Introduction to Medical Biology and Genetics PDF
Document Details
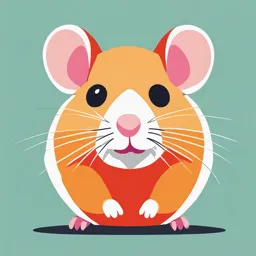
Uploaded by ImprovingOxygen1016
Okan University
Dr. Hilal Eren Gözel
Tags
Related
- Chapter VIII - Medical Biology - Genetics 2023-2024 PDF
- Introduction To Medical Biology And Genetics PDF
- Introduction To Medical Biology PDF
- Medical Biology and Genetics Lecture Notes 2024 PDF
- Kocaeli Health And Technology University Medical Biology and Genetics Lecture 2 2024 PDF
- Introduction to Medical Biology and Genetics PDF
Summary
This document provides an introduction to medical biology and genetics. It discusses the universal features of cells, such as their use of DNA and ATP, and the differences between prokaryotic and eukaryotic cells. It also highlights the divisions of life on Earth, including the roles of prions, viroids, and viruses.
Full Transcript
Introduction to Medical Biology and Genetics Dr. Hilal Eren Gözel Contact Info [email protected] 1st Floor, Room #129 Universal features of cells There are more than 10 million species on Earth. Although each species is different, they all rep...
Introduction to Medical Biology and Genetics Dr. Hilal Eren Gözel Contact Info [email protected] 1st Floor, Room #129 Universal features of cells There are more than 10 million species on Earth. Although each species is different, they all reproduce yielding a progeny that represent the copies of themselves (Heredity). This hereditary information drives a complex system of chemical processes that are crucial for the cell organization and its survival. Although, the organisms can be unicellular or multicellular(like us), the whole organism has been generated by cell divisions from a single cell. The single cell, therefore, is the vehicle for all of the hereditary information that defines each species. Universal features of cells All cells store their hereditary information in the same chemical code system: DNA (or RNA for viruses) DNA or RNA codes (A, C, G, T or U) and their structures are universal in all organisms. The mechanisms that take part in reading the hereditary information (Protein synthesis) or replication of it, occur very similarly in different species. All cells use proteins as catalysts (to increase rate of chemical reactions). All cells use ATP as their free energy source. All cells are enclosed in a plasma membrane across which nutrients and waste materials must pass. All cells have ribosomes. Differences of Cells: Size Shape Chemical requirements 1 mm Similar And Different! The scientific study of how living things are classified is called taxonomy. Similar And Different! The binomial nomenclature system combines two names into one to give all species unique scientific names. Divisions of life Cells are the basic structural and functional units of every organism. They have two distinct types: Prokaryotic and Eukaryotic. Prokaryotic cells: Bacteria and Archaea Eukaryotik cells: Protists, fungi, animals, and plants Prion, Viroid and Virus!!! Divisions of life Prokaryotic cells Eukaryotic cells No nuclear membrane (Nucleoid) True nucleus, consisting of nuclear membrane No Membrane-enclosed organelles Lysosomes, Golgi complex, endoplasmic reticulum, mitochondria & chloroplasts Cell Wall: Usually present; chemically complex (typical Cell Wall: When present, chemically simple bacterial cell wall includes peptidoglycan) Plasma membrane: No carbohydrates and generally lacks Plasma membrane: Sterols and carbohydrates that serve as sterols receptors present Cytoplasm: Simpler cytoskeleton Cytoplasm: Cytoskeleton; cytoplasmic streaming Ribosomes smaller in size (70S) Larger size (80S) Single circular DNA, lacks histones Linear DNA with histones Cell division: Binary Division Cell division: Mitosis Sexual reproduction: No meiosis; transfer of DNA fragments Meiosis only (conjugation) Divisions of life present in (ribosome-studded) and smooth regions Nucleolus: nonmembranous mal cells, structure involved in production cluster of of ribosomes; a nucleus has NUCLEUS ANIMATION Rough ER Smooth ER Nuclear envelope Rough www.mast within an one or more nucleoli endoplasmic NUCLEUS Nucleolus Smooth BioFlix® 3-D he plasma reticulum endoplasmic Animal Cell membrane Chromatin: material consisting Chromatin reticulum of DNA and proteins; visible in a dividing cell as individual me: region condensed chromosomes the cell’s bules are ontains a centrioles Plasma membrane: Ribosomes (small b membrane enclosing the cell Central vacuole: promin in older plant cells; func breakdown of waste pr N: Golgi apparatus macromolecules; enlarg shape; major mechanism of pla movement; e made of Microfilament es: Intermediate aments filaments Microtubules aments Ribosomes (small brown tubules dots): complexes that make proteins; free in cytosol or bound to rough ER or nuclear Mitochondrion envelope Peroxisome li: Chloroplast: photosynthe at Plasma membrane organelle; converts energ l’s sunlight to chemical ener ea stored in sugar molecules Cell wall: outer Golgi apparatus: organelle layer that maintains active cell’s shape and protects cell from in synthesis, modification, sorting, mechanical damage; made of cellulose, and secretion of cell products other polysaccharides, and protein Plasmodesmata: cytoplasmic In plant cell channels through cell walls that Chloroplast connect the cytoplasms of Central vacu Wall of adjacent cell Cell wall ganelle adjacent cells Plasmodesm ecialized In animal cells but not plant cells: ions; Lysosome: digestive Lysosomes Mitochondrion: organelle where organelle where Differences Between Plant and Animal Cells Differences Between Plant and Animal Cells Divisions of life Prion (Proteinaceous infectious particle): Prions are not considered living organisms because they are misfolded protein molecules which may propagate by transmitting a misfolded protein state. If a prion enters a healthy organism, it induces existing, properly folded proteins to convert into the misfolded prion form. In this way, the prion acts as a template to guide the misfolding of more proteins into prion form. Divisions of life Bovine spongiform encephalopathy (BSE): It is commonly known as mad cow disease, is a neurodegenerative disease of cattle. Symptoms include abnormal behavior, trouble walking, and weight loss. Time from onset of symptoms to death is generally weeks to months. Clinical Correlation Creutzfeldt–Jakob disease (CJD): It is a form of brain damage that leads to a rapid decrease of movement and mental function. Divisions of life - Virus Virus: A virus is a small infectious agent that replicates only inside the living cells of other organisms. They reproduce by creating multiple copies of themselves through self-assembly by using host’s metabolism. This infectious particle consisting of nucleic acid enclosed in a protein coat(capsid) and, for some viruses, surrounded by a membranous envelope. No organelles, only some genes. Their genomes may consist of double-stranded