Molecular Biology of Gene Lecture Notes PDF
Document Details
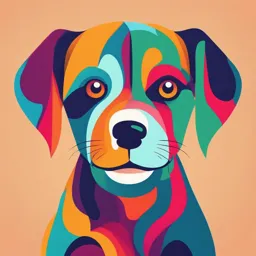
Uploaded by IdolizedLandArt
Dr.Nuha Joseph Kandala
Tags
Related
- Becker's World of the Cell Tenth Edition Chapter 16 PDF
- Introduction to Molecular Biology PDF
- Molecular Regulation and Signaling (Lesson 3) PDF
- Quiz-Decoding the Genome: A Quiz on Gene Structure and Transcription PDF
- Lecture 01, Eukaryotic Gene Structure & Organization PDF
- Non-Coding DNA & Gene Structure - PDF
Summary
These lecture notes cover supercoiling, a crucial aspect of DNA structure. The notes detail how DNA twists around itself and how this affects its overall structure, including concepts of positive and negative supercoiling and the role of topoisomerases. The notes also include diagrams and figures to illustrate the concepts.
Full Transcript
# Molecular Biology of Gene ## Lecture (4): Supercoiling affects the structure of DNA - Within the cell, the DNA helix is wound up into coils, known as supercoiling. - The consequences of supercoiling depend on whether the DNA is twisted around itself in the same sense as the two strands within the...
# Molecular Biology of Gene ## Lecture (4): Supercoiling affects the structure of DNA - Within the cell, the DNA helix is wound up into coils, known as supercoiling. - The consequences of supercoiling depend on whether the DNA is twisted around itself in the same sense as the two strands within the double helix (clockwise) or in the opposite sense. Twisting in the same sense produces positive supercoiling. - This has the effect of causing the DNA strands to wound around one another more tightly so that there are more base pairs per turn. - Twisting in the opposite sense produces negative supercoiling. This causes the DNA strands to be rotated around one another less tightly, so there are fewer base pairs per-turn. ### Figure (1): Interaction between twisting and supercoiling. (a) A ribbon with a single complete twist, without supercoiling. (b) The same ribbon, is allowed to form a supercoil. - Closed DNA molecules of identical sequences may have different linking numbers, reflecting different degrees of supercoiling. - Molecules of DNA that are the same except for their linking numbers are called topological isomers. The level of supercoiling within a particular DNA molecule can be described by its linking number (Lk). - This number corresponds to the number of double helical turns in the original linear molecule (the number of times one strand crosses over the other in space). There are three parameters involved: - Twist (T): the number of turns of the duplex, it is determined by the number of base pairs per turn. - For a relaxed closed circular DNA lying flat in a plane, the twist is the total number of base pairs divided by the number of base pairs per turn. - Linking number (L): represents the turning of the axis of the duplex in space. - Writhe (W): essentially a measure of the degree of supercoiling, it can be considered as the number of times the strip crosses over itself in a defined direction. For a relaxed molecule, W = 0, and the linking number equals the twist. These two parameters vary according to the conformation: - in (a), there is one twist (T=1) but no supercoiling (W=0), - while in (b) there is no twist (T=0) and the strip crosses itself once (W=1). - The linking number is therefore a measure of the overall twisting of the strip, or for DNA, the total number of times that the DNA strands wrap around one another. - If the ends of the strip are not free to rotate, then the linking number will remain constant. - Most of the DNA molecules we will be considering are circular, and therefore do not contain ends that can rotate. ### Figure (2): Supercoiling of a circular molecule. (a) The 'molecule' has one twist and no supercoils. (b) and (c) introduces negative supercoils and increases the amount of twisting. - Since the linking number (L) remains the same, the three forms are interchangeable without breaking the circle. The Action of topoisomerases is shown in Figure (3): - Structure A is not supercoiled: the two crossing points are of opposite signs and cancel one another. - The topoisomerase makes a double strand break between L and M, passes the X-Y region through the gap and reseals the break between L and M. This changes the signs of W at that point, so structure B is now negatively supercoiled. ### Figure (3): Topoisomerases relax or introduce supercoils in DNA. - Changes in the topology of DNA can be caused in several ways. - In order to start replication or transcription, the two strands of DNA must be unwound. - In the case of replication, the two strands separate permanently, and each reforms a duplex with the newly-synthesized daughter strand. - In the case of transcription, the movement of RNA polymerase creates a region of positive supercoiling in front and a region of negative supercoiling behind the enzyme. This must be resolved before the positive supercoils impede the movement of the enzyme. ### Figure (4) - When a circular DNA molecule is replicated, the circular