the medium term (SoAC, 2014–2015). This is likely to increase air temperature in critical summer months (Gartland, 2008). Current planning and building guidelines address the issue of urban heat via planning rules prescribing minimum open space standards for various types of dwelling on various lot sizes usually as a percentage of the block area. The nature of open space – lawns and trees or concrete or stone paving – is not prescribed. This has led to concrete and stone paving on sides and rear of new developments. The purpose of open space can be made effective towards mitigating UHI effect. Public spaces comprising of carparks, open spaces, roads and other transport networks can provide enough space to increase tree canopy by 30% without compromising the functionality of space and provide up to 2 1C cooler urban climates compared to business as usual scenario. Urban heat measurement and mitigation strategies need to be incorporated in urban policy making and development processes for more resilient urban futures. 7. Conclusions Urban temperatures are predicted to increase due to climate change (Cleugh et al., 2011; CSIRO, 2014). The temperatures in our cities are likely to increase up to 8 1C further due to the urban heat island effect. More heat is absorbed and emitted with delay by expanses of asphalt, concrete and other heat-storing building materials. In this context, it is crucial to understand the possibilities for the transformation of existing urban fabrics towards a more liveable and sustainable future (Bosselmann, 2008). This can be implemented by landscape transformation in existing urban spaces (Santamouris et al., 2011). A better understanding of daily urban heat variations in cities assists urban policy making and public life management in the context of climate change. Having thermally comfortable urban microclimates is a fundamental characteristic of healthy and vibrant public spaces (Gehl, 2010). This UHI study of Adelaide indicates that daily heat stress may vary within walkable distances of less than 1 km in urban settings. Therefore, urban planning professionals and decision makers are required to consider diurnal heat stress alongside nocturnal UHI effect in planning more resilient and liveable cities. The study also indicates that lack of tree canopy correlates to higher intensity of the UHI effect in temperate climate. Urban trees provide local shade and evaporative cooling. Increased urban greenery to reach the ideal ratio of 30% combined with water sensitive urban design can provide up to 2 1C cooler urban climates compared to business as usual scenario and assist achieving cooler and healthier urban environments in the context of climate change. Research limitations and further studies This case study is based on mobile traverse monitoring of UHI effect in Adelaide during winter. It is envisaged that more significant results may emerge from a similar setup during summer. This can be the subject of follow-up studies. In further research, more than one temperature sensor can be used in different heights to facilitate surface-air temperature correlations. Also, using globe temperature data loggers can extend research findings through combining air and surface temperature. Using a fixed weather stations network is the study area can reveal more comprehensive findings. However, it needs special reservations regarding sensors’ calibration and safety of their location. Methods and results can be further tested in other cities with similar and different climates. Acknowledgments This paper draws on the work of the authors at the University of South Australia. It is a part of a research project on Urban Microclimates of Sydney, Melbourne and Adelaide - supported by the CRC (RP2005) for Low Carbon Living and a range of industry and government stakeholders including the City of Adelaide. References AEROmetrex, 2015. (Cartographer). Andreou, E., 2013. Thermal comfort in outdoor spaces and urban canyon microclimate. Renew. Energy 55, 182–188. Arnfield, A.J., 2003. Two decades of urban climate research: a review of turbulence, exchanges of energy and water, and the urban heat island. Int. J. Climatol. 