DNA, single-stranded DNA, double- stranded RNA, or single-stranded RNA, depending on the type of virus. Divisions of life RNA Capsomere DNA Membranous envelope RNA Capsid Head DNA Virus: Capsomere Tail of capsid sheath Tail fiber Glycoprotein Glycoproteins 18 × 250 nm 70–90 nm (diameter) 80–200 nm (diameter) 80 × 225 nm 20 nm 50 nm 50 nm 50 nm (a) Tobacco mosaic virus has (b) Adenoviruses have an (c) Influenza viruses have an (d) Bacteriophage T4, like a helical capsid with the icosahedral capsid with a outer envelope studded with other “T-even” phages, has a overall shape of a rigid rod. glycoprotein spike at each glycoprotein spikes. The complex capsid consisting of vertex. genome consists of eight an icosahedral head and a different RNA molecules, each tail apparatus. wrapped in a helical capsid. Clinical Correlation: Influenza viruses Seasonal influenza (the flu) is an acute respiratory infection caused by influenza viruses. It is common in all parts of the world. Most people recover without treatment! Influenza spreads easily between people when they cough or sneeze. Vaccination is the best way to prevent the disease. World Health Organization – Facts There are around a billion cases of seasonal influenza annually, including 3–5 million cases of severe illness. It causes 290 000 to 650 000 respiratory deaths annually. Ninety-nine percent of deaths in children under 5 years of age with influenza-related lower respiratory tract infections are in developing countries. There are four types of influenza viruses: A, B, C, and D. Influenza A and B viruses cause seasonal epidemics of disease in people (known as flu season) almost every winter. Influenza A viruses are the only influenza viruses known to cause flu pandemics (i.e., global epidemics of flu disease). A pandemic can occur when a new and different influenza A virus emerges that infects people, has the ability to spread efficiently among people, and against which people have little or no immunity. Influenza C virus infections generally cause mild illness and are not thought to cause human epidemics. Influenza D viruses primarily affect cattle but are not known to infect people to cause illness. Influenza A viruses Influenza A viruses are classified by subtypes based on the properties and combinations of their surface proteins: [hemagglutinin (HA), neuraminidase (NA)]. RNP: Ribonucleoprotein HA binds to a virus receptor on the host cell and mediates virus attachment to the cell surface. The hydrolytic enzyme NA cleaves sialic acid from viral receptors and Influenza viruses Swine flu Influenza viruses Mahal, A., Duan, M., Zinad, D. S., Mohapatra, R. K., Obaidullah, A. J., Wei, X.,... & Zhu, Q. (2021). Recent progress in chemical approaches for the development of novel neuraminidase inhibitors. RSC advances, 11(3), 1804-1840. Levels of Organization Cell Cells are the smallest units that still retain the characteristics of life, including complex organization, metabolic activity and reproductive behavior. 1665, Robert Hooke observed tiny, empty compartments of a thinly sliced piece of cork under microscope. He gave them the Latin name «cellulae» (small rooms) Theories in Science A scientific theory is an explanation of an aspect of the natural world that can be repeatedly tested and verified in accordance with the scientific method, using accepted protocols of observation, measurement, and evaluation of results. A theory is general enough to spin off many new, specific hypotheses that can be tested. Cell theory «Schleiden» 1838 - Plant tissues are made of cells. «Schwann» 1839 - Animal tissues are made of cells. All living organisms are composed of one or more cells. The cell is the basic living unit of organization for all organisms. All cells arise from preexisting cells. References & Thank You WHO – who.org cdc.gov Mahal, A., Duan, M., Zinad, D. S., Mohapatra, R. K., Obaidullah, A. J., Wei, X.,... & Zhu, Q. (2021). Recent progress in chemical approaches for the development of novel neuraminidase inhibitors. RSC advances, 11(3), 1804-1840. The Cell & Organelles Dr. Hilal Eren Gözel Faculty of Medicine, Department of Medical Biology & Genetics I. Okan University Contact Info [email protected] 1st Floor – Office #129 Objectives The secrets of Cell Hidden jewels: Organelles Clinical Correlations The Cell Cells are the basic structural and functional units of all multicellular organisms. Cells can be divided into two major compartments: the cytoplasm and the nucleus. In general, the cytoplasm is the part of the cell located outside the nucleus. The Cell – Cytoplasmic Matrix (Cytosol) Cytoplas. matrix + organelles + inclusions = Cytoplasm The matrix consists of a variety of solutes, including inorganic ions (Na+, K+, Ca2+) and organic molecules such as intermediate metabolites, carbohydrates, lipids, proteins, and RNAs. (pH: 7.2) The cytoplasmic matrix is the site of physiologic processes that are fundamental to the cell’s existence (protein synthesis, breakdown of nutrients). Inclusions are not usually surrounded by a plasma membrane. They consist of such diverse materials as crystals, pigment granules, lipids, glycogen, and other stored waste products. The Cell – Organelles An organelle is a specialized structures within a cell that perform a specific function. Like organs in the body!! Organelles are described as Membranous organelles with plasma membranes that separate the internal environment of the organelle from the cytoplasm, Nonmembranous organelles without plasma membranes. The Cell – Organelles The Membraneous organelles: The Nonmembraneous organelles: Plasma (Cell) membrane Ribosome Endoplasmic reticulum Microtubules and filaments Centriole Golgi apparatus Mitochondria Lysosome Endosomes, peroxisomes, transport vesicles The Cell – Nucleus The nucleus contains most of the cell’s genes and is usually the most conspicuous organelle - averaging about 5 μm in diameter. Components of Nucleus: Nuclear membrane Chromatin Nucleolus The Cell – Nucleus The nuclear envelope encloses the nucleus, separating it from the cytoplasm. The nuclear membrane is a double membrane; each membrane consists of a lipid bilayer. Nucleus The two membranes, each a lipid bilayer with associated proteins, are separated by a space of 20–40 nm. The envelope is perforated by pore structures that are about 100 nm in diameter. Nucleus At the lip of each pore, the inner and outer membranes of the nuclear envelope are continuous. An intricate protein structure called a pore complex lines each pore and plays an important role in the cell by regulating the entry and exit of proteins and RNAs, as well as large complexes of macromolecules. Nucleus The nuclear side of the envelope is lined by the nuclear lamina, a netlike array of protein filaments that maintains the shape of the nucleus by mechanically supporting the nuclear envelope. Nucleus 1 µm Nucleolus Chromatin Nuclear envelope: Inner membrane Outer membrane Nuclear pore Pore