products may be catenated, with one passed through the other. - They must be separated in order for the daughter molecules to segregate to separate daughter cells. - Yet another situation in which supercoiling is important is the folding of the DNA thread into a chain of nucleosomes in the eukaryotic nucleus. - All of the situations are resolved by the actions of topoisomerases. - Topoisomerases are enzymes tha catalyze changes in the topology of DNA by transiently breaking one or both strands of DNA, passing the unbroken strand(s) through the gap, and then resealing the gap. - The ends that are generated by the break are never free, they are covalently linked to the enzyme. - The common action for all topoisomerases is to link one end of each broken strand to a tyrosine residue in the enzyme. - A type I enzyme links to the single broken strand (act by breaking single strand of DNA); a type II enzyme links to one end of each broken strand (act by breaking double strand of DNA). - The topoisomerases are further divided into the A and B groups according to whether the linkage is to a 5' phosphate or 3' phosphate. - The use of the transient phosphodiester-tyrosine bond suggests a mechanism for the action of the enzyme; it transfers a phosphodiester bond(s) in DNA to the protein, manipulates the structure of one or both DNA strands, and then rejoins the bond(s) in the original strand. - The E. coli enzymes are all of type A, using links to 5 phosphate. - This is the general pattern for bacteria. - All four possible types of topoisomerase (IA, IB, IIA, IIB) are found in eukaryotes. - The enzyme binds to a region in which duplex DNA becomes separated into its single strands; then it breaks one strand, pulls the other strand through the gap, and finally seals the gap. - The transfer of bonds from nucleic acid to protein explains how the enzyme can function without requiring any input of energy, their energy has been conserved through the transfer reactions. ## Separation of Topoisomers by Electrophoresis - DNA molecules of different sizes are routinely separated by electrophoresis on agarose gels. - The mobility of a DNA molecule in such a gel depends on both its molecular weight and its conformation. - Heavier molecules travel more slowly but among molecules of the same molecular weight, those that are more compact move faster. - Consequently, for a given DNA molecule, supercoiled cccDNA moves faster than open circular DNA, which in turn moves faster than linearized DNA. - For small circular molecules of DNA, it is even possible to separate topoisomers with different numbers of superhelical twists. - The more superhelical twists, the more compact the molecule is and the faster it moves in an electrophoretic field (Figure - 5). ### Figure (5): Separation of Supercoiled DNA by Electrophoresis - Supercoiled DNA molecules, all of identical sequence, were electophoresed to reveal multiple bands, with each band differing in the number of supercoils. - The number of supercoils is shown beside the band. - Zero refers to open circular DNA, which is not supercoiled. ## Histones Package DNA in Eukaryotes - Plants and animals have vastly more DNA than bacteria and must fold this DNA to fit into the cell nucleus. - Typical bacteria carry approximately 4,000 genes on a single chromosome, which is about one millimeter long. - The chromosome is thus 1,000 times longer than the bacterial cell in which it fits. - Eukaryotic chromosomes may be as much as a centimeter long and must be folded up to fit into the cell nucleus, which is five microns across, a necessity for a 2,000-fold shortening. - Each cell within our body contains a huge amount of DNA chromosomes of the human genome contain approximately 3.2 × 10^9 base pairs of DNA. - Since we are diploid organisms, the total amount of DNA in most of our cells totals 6.4×10^9 base pairs At 0.33 nm per base pair, this corresponds to an overall length of approximately 2.1 m. - The answer is that the DNA is highly compacted. It is associated with a number of proteins that results in the wrapping of DNA into nucleosomes. - However, eukaryotic chromosomes are not circular, and instead of supercoiling using DNA gyrase, the mechanism of packaging involves winding the DNA around special proteins, the histones. - Eukaryotic DNA starts folding by coiling around the histones, positively charged proteins that neutralize the negative charge of the DNA itself. - DNA with histones bound to it was named chromatin when it was first discovered in chromosomes, chromatin fibers are composed of linear arrays of spherical particles called nucleosomes (v-bodies). - Chromatin consists of roughly spherical subunits, the nucleosomes, each containing approximately 200 bp of DNA and nine histones, two each of H2A, H2B, H3 and H4 and one of H1 (Figure.