23 (1), 1–26. http://dx.doi. org/10.1002/joc.859. Daily variation of urban heat island effect and its correlations to urban greenery Ashie, Y., 2008. Management of Urban Heat Environment. Hanaki, K. (Ed.), Urban Environmental Management and Technology, 1. Springer Japan, Tokyo, pp. 215–238. Bosselmann, P., 2008. Urban Transformation: Understanding City Design and Form. Island Press, Washington DC. Bourbia, F., Boucheriba, F., 2010. Impact of street design on urban microclimate for semi arid climate (constantine). Renew. Energy 35 (2), 343–347. http://dx.doi.org/10.1016/j.renene.2009.07.017. Bryman, A., 2008. Social Research Methods. Oxford University Press, Oxford. Clay, R., Guan, H., Wild, N., Bennett, J., kumar,, Vinod, Ewenz, C., 2016. Urban heat island traverses in the city of Adelaide, South Australia. Urban Clim. 17, 89–101. http://dx.doi.org/10.1016/ j.uclim.2016.06.001. Cleugh, H., Smith, M.S., Battaglia, M., Graham, P., 2011. Climate Change in Australia: Technical Report. CSIRO, Aspendale VIC. Coutts, A., Tapper, N., Beringer, J., Loughnan, M., Demuzere, M., 2012. Watering our cities: the capacity for water sensitive urban design to support urban cooling and improve human thermal comfort in the Australian context. Progr. Phys. Geogr., 1–27. CSIRO, 2014. State of the Climate Aspendale VIC: CSIRO and Australian Bureau of Meteorology. Erell, E., 2008. The application of urban climate research in the design of cities. Adv. Build. Energy Res. 2 (1), 95–121. Gartland, L., 2008. Heat Islands: Understanding and Mitigating Heat in Urban Areas. Earthscan, Washington. Gehl, J., 2010. Cities for People. Island Press, Washington, DC. Google Earth, 2015. (Cartographer). Guan, H., Bennett, J., Ewenz, C., Benger, S., Vinodkumar, Zhu, S., Soebarto, V., 2013. Characterisation, Interpretation and Implications of the Adelaide Urban Heat Island. Flinders University, Adelaide. Hatvani-Kovacs, G., Belusko, M., Pockett, J., Boland, J., 2016. Assessment of heatwave impacts. Proc. Eng. 169, 316–323. http: //dx.doi.org/10.1016/j.proeng.2016.10.039. Hien, W.N., Urban heat island research: Challenges and potential, Frontiers of Architectural Research, 5(2), 2016, 276-278, ISSN 2095-2635, http://dx.doi.org/10.1016/j.foar.2016.04.001. Ichinose, T., Matsumoto, F., Kataoka, K., 2008. Counteracting Urban Heat Islands in Japan. In: Peter, D. (Ed.), Urban Energy Transition: From Fussil Fuels to Renewable Power. Elsevier, Amsterdam, pp. 365–380. Johansson, E., 2006. Influence of urban geometry on outdoor thermal comfort in a hot dry climate: a study in Fez, Morocco. Build. Environ. 41 (10), 1326–1338. http://dx.doi.org/10.1016/ j.buildenv.2005.05.022. Karatasou, S., Santamouris, M., Geros, V., 2006. Urban Building Climatology. In: Santamouris, M. (Ed.), Environmental Design of Urban Buildings: An Integrated Approach. Earthscan, London, pp. 95–119. Kolokotsa, D., Psomas, A., Karapidakis, E., 2009. Urban heat island in Southern Europe: the case study of Hania, Crete. Sol. Energy 83 (10), 1871–1883. http://dx.doi.org/10.1016/j.solener.2009.06.018. Krüger, E.L., Minella, F.O., Rasia, F., 2011. Impact of urban geometry on outdoor thermal comfort and air quality from field measurements in Curitiba, Brazil. Build. Environ. 46 (3), 621–634. Lin, T.P., Matzarakis, A., Hwang, R.L., 2010. Shading effect on longterm outdoor thermal comfort. Build. Environ. 45 (1), 213–221. http://dx.doi.org/10.1016/j.buildenv.2009.06.002. Mirzaei, P.A., Haghighat, F., 2010. Approaches to study urban heat island – abilities and limitations. Build. Environ. 45 (10), 2192–2201. http://dx.doi.org/10.1016/j.buildenv.2010.04.001. 537 Morris, C., Simmonds, I., Plummer, N., 2001. Quantification of the influences of wind and cloud on the nocturnal urban heat island of a large city. J. Appl. Meteorol. 