complex Rough ER Surface of nuclear envelope Ribosome 1 µm 0.25 µm Close-up of nuclear envelope Pore complexes (TEM) Nuclear lamina (TEM) Nucleus In the nucleus, DNA and proteins form genetic material called chromatin. Chromatin condenses to form discrete chromosomes. Some of the proteins help coil the DNA molecule of each chromosome, reducing its length and allowing it to fit into the nucleus (histon). A typical human cell has 46 chromosomes in its nucleus; the exceptions are the sex cells (eggs and sperm), which have only 23 chromosomes in humans. Nucleus Nucleus In most cells, chromatin does not have a homogeneous appearance; rather, clumps of densely staining chromatin are embedded in a more lightly staining background. The densely staining material is highly condensed chromatin called heterochromatin, and the lightly staining material (where most transcribed genes are located) is a dispersed form called euchromatin. Nucleus Heterochromatin Euchromatin Nucleolus The nucleolus (pl., nucleoli) is a small area within the nucleus that contains DNA in the form of transcriptionally active ribosomal RNA (rRNA) genes, RNA, and proteins. The nucleolus is the site of rRNA synthesis and contains regulatory cell-cycle proteins. It is the primary site of ribosomal production and assembly. Some cells contain more than one nucleolus. Ribosome Ribosomes are particles made of ribosomal RNA and proteins. Ribosomes carry out protein synthesis in two locations: In the cytosol (free ribosomes) On the outside of the endoplasmic reticulum or the nuclear envelope (bound ribosomes) Bound and free ribosomes are structurally identical, and ribosomes can alternate between the two roles. Ribosome Fig. 6-11 Cytosol Endoplasmic reticulum (ER) Free ribosomes Bound ribosomes Large subunit Small 0.5 µm subunit TEM showing ER and ribosomes Diagram of a ribosome Ribosome Most of the proteins made on free ribosomes function within the cytosol; examples are enzymes that catalyze the first steps of sugar breakdown (e.g. Hexokinase). Bound ribosomes generally make proteins that are destined for insertion into membranes, for packaging within certain organelles such as lysosomes, or for export from the cell (secretion). Cells that specialize in protein secretion—for instance, the cells of the pancreas that secrete digestive enzymes—frequently have a high proportion of bound ribosomes. Clinical Correlation: Ribosome Ribosomopathies are diseases caused by alterations in the structure or function of ribosomal components. Clinical features of the ribosomopathies can include bone marrow failure, developmental abnormalities, and increased risk of cancer. However, ribosomal dysfunction can cause a wide range of signs and symptoms, and presentation and severity can differ dramatically even among patients with the same diagnosis. Clinical Correlation: Ribosome Ref: Nakhoul, H., Ke, J., Zhou, X., Liao, W., Zeng, S. X., & Lu, H. (2014). Ribosomopathies: mechanisms of disease. Clinical Medicine Insights: Blood Disorders, 7, CMBD-S16952. The Endoplasmic Reticulum The endoplasmic reticulum (ER) (The word endoplasmic means “within the cytoplasm,” and reticulum is Latin for “little net.”) consists of a network of membranous tubules and sacs called cisternae. The ER membrane is continuous with the nuclear envelope There are two distinct regions of ER: Smooth ER, which lacks ribosomes Rough ER, with ribosomes studding its surface The Endoplasmic Reticulum Smooth ER Rough ER Nuclear envelope The Endoplasmic ER lumen Reticulum Cisternae Ribosomes Transitional ER Transport vesicle 200 nm Smooth ER Rough ER The Rough Endoplasmic Reticulum The rough ER; Has bound ribosomes, which produce secretory proteins which are mostly glycoproteins (proteins covalently bonded to carbohydrates) Distributes transport vesicles, proteins surrounded by membranes Is a membrane factory for the cell (adds membrane proteins and phospholipids) The Smooth Endoplasmic Reticulum The smooth ER; Synthesizes lipids (oils, phospholipids, and steroids), Metabolizes carbohydrates, Stores calcium (triggers muscle contraction), Detoxifies poison and drugs (adding hydroxyl groups to drug molecules, making them more soluble and easier to flush from the body). The Smooth Endoplasmic Reticulum The sedative phenobarbital and other barbiturates are examples of drugs metabolized in this manner by smooth ER in liver cells. In fact, barbiturates, alcohol, and many other drugs induce the proliferation of smooth ER and its associated detoxification enzymes, thus increasing the rate of detoxification. This, in turn, increases tolerance to the drugs, meaning that higher doses are required to achieve a particular effect, such as sedation. Also, because some of the detoxification enzymes have relatively broad action, the proliferation of smooth ER in response to one drug can increase tolerance to other drugs as well. Barbiturate abuse, for example, can decrease the effectiveness of certain antibiotics and other useful drugs. The Smooth Endoplasmic Reticulum Among the steroids produced by the smooth ER in animal cells are the sex hormones of vertebrates and the various steroid hormones (androgens, estrogens, and progestogens) secreted by the adrenal glands. The cells that synthesize and secrete these hormones—in the testes and ovaries, for example—are rich in smooth ER, a structural feature that fits the function of these cells. The Golgi Apparatus The Golgi apparatus consists of flattened membranous sacs called cisternae. Functions of the Golgi apparatus: Modifies/stores/sends products of the ER Manufactures certain macromolecules Sorts and packages materials into transport vesicles Add molecular identification tags as address in mailing labels. The Golgi Apparatus cis face ( receiving side of Golgi 0.1 µm apparatus) Cisternae trans face ( shipping side of Golgi TEM of Golgi apparatus apparatus) Lysosome Digestive compartment of cell. A lysosome is a membranous sac of hydrolytic enzymes that can digest macromolecules Lysosomal enzymes can hydrolyze proteins, fats, polysaccharides, and nucleic acids Lysosomal enzymes work best in the acidic environment found in lysosomes. If a lysosome breaks open or leaks its contents (enzymes) into the cell, does the cell be digested??? Nucleus 1 µm Some types of cell can engulf another cell by phagocytosis; this forms a food vacuole Lysosome Digestive A lysosome fuses with Lysosome enzymes the food vacuole and Plasma digests the molecules membrane Digestion Food vacuole (a) Phagocytosis Macrophages, a type of white blood cell that helps defend the body by engulfing and destroying bacteria and other invaders Vesicle containing 1 µm two damaged organelles Mitochondrion Lysosomes also use enzymes fragment to recycle the cellʼs own Peroxisome fragment organelles and macromolecules, a process called autophagy Lysosome Peroxisome Mitochondrion Digestion Vesicle (b) Autophagy Clinical Correlation: Lysosome Lysosomal Storage Diseases (LSDs) are a group of over 70 rare inherited metabolic disorders that result from defects in lysosomal function. The accumulation of substrates in excess in various organs' cells due to the defective functioning of lysosomes causes dysfunction of those organs. Clinical Relation: Lysosome Metachromatic leukodystrophy Sphingolipidoses – Type of lipid Mucopolysaccharidoses Type of sugar Mitochondrium Mitochondria change energy from one form to another! Mitochondria are the sites of cellular respiration, a metabolic process that generates ATP. Mitochondria; Have a double membrane Have proteins made by free ribosomes Contain their own DNA (mtDNA) Mitochondrium Mitochondria have a smooth outer membrane and an inner membrane folded into cristae. The inner membrane creates two compartments: intermembrane space and mitochondrial matrix. Some metabolic steps of cellular respiration are catalyzed in the mitochondrial matrix. Cristae present a large surface area for enzymes that synthesize ATP. Mitochondrium Intermembrane space Outer membrane Free ribosomes in the mitochondrial matrix Inner membrane Cristae Matrix 0.1 µm Mitochondrium Not just Energy!! Cytochrome c à Initiates apoptosis (programmed cell death!) Heat production! Thermogenin in brown adipose tissue. Ca2+ storage (secondary messenger) à coordinate processes such as neurotransmitter release in nerve cells and release of hormones in endocrine cells. Steroid synthesis Mature Erythrocytes do not have Mitochondria!! Mitochondrium Endosymbiont theory Mitochondria and chloroplasts display similarities with bacteria. Theory states that an early ancestor of eukaryotic cells engulfed an oxygen-using nonphotosynthetic prokaryotic cell. Eventually, the engulfed cell formed a relationship with the host cell in which it was enclosed, becoming an endosymbiont (a cell living within another cell). Endosymbiont theory Mitochondrium Endosymbiont theory – Evidences: The ancestral engulfed prokaryotes had two outer membranes, which became the double membranes of mitochondria and chloroplasts. Like prokaryotes, mitochondria and chloroplasts contain ribosomes, as well as circular DNA molecules attached to their inner membranes. The DNA in these organelles programs the synthesis of some of their own proteins, which are made on the ribosomes inside the organelles. Mitochondria and chloroplasts are autonomous (somewhat independent) organelles that grow and reproduce within the cell. Clinical Correlation: Mitochondrium MERRF syndrome (Myoclonic Epilepsy with Ragged Red Fibers) is a rare mitochondrial disease. Multisystem disorder characterized by myoclonus (often the first symptom) followed by generalized epilepsy, ataxia, weakness, and dementia. Symptoms usually first appear in childhood or adolescence after normal early development. The diagnosis is based on clinical features and a muscle biopsy finding of ragged red fibers. From: https://rarediseases.info.nih.gov/diseases/7144/myoclonic-epilepsy-with-ragged-red-fibers Clinical Relation: Mitochondrium Peroxisomes Peroxisomes are specialized metabolic compartments bounded by a single membrane Peroxisomes produce hydrogen peroxide and convert it to water Oxygen is used to break down different types of molecules (e.g. fatty acids) and send them to mitochondria for fuel (function in catabolism of long fatty chains.) Peroxisomes in the liver detoxify alcohol and other harmful compounds by transferring hydrogen from the poisons to oxygen. Chloroplast Peroxisome Mitochondrion 1 µm Clinical Correlation: Peroxisome The peroxisomal disorders represent a group of genetic diseases which there is an impairment in one or more peroxisomal functions. Zellweger syndrome belongs to a group of diseases called peroxisome biogenesis disorders (PBD). The diseases are caused by defects in any one of the PEX genes that are required for the normal formation and function of peroxisomes. Peroxisomes are required for normal brain development and function and the formation of myelin, the whitish substance that coats nerve fibers. They also are required for normal eye, liver, kidney, and bone functions. Clinical Correlation: Peroxisome The Zellweger spectrum disorders result from dysfunctional lipid metabolism, including the over-accumulation of very long-chain fatty acids and phytanic acid, and defects of bile acids and plasmalogens--specialized lipids found in cell membranes and myelin sheaths of nerve fibers. Centrosome – Centrioles In animal cells, microtubules grow out from a centrosome, a region that is often located near the nucleus and is considered a “microtubule-organizing center.” These microtubules function as compression-resisting girders of the cytoskeleton. Before an animal cell divides, the centrioles replicate. Although centrosomes with centrioles may help organize microtubule assembly in animal cells, they are not essential for this function in all eukaryotes (not present in fungi and plant cells). Within the centrosome is a pair of centrioles, each composed of nine sets of triplet microtubules arranged in a ring. can also be disassembled and their tubulin used to build mi- crotubules elsewhere in the cell. Because of the orientation Centrosome of tubulin dimers, the two ends of a microtubule are slightly different. One end can accumulate or release tubulin dimers at a much higher rate than the other, thus growing and shrinking significantly during cellular activities. (This is called the “plus end,” not because it can only add tubulin proteins but because it’s the end where both “on” and “off” Microtubule rates are much higher.) Centrosome – Microtubules shape and support the cell and also serve as tracks along which organelles equipped with motor proteins Centrioles Centrioles can move. In addition to the example in Figure 6.21, micro- 0.25 µm tubules guide secretory vesicles from the Golgi apparatus to the plasma membrane. Microtubules are also involved in the separation of chromosomes during cell division, which will be discussed in Chapter 12. Centrosomes and Centrioles In animal cells, microtubules grow out from a centrosome, a region that is often located near the nucleus and is considered a “microtubule-organizing center.” These microtubules function as compression-resisting girders of the cytoskeleton. Within the centrosome is a pair of centrioles, each composed of nine sets of triplet microtubules arranged in a ring (Figure 6.22). Before an animal cell divides, Longitudinal section Microtubules Cross section the centrioles replicate. Although centrosomes with centrioles of one centriole of the other centriole References & Thank You… Cytoskeleton, ECM, Intercellular Junctions Dr. Hilal Eren Gözel [email protected] Faculty of Medicine, Department of Medical Biology & Genetics Cytoskeleton Objectives Types of fibers and their functions ECM Intercellular Junctions Clinical Correlation Cytoskeleton The cytoskeleton is a network of fibers extending throughout the cytoplasm. M