- 6). ### Figure (6) - The histones play the most essential structural role. - A nucleosome core particle consists of two copies each of histones H2A, H2B, H3 and H4 to form a histone octamer and two coils of DNA are wrapped in a left-handed superhelix, which completes about 1.7 turns per nucleosome. - wrapped around them. Each coil of DNA is approximately 75bp long so that this core particle accommodates 150 bp of DNA overall. - The remaining 50 bp or so of DNA forms a linker region between neighboring core particles. - The proteins associated with DNA in chromatin are divided into basic, positively charged histones and less positively charged non-histones. - Histones contain large amounts of the positively charged amino acids lysine and arginine, making it possible for them to bind through electrostatic interactions to the negatively charged phosphate groups of the DNA nucleotides. - Histone H1, functions to seal the DNA around the nucleosome. - The formation of nucleosomes represents the first level of packaging, whereby the DNA is reduced to about one-third of its original length. - Instead, the 10 nm chromatin fiber is further packed into a thicker 30 nm fiber, which was originally called a solenoid. - The formation of the 30 nm fiber creates a second level of packaging, in which the overall length of the DNA is reduced some two fold. - The 30 nm fibre forms a series of looped domains that further condense the structure of the chromatin fiber. - The fibers are then coiled into the chromosome arms that constitute a chromatid. - In the overall transition from fully extended DNA helix to the extremely condensed status of the mitotic chromosome, a packaging ratio of about 500:1 must be achieved. ### Figure (7) - The core histones have a body of about 80 amino acids and a tail of 20 amino acids at the N-terminal end. - This tail contains several lysine residues that may have acetyl groups added or removed (Figure -8). - This is thought to partly control the state of DNA packaging and hence of gene expression. - Thus, in active chromatin, the core histones are highly acetylated. ### Figure (8) - Histone Tails May Be Acetylated - The N-terminal domains of some of the histone proteins are free for acetylation as indicated by "acetyl." - The single letter system for naming amino acids is used. ## Summary of Chromosome Folding | Level of Folding | Consists of | Base Pairs per Turn | |---|---|---| | DNA double helix | nucleotides | 10 | | Nucleosomes | 200 base pairs each | 100 | | 30 nanometer fiber | 6 nucleosomes per turn | 1,200 | | Loops | 50 helical turns per loop | 60,000 | | Chromatid | 2,000 loops | | ## Histones Are Highly Conserved and Originated Among the Archaebacteria - Of all known proteins, the eukaryotic core histones, especially H3 and H4, are the most highly conserved during evolution. - For example, only two amino acids are different, out of 102, between the H4 of cows and peas. - The linke histone, H1, is more variable in composition. - Typical bacteria (i.e., the eubacteria) do not possess histones. - Large numbers of "histone-like proteins" are found bound to bacterial chromosomes. - Despite the name, these are not homologous in sequence to true histones nor do they form nucleosomes for packaging DNA. - However, some members of the genetically distinct lineage of archaebacteria (e.g., the methane bacteria), do possess histones. - Archaeal histones vary significantly from each other. - They are 65–70 amino acids long and are missing the tails characteristic of eukaryotic histones. - Archaeal nucleosomes accommodate a little under 80 bp of DNA and contain a tetramer of the archaeal histone. They are probably homologous to the (H3 + H4)2 tetramers found in the core of the eukaryotic nucleosome. ## Terms: - chromatin: Complex of DNA plus protein which constitutes eukaryotic chromosomes - histone: Special positively charged protein that binds to DNA and helps to maintain the structure of chromosomes in eukaryotes - nucleosome: Subunit of a eukaryotic chromosome consisting of DNA coiled around histone proteins. - 30 nanometer fiber: Chain of nucleosomes that is arranged helically, approximately 30 nm in diameter - euchromatin: Normal chromatin, as opposed to heterochromatin. - heterochromatin: A highly condensed form of chromatin that cannot be transcribed because it cannot be accessed by RNA polymerase - chromatid: Single double-helical DNA molecule making up whole or half of a chromosome. A chromatid also contains histones and other DNA associated proteins. - potential intrastrand triplex (PIT): Stretch of DNA that might be expected from its sequence to form H-type triplex DNA - Hoogsteen base pair: A type of nonstandard base pair found in triplex DNA, in which a pyrimidine is bound sideways on to a purine - allele: One particular version of a gene, or more broadly, a particular version of any locus on a molecule of DNA - bp: Abbreviation for base pair(s) - locus (plural, loci): A place or location on a chromosome; it may be a genuine gene or just any site with variations in the DNA sequence that can be detected, like RFLPs or VNTRS - Mbp: Megabase pairs or million base pairs - non-coding DNA: DNA that does not code for proteins or functional RNA molecules - exon: Segment of a gene that codes for protein and that is still present in the messenger RNA after