40 (2), 169–182. Neuman, W.L., 2011. Social Research Methods: Qualitative and Quantitative Approaches 7th ed. Pearson/Allyn & Bacon, Boston. Nikolopoulou, M., 2011. Urban Open Spaces and Adaptation to Climate Change. In: Richter, M., Weiland, U. (Eds.), Applied Urban Ecology:: A Global Framework. Wiley-Blackwell, Chichester, pp. 106–122. Oke, T.R., 2006a. Initial Guidance to Obtain Representative Meteorological Observations at Urban Sites: Instruments and Observing Methods. World Meteorological Organization, Canada (IOM Report No. 81). Oke, T.R., 2006b. Towards better scientific communication in urban climate. Theor. Appl. Climatol. 84 (1), 179–190. http://dx.doi. org/10.1007/s00704-005-0153-0. Rizwan, A., Dennis, L., Liu, C., 2008. A review on the generation. Determ. Mitig. Urban Heat. Isl. 20 (1), 120–128. Runnalls, K.E., Oke, T.R., 2000. Dynamics and controls of the nearsurface heat island of Vancouver, British Columbia. Phys. Geogr. 21 (4), 283–304. http://dx.doi.org/10.1080/02723646.2000.10642711. Samuels, R., Randolph, B., Graham, P., McCormick, T., Pollard, B., 2010. Micro-Urban-Climatic Thermal Emissions: in a MediumDensity Residential Precinct. Sydney. Santamouris, M., Cartalis, C., Synnefa, A., 2015. Local urban warming, possible impacts and a resilience plan to climate change for the historical center of Athens, Greece. Sustain. Cities Soc. 19, 281–291. http://dx.doi.org/10.1016/j.scs.2015.02.001. Santamouris, M., Synnefa, A., Karlessi, T., 2011. Using advanced cool materials in the urban built environment to mitigate heat islands and improve thermal comfort conditions. Sol. Energy 85 (12), 3085–3102. Soltani, A., Mehraein, M., Sharifi, E., 2012. Urban features and energy consumption at local level. J. Urban Environ. Eng. 6 (2), 43–47. http://dx.doi.org/10.4090/juee.2012.v6n2.043047. Sharifi, E., Lehmann, S., 2014. Comparative analysis of surface urban heat island effect in central Sydney. J. Sustain. Dev. 7 (3), 23–34. http://dx.doi.org/10.5539/jsd.v7n3p23. Sharifi, E., Sivam, A., Boland, J., 2016. Resilience to heat in public space: a case study of Adelaide, South Australia. J. Environ. Plan. Manag. 59 (10), 1833–1854. http://dx.doi.org/10.1080/ 09640568.2015.1091294. Sharifi, E., Sivam, A., Karuppannan, S., Boland, J., 2017. Landsat Surface Temperature Data Analysis for Urban Heat Resilience: Case Study of Adelaide. In: Geertman, S., Allan, A., Pettit, C., Stillwell, J. (Eds.), Planning Support Science for Smarter Urban Futures. Springer, pp. 433–448. Sharma, A., 2015. Urban greenways: Operationalizing design syntax and integrating mathematics and science in design, Frontiers of Architectural Research, 4(1), 24-34, ISSN 2095-2635, http://dx. doi.org/10.1016/j.foar.2014.11.002. SoAC, 2014&;2015. State of Australian Cities. Department of Infrastructure and Transport, Commonwealth of Australia, Major Cities Unit, Canberra. Spronken-Smith, R.A., Oke, T.R., 1998. The thermal regime of urban parks in two cities with different summer climates. Int. J. Remote Sens. 19 (11), 2085–2104. http://dx.doi.org/10.1080/ 014311698214884. Stewart, I.D., 2000. Influence of meteorological conditions on the intensity and form of the urban heat island effect in Regina. Can. Geogr. 44 (3), 271–285. http://dx.doi.org/10.1111/ j.1541-0064.2000.tb00709.x. Stone, B., 2012. City and the Coming Climate: Climate Change in the Places we Live. Cambridge University Press, New York. 538 Svensson, M.K., 2004. Sky view factor analysis–implications for urban air temperature differences. Meteorol. Appl. 11 (3), 201–211. Williams, S., Nitschke, M., Sullivan, T., Tucker, G.R., Weinstein, P., Pisaniello, D.L., Bi, P., 2012. Heat and health in Adelaide, South Australia: assessment of heat thresholds and temperature relationships. Sci. Total Environ. 414, 126–133. http://dx.doi. org/10.1016/j.scitotenv.2011.11.038. A. Soltani, E. Sharifi Wong, N.H., Jusuf, S.K., 2010. Study on the microclimate condition along a green pedestrian canyon in Singapore. Archit. Sci. Rev. 53 (2), 196–212. Wong, N.H., Yu, C., 2008. Tropical Urban Heat Islands: Climate, Buildings and Greenery. Taylor & Francis, New York. Yow, D.M., 2007. Urban heat islands: observations, impacts, and adaptation. Geogr. Compass 1 (6), 1227–1251. http://dx.doi. org/10.1111/j.1749-8198.2007.00063.x.