icrotubule M icrofilaments 0.25 µm Cytoskeleton The cytoskeleton helps to support the cell and maintain its shape It interacts with motor proteins to produce motility Inside the cell, vesicles can travel along “monorails” provided by the cytoskeleton The cytoskeleton provides anchorage for many organelles and even cytosolic enzyme molecules. Vesicle ATP Receptor for motor protein M otor protein M icrotubule (ATP powered) of cytoskeleton (a) M icrotubule Vesicles 0.25 µm (b) Cytoskeleton It is composed of three types of molecular structures: Microtubules are the thickest of the three components of the cytoskeleton Microfilaments, also called actin filaments, are the thinnest components Intermediate filaments are fibers with diameters in a middle range Cytoskeleton Video Link: https://www.youtube.com/watch?v=YTv9ItGd050 Microtubule Microtubules have a crucial organizing role in all eukaryotic cells. These long and relatively stiff hollow tubes of protein can rapidly disassemble in one location and reassemble in another. In a typical animal cell, microtubules grow out from a small structure near the center of the cell called the centrosome. Microtubules Extending out toward the cell periphery, they create a system of tracks within the cell, along which vesicles, organelles, and other cell components can be transported. They are mainly responsible for transporting and positioning membrane-enclosed organelles within the cell and for guiding the intracellular transport of various cytosolic macromolecules. When a cell enters mitosis, the cytoplasmic microtubules disassemble and then reassemble into an intricate structure called the mitotic spindle. The mitotic spindle provides the machinery that will segregate the chromosomes equally into the two daughter cells just before a cell divides. Microtubules Microtubules can also form stable structures, such as rhythmically beating cilia and flagella. 10 µm Column of tubulin dimers 25 nm a b Tubulin dimer Cytoskeleton – Micro filaments Microfilaments are thin, solid, and flexible rods about 7 nm in diameter, built as a twisted double chain of actin subunits. The structural role of microfilaments is to bear tension, resisting pulling forces within the cell. Actin filaments are linked by actin-binding proteins into a meshwork that supports the plasma membrane and gives it mechanical strength. Thus, they help support the cell’s shape. (Stable structure) They are essential for many of the cell’s movements (Temporary structure). In human red blood cells, a simple and regular network of fibrous proteins (including actin and spectrin filaments) attaches to the plasma membrane, providing the support necessary for the cells to maintain their simple discoid shape. Bundles of microfilaments make up the core of microvilli of intestinal cells. Cytoskeleton – Micro filaments Microfilaments that function in cellular motility contain the protein myosin in addition to actin. In muscle cells, thousands of actin filaments are arranged parallel to one another. Thicker filaments composed of myosin interdigitate with the thinner actin fibers. Cytoskeleton – Micro filaments Localized contraction brought about by actin and myosin also drives amoeboid movement. Pseudopodia (cellular extensions) extend and contract through the reversible assembly and contraction of actin subunits into microfilaments. Cytoskeleton – Micro filaments Cytoplasmic streaming is a circular flow of cytoplasm within cells. This streaming speeds distribution of materials within the cell. 10 µm Actin subunit 7 nm Intermediate Filaments Intermediate filaments have great tensile strength, and their main function is to enable cells to withstand the mechanical stress that occurs when cells are stretched. Intermediate filaments are the toughest and most durable of the cytoskeletal filaments (anchorage!) There they are often anchored to the plasma membrane at cell– cell junctions called desmosomes where the plasma membrane is connected to that of another cell. Inside nucleus, they form a meshwork called the nuclear lamina, which underlies and strengthens the nuclear envelope. Intermediate Filaments An intermediate filament is like a rope in which many long strands are twisted together to provide tensile strength. Intermediate Filaments Intermediate filaments are particularly prominent in the cytoplasm of cells that are subject to mechanical stress. They are also abundant in muscle cells and in epithelial cells such as those of the skin. In all these cells, intermediate filaments distribute the effect of locally applied forces, thereby keeping cells and their membranes from tearing in response to mechanical shear. Intermediate Filaments Intermediate filaments can be grouped into four classes: (1) keratin filaments in epithelial cells; (2) vimentin and vimentin-related filaments in connective-tissue cells, muscle cells, and supporting cells of the nervous system (glial cells); (3) neurofilaments in nerve cells; and (4) nuclear lamins, which strengthen the nuclear envelope. The first three filament types are found in the cytoplasm, whereas the fourth is found in the nucleus. Clinical Correlation – Epidermolysis bullosa simplex EBS is an inherited genetic (Autosomal dominant) disorder resulting from mutations in the genes encoding keratin 5 or keratin 14. These genetic mutations prevent the proper formation of keratin structures in the skin’s epidermis which cause the skin to become very fragile. Any trauma or friction to the skin can cause painful blisters and bleedings. Blisters especially occurs on the hands and soles of the feet! Thickened skin that may be scarred or change colour over time. 5 µm Keratin proteins Fibrous subunit (keratins coiled together) 8–12 nm Borders of the Cell and Beyond Most cells synthesize and secrete materials that are external to the plasma membrane These extracellular structures include: The extracellular matrix (ECM) of animal cells Intercellular junctions Extracellular Matrix (ECM) Since animal cells have no cell wall, ECM provides biochemical and structural support to surrounding cells. The composition of ECM varies between multicellular structures; however, cell adhesion, cell-to-cell communication and differentiation are common functions of the ECM. The ECM is made up of glycoproteins such as collagen, proteoglycans, and fibronectin ECM proteins bind to receptor proteins in the plasma membrane called integrins ECM Intercellular Junctions Intercellular Junctions Neighboring cells in tissues, organs, or organ systems often adhere, interact, and communicate through direct physical contact Intercellular junctions facilitate this contact There are several types of intercellular junctions Tight junctions Desmosomes Gap junctions Intercellular Junctions At tight junctions, membranes of neighboring cells are pressed together, preventing leakage of extracellular fluid Intercellular Junctions Desmosomes (anchoring junctions) fasten cells together into strong