processing is complete - intergenic DNA: Non-coding DNA that lies between genes - intervening sequence: An alternative name for an intron - intron: Segment of a gene that does not code for protein but is transcribed and forms part of the primary transcript - primary transcript: The original RNA molecule obtained by transcription from a DNA template, before any processing or modification has occurred - consensus sequence: Idealized base sequence consisting of the bases most often found at each position. - pseudogene: Defective copy of a genuine gene - repeated sequences: DNA sequences that exist in multiple copies - repetitive sequences: Same as repeated sequences - highly repetitive DNA: DNA sequences that exist in hundreds of thousand of copies - long interspersed element (LINE): Long sequence found in multiple copies that makes up much of the moderately repetitive DNA of mammals - moderately repetitive sequence: DNA sequences that exist in thousands of copies (but less than a hundred thousand) - short interspersed element (SINE): Short sequence found in multiple copies that makes up much of the highly or moderately repetitive DNA of mammals - Alu element: An example of a SINE, a particular short DNA sequence found in many copies on the chromosomes of humans and other primates - B1 element: An example of a SINE found in mice; the precursor sequence from which the human Alu element evolved - satellite DNA: Highly repetitive DNA of eukaryotic cells that is found as long clusters of tandem repeats and is permanently coiled tightly into heterochromatin - tandem repeats: Repeated sequences of DNA (or RNA) that lie next to each other - unequal crossing over: Crossing over in which the two segments that cross over are of different lengths; often due to misalignment during pairing of DNA strands - centromere: Structure found on a chromosome and used to build and organize microtubules during mitosis - centromere sequence (CEN): A recognition sequence found at the centromere and needed for attachment of the spindle fibers - DNA fingerprint: Individually unique pattern due to multiple bands of DNA produced using restriction enzymes, separated by electrophoresis and usually visualized by Southern blotting - heterochromatin: Highly condensed form of chromatin that is genetically inert - junk DNA: Defective selfish DNA that is of no use to the host cell it inhabits and which can no longer move or express its genes - mini-satellite: Another term for a VNTR (variable number tandem repeats) - variable number tandem repeats (VNTR): Cluster of tandemly repeated sequences in the DNA, whose number of repeats differs from one individual to another - selfish DNA: A sequence of DNA that manages to replicate but which is of use to the host cell it inhabits - inverted repeat: Sequence of DNA that is the same when read forwards as when read backwards, but on the other complementary strand. One type of palindrome - mirrorlike palindrome: Sequence of DNA that is the same when read forwards and backwards on the same strand. One type of palindrome - palindrome: A sequence that reads the same backwards as forwards - hairpin: A double stranded base-paired structure formed by folding a single strand of DNA or RNA back upon itself - stem and loop: Structure made by folding an inverted repeat sequence - bent DNA: Double helical DNA that is bent due to several runs - negative supercoiling: Supercoiling with a left handed or counter clockwise twist - supercoiling: Higher level coiling of DNA that is already a double helix ### Table 4.03: Components of the Eukaryotic Genome | Component | Number of copies | |---|---| | Unique sequences | | | Protein encoding genes-comprising upstream regulatory region, exons and introns | | | Genes encoding non-translated RNA (snRNA, snoRNA, 7SL RNA, telomerase RNA, Xist RNA, a variety of small regulatory RNAs) | | | Non-repetitive intragenic non-coding DNA | | | Interspersed Repetitive DNA | | | Pseudogenes | | | Short Interspersed Elements (SINES) | | | Alu element (300 bp) | 1,000,000 copies | | MIR families (average -130 bp) (mammalian-wide interspersed repeat) | 400,000 copies | | Long Interspersed Elements (UNEs) | | | UNE-1 family average -800 bp) | 200,000 - 500,000 copies | | UNE-2 family (average -250 bp) | 270,000 copies | | Retrovirus like elements (500-1300 bp) | 250,000 coples | | DNA transposons (variable; average -250 bp) | 200,000 copies | | Tandem Repetitive DNA | | | Ribosomal RNA genes | 5 clusters of about 50 tandem repeats on 5 different chromosomes | | Transfer RNA genes | multiple copies plus several pseudogenes | | Telomere sequences | several kb of a 6 bp tandem repeat | | Mini-satellites (- VNTRS) | blocks of 0.1 to 20 kbp of short tandem repeats (15-50 bp), most located close to telomeres | | Centromere sequence (u-satellite DNA) | 171 bp repeat, binds centromere proteins | | Satellite DNA | blocks of 100 kbp or longer of tandem repeats of 20 to 200 bp, most located close lo contromoros | | Mega-satellite DNA | blocks of 100 kbp or longer of tandem repeats of 1 to 5 kbp, various locations |