sheets Intercellular Junctions Gap junctions (communicating junctions) provide cytoplasmic channels between adjacent cells References &Thank You CELL MEMBRANE & MEMBRANE TRANSPORT THIS LECTURE Cell Membrane – Structure, Function Types of Transport CELL MEMBRANE The plasma membrane is the edge of life, the boundary that separates the living cell from its surroundings (8 nm thick). Plasma membrane exhibits selective permeability (it allows some substances to cross it more easily than others). A eukaryotic plasma membrane protein plays a crucial role in nerve cell signaling. Lipids and proteins are the staple ingredients of membranes, although carbohydrates are also important. The most abundant lipids in most membranes are phospholipids. A phospholipid is an amphipathic molecule, meaning it has both a hydrophilic region and a hydrophobic region. WATER Hydrophilic head Hydrophobic tail WATER CELL MEMBRANE Membrane proteins reside in the phospholipid bilayer with their hydrophilic regions on the outside. This molecular arrangement would maximize contact of hydrophilic regions of proteins and phospholipids with water in the cytosol and extracellular fluid, while providing their hydrophobic parts with a nonaqueous environment. In this fluid mosaic model, the membrane is a mosaic of protein molecules bobbing in a fluid bilayer of phospholipids. The fluid mosaic model states that a membrane is a fluid structure with a “mosaic” of various proteins embedded in it. Phospholipid bilayer Hydrophobic regions Hydrophilic of protein regions of protein CELL MEMBRANE The Fluidity of Membranes Membranes are not static sheets of molecules locked rigidly in place. A membrane is held together primarily by hydrophobic interactions, which are much weaker than covalent bonds. Most of the lipids and some of the proteins can shift about laterally. It is quite rare, however, for a molecule to flip-flop transversely across the membrane, switching from one phospholipid layer to the other. Lateral movement Flip-flop (~107 times per second) (~ once per month) (a) Movement of phospholipids Fluid Viscous Unsaturated hydrocarbon Saturated hydro- tails with kinks carbon tails (b) Membrane fluidity Cholesterol (c) Cholesterol within the animal cell membrane CELL MEMBRANE The Fluidity of Membranes Some membrane proteins seem to move in a highly directed manner, perhaps driven along cytoskeletal fibers by motor proteins connected to the membrane proteins’ cytoplasmic regions. However, many other membrane proteins seem to be held immobile by their attachment to the cytoskeleton or to the extracellular matrix. CELL MEMBRANE The Fluidity of Membranes A membrane remains fluid as temperature decreases until finally the phospholipids settle into a closely packed arrangement and the membrane solidifies, much as bacon grease forms lard when it cools. The temperature at which a membrane solidifies depends on the types of lipids it is made of. The membrane remains fluid to a lower temperature if it is rich in phospholipids with unsaturated hydrocarbon tails. Because of kinks in the tails where double bonds are located, unsaturated hydrocarbon tails cannot pack together as closely as saturated hydrocarbon tails, and this makes the membrane more fluid. Fluid Viscous Unsaturated hydrocarbon Saturated hydro- tails with kinks carbon tails (b) Membrane fluidity CELL MEMBRANE The steroid cholesterol has different effects on membrane fluidity at different temperatures. At warm temperatures (such as 37°C), cholesterol prevents the movement of phospholipids. At cool temperatures, it maintains fluidity by preventing tight packing. Cholesterol can be thought of as a “fluidity buffer” for the membrane, resisting changes in membrane fluidity that can be caused by changes in temperature. Cholesterol High Temperature Low Temperature (c) Cholesterol within the animal cell membrane CELL MEMBRANE Membrane Proteins A membrane is a collage of different proteins embedded in the fluid matrix of the lipid bilayer. Proteins determine most of the membrane’s specific functions. There are two major populations of membrane proteins: integral proteins and peripheral proteins. CELL MEMBRANE Membrane Proteins Peripheral proteins are not embedded in the lipid bilayer at all; they are appendages loosely bound to the surface of the membrane, often to exposed parts of integral proteins Integral proteins penetrate the hydrophobic interior of the lipid bilayer. CELL MEMBRANE Membrane Proteins The majority of integral proteins are transmembrane proteins, which span the membrane; other integral proteins extend only partway into the hydrophobic interior. The hydrophobic regions of an integral protein consist of one or more stretches of nonpolar amino acids usually coiled into α helices. The hydrophilic parts of the molecule are exposed to the aqueous solutions on either side of the membrane. Some proteins also have a hydrophilic channel through their center that allows passage of hydrophilic substances. N-terminus EXTRACELLULAR SIDE C-terminus CYTOPLASMIC Helix SIDE Six major functions of membrane proteins: Transport Enzymatic activity Signal transduction Cell-cell recognition Intercellular joining Attachment to the cytoskeleton and extracellular matrix (ECM) Major functions of membrane proteins: Transport: A protein that spans the membrane may provide a hydrophilic channel across the membrane that is selective for a particular solute. Other transport proteins shuttle a substance from one side to the other by changing shape. Some of ATP these proteins hydrolyze ATP as an energy source to actively pump substances across the membrane. Enzymes Major functions of membrane proteins: Enzymatic activity: A protein built into the membrane may be an enzyme with its active site exposed to substances in the adjacent solution. In some cases, several enzymes in a membrane are organized as a team that carries out sequential steps of a metabolic pathway. Signaling molecule Major functions of membrane Receptor proteins: Signal transduction: A membrane protein (receptor) may have a binding site with a specific shape that fits the shape of a chemical messenger, such as a hormone. The external messenger (signaling molecule) may cause the protein to change shape, allowing it to relay the message to the inside of the cell, usually by binding to a cytoplasmic Signal transduction protein. Major functions of membrane proteins: Cell-cell recognition: Some glycoproteins serve as identification tags that are specifically recognized by Glyco- membrane proteins of other cells. This protein type of cell-cell binding is usually short-lived compared to intercellular joining. Major functions of membrane proteins: Intercellular joining: Membrane proteins of adjacent cells may hook together in various kinds of junctions, such as gap junctions or tight junctions. This type of binding is more long- lasting than the cell-cell recognition. Major functions of membrane proteins: Attachment to the cytoskeleton and extracellular matrix (ECM): Microfilaments or other elements of the cytoskeleton may be noncovalently bound to membrane proteins (integrins), a function that helps maintain cell shape and stabilizes the location of certain membrane proteins. Proteins that can bind to ECM molecules can coordinate extracellular and intracellular changes. Signaling molecule Enzymes Receptor ATP Signal transduction (a) Transport (b) Enzymatic activity (c) Signal transduction Glyco- protein (d) Cell-cell recognition (e) Intercellular joining (f) Attachment to the cytoskeleton and extracellular matrix (ECM) CELL MEMBRANE The Role of Membrane Carbohydrates in Cell-Cell Recognition Cells recognize each other by binding to surface molecules, often carbohydrates, on the plasma membrane. Membrane carbohydrates may be covalently bonded to lipids (forming glycolipids) or more commonly to proteins (forming glycoproteins) Carbohydrates on the external side of the plasma membrane vary among species, individuals, and even cell types in an individual e.g. Blood types! CELL MEMBRANE The Permeability of the Lipid Bilayer A cell must exchange materials with its surroundings, a process controlled by the plasma membrane. Plasma membranes are selectively permeable, regulating the cell’s molecular traffic. Hydrophobic (nonpolar) molecules, such as hydrocarbons, can dissolve in the lipid bilayer and pass through the membrane rapidly. Polar molecules, such as sugars, do not cross the membrane easily. CELL MEMBRANE The Permeability of the Lipid Bilayer – Transport Proteins Transport proteins allow passage of hydrophilic substances across the membrane. Some transport proteins, called channel proteins, have a hydrophilic channel that certain molecules or ions can use as a tunnel. Channel proteins called aquaporins facilitate the passage of water. CELL MEMBRANE The Permeability of the Lipid Bilayer – Transport Proteins Other transport proteins, called carrier proteins, bind to molecules and change shape to shuttle them across the membrane. ATP A transport protein is specific for the substance it moves. Channel Carrier CELL MEMBRANE Types of Transport – Passive Transport Diffusion is the tendency for molecules to spread out evenly into the available space. Although each molecule moves randomly, diffusion of a population of molecules may exhibit a net movement in one direction. At dynamic equilibrium, as many molecules cross one way as cross in the other direction. Molecules of dye Membrane (cross section) WATER Net diffusion Net diffusion Equilibrium (a) Diffusion of one solute CELL MEMBRANE Types of Transport – Passive Transport A substance will diffuse from where it is more concentrated to where it is less concentrated. Substances diffuse down their concentration gradient, the difference in concentration of a substance from one area to another. No work must be done to move substances down the concentration gradient. The diffusion of a substance across a biological membrane is passive transport because it requires no energy from the cell to make it happen. Net diffusion Net diffusion Equilibrium Net diffusion Net diffusion Equilibrium (b) Diffusion of two solutes CELL MEMBRANE Types of Transport – Passive Transport Osmosis is the diffusion of water across a selectively permeable membrane. Water diffuses across a membrane from the region of lower solute concentration to the region of higher solute concentration. Lower Higher Same concentration concentration concentration of sugar of solute (sugar) of sugar H2O Selectively permeable membrane Osmosis CELL MEMBRANE Types of Transport – Passive Transport Water balance in Cells without wall Tonicity is the ability of a solution to cause a cell to gain or lose water. Isotonic solution: Solute concentration is the same as that inside the cell; no net water movement across the plasma membrane. Hypertonic solution: Solute concentration is greater than that inside the cell; cell loses water. Hypotonic solution: Solute concentration is less than that inside the cell; cell gains water. Hypotonic solution Isotonic solution Hypertonic solution H2O H2O H2O H2O Erythrocyte swell Lysed Normal Shriveled CELL MEMBRANE Types of Transport – Passive Transport In facilitated diffusion, transport proteins speed the passive movement of molecules across the plasma membrane. Channel proteins provide corridors that allow a specific molecule or ion to cross the membrane Channel proteins include Aquaporins, for facilitated diffusion of water Ion channels that open or close in response to a stimulus (gated channels) EXTRACELLULAR FLUID Channel protein Solute CYTOPLASM (a) A channel protein Carrier proteins undergo a subtle change in shape that translocates the solute-binding site across the membrane Carrier protein Solute (b) A carrier protein CELL MEMBRANE Types of Transport – Passive Transport Facilitated diffusion is still passive because the solute moves down its concentration gradient. Some transport proteins, however, can move solutes against their concentration gradients. CELL MEMBRANE Types of Transport – Active Transport Active transport moves substances against their concentration gradient. Active transport requires energy, usually in the form of ATP. Active transport is performed by specific proteins embedded in the membranes. Active transport allows cells to maintain concentration gradients that differ from their surroundings. The sodium-potassium pump is one type of active transport system. EXTRACELLULAR Na+ [Na+] high FLUID Na+ [K+] low Na+ Na+ Na+ Na+ Na+ Na+ [Na+] low ATP Na+ P [K+] high P CYTOPLASM ADP 1 Cytoplasmic Na+ binds to 2 Na+ binding stimulates 3 Phosphorylation causes the phosphorylation by ATP. protein to change its shape. Na+ the sodium-potassium pump. is expelled to the outside. P P 6 K+ is released, and the 5 Loss of the phosphate restores 4 K+ binds on the extracellular side and cycle repeats. the protein’s original shape. triggers release of the phosphate group. Passive transport Active transport ATP Diffusion Facilitated diffusion CELL MEMBRANE Types of Transport – Bulk Transport Small molecules and water enter or leave the cell through the lipid bilayer or by transport proteins. Large molecules, such as polysaccharides and proteins, cross the membrane in bulk via vesicles. Bulk transport requires energy. CELL MEMBRANE Types of Transport – Bulk Transport – Exocytosis In exocytosis, transport vesicles migrate to the membrane, fuse with it, and release their contents. Many secretory cells use exocytosis to export their products. ER Transmembrane glycoproteins Secretory protein Glycolipid Golgi apparatus Vesicle Exocytosis Plasma membrane: Cytoplasmic face Extracellular face Transmembrane Secreted glycoprotein protein Membrane glycolipid CELL MEMBRANE Types of Transport – Bulk Transport – Endocytosis In endocytosis, the cell takes in macromolecules by forming vesicles from the plasma membrane Endocytosis is a reversal of exocytosis, involving different proteins There are three types of endocytosis: Phagocytosis (“cellular eating”) Pinocytosis (“cellular drinking”) Receptor-mediated endocytosis In phagocytosis a cell engulfs a particle in a vacuole The vacuole fuses with a lysosome to digest the particle PHAGOCYTOSIS EXTRACELLULAR CYTOPLASM 1 µm FLUID Pseudopodium Pseudopodium of amoeba “Food” or other particle Bacterium Food vacuole Food vacuole An amoeba engulfing a bacterium via phagocytosis (TEM) In pinocytosis, molecules are taken up when extracellular fluid is “gulped” into tiny vesicles PINOCYTOSIS 0.5 µm Plasma membrane Pinocytosis vesicles forming (arrows) in a cell lining a small blood vessel (TEM) Vesicle RECEPTOR-MEDIATED ENDOCYTOSIS Coat protein Receptor Coated vesicle Coated pit Ligand In receptor-mediated endocytosis, binding of ligands to receptors triggers vesicle formation A ligand is any molecule that binds specifically to a receptor site of another molecule References & Thank You! Introduction to Nucleic Acids Dr. Hilal Eren Gözel I. Okan University DNA is the genetic material Hereditary information is encoded in the chemical language of DNA and reproduced in all the cells of our body. It is the DNA program that directs the development of many different types of traits. How is the DNA structure discovered? By the 1920s, scientists generally agreed that genes reside on chromosomes, and they knew that chromosomes are composed of both DNA and proteins. Which one is the hereditary molecule? Griffith Experiment!! In 1928, Fred Griffith was studying Streptococcus pneumoniae (pneumococcus), a bacterium that causes pneumonia. As antibiotics had not yet been discovered, infection with this organism was usually fatal. Griffith Experiment – Messages from the dead Pneumococci come in two forms: a pathogenic form that causes a lethal infection when injected into animals, and a harmless form that is easily conquered by the animal’s immune system and does not produce an infection. Griffith Experiment – Messages from the dead When the pathogenic pneumococci that had been killed by heating were no longer able to cause infection! Griffith Experiment – Messages from the dead The surprise came when Griffith injected both heat-killed pathogenic bacteria and live harmless bacteria into the same mouse. This combination proved that: not only did the animals die of pneumonia, but Griffith found that their blood was teeming with live bacteria of the pathogenic form. He mentioned that there is a ‘transforming principle’ that causes that phenomenon. Transformation is the genetic alteration of a cell resulting from the direct uptake and incorporation of exogenous genetic material from its surroundings through the cell membrane(s). 15-year-old Experiment - Avery, MacLeod, and McCarty Experiment What is this transforming principle???? The first strong evidence that genes are made of DNA: The American bacteriologist Oswald Avery and his colleagues Colin MacLeod and Maclyn McCarty, following up on Griffith’s work, discovered that the harmless pneumococcus could be transformed into a pathogenic strain in a culture tube by exposing it to an extract prepared from the pathogenic strain (1944). They successfully purified the “transforming principle” from this soluble extract and to demonstrate that the active ingredient was DNA. How is the DNA structure discovered? Erwin Chargaff analyzed the base composition of DNA from a number of different organisms In 1947, Chargaff reported That DNA composition (number of different nucleotides) varies from one species to the next (evidence of molecular diversity among species) That the number of adenines approximately equaled the number of thymines, and the number of guanines approximately equaled the number of cytosines. The Search for the Genetic Material: Scientific Inquiry Chargaff Rules: 1. The base composition varies between species, 2. Within each species, the number of A and T bases are equal, and the number of G and C bases are equal. How is the DNA structure discovered? Maurice Wilkins and Rosalind Franklin were using a technique called X-ray crystallography to study molecular structure In May 1952, Raymond Gosling a graduate student working under the supervision of Rosalind Franklin took an X-ray diffraction image, labeled as "Photo 51" (a) Rosalind Franklin (b) Franklin’s X-ray diffraction Figure 16.6 a, b Photograph of DNA (6) Antiparallel How is the DNA structure discovered? Franklin had concluded that DNA was composed of two antiparallel sugar-phosphate backbones, with the nitrogenous bases paired in the molecule’s interior The nitrogenous bases are paired in specific combinations: adenine with thymine, and cytosine with guanine (a) Rosalind Franklin (b) Franklin’s X-ray diffraction Figure 16.6 a, b Photograph of DNA How is the DNA structure discovered? When James Watson came to Cambridge University to visit Francis Crick, he saw this photograph. Watson was familiar with the type of X-ray diffraction pattern that helical molecules produce, and an examination of the photo confirmed that DNA was helical in shape and made up from two helices. (a) Rosalind Franklin (b) Franklin’s X-ray diffraction Figure 16.6 a, b Photograph of DNA How is the DNA structure discovered? In 1953, James Watson and Francis Crick shook the world with an elegant double-helical model for the structure of deoxyribonucleic acid, or DNA https://www.nature.com/articles/171737a0 re 16.1 Components of Nucleic Acids Nucleic acids are polymers called polynucleotides Each polynucleotide is made of monomers called nucleotides Each nucleotide consists of a nitrogenous base, a pentose sugar, and a phosphate group The portion of a nucleotide without the phosphate group is called a nucleoside (nitrogenous base + pentose sugar) 5' end 5'C 3'C Nucleoside Nitrogenous base 5'C Phosphate 3'C group Sugar 5'C (pentose) 3'C (b) Nucleotide 3' end (a) Polynucleotide, or nucleic acid Nucleotide Monomers Nucleoside = nitrogenous base + sugar There are two families of nitrogenous bases: Pyrimidines (cytosine, thymine, and uracil) have a single six-membered ring Purines (adenine and guanine) have a six-membered ring fused to a five-membered ring In DNA, the sugar is deoxyribose; in RNA, the sugar is ribose Nucleotide = nucleoside + phosphate group Nitrogenous bases Pyrimidines Cytosine (C) Thymine (T, in DNA) Uracil (U, in RNA) Purines Adenine (A) Guanine (G) (c) Nucleoside components: nitrogenous bases The DNA Double Helix A DNA molecule has two polynucleotides spiraling around an imaginary axis, forming a double helix In the DNA double helix, the two backbones run in opposite 5 → 3 directions from each other, an arrangement referred to as antiparallel One DNA molecule includes many genes The nitrogenous bases in DNA pair up and form hydrogen bonds: adenine (A) always with thymine (T), and guanine (G) always with cytosine (C) 5’ end O OH P Hydrogen bond –O 3’ end O OH O T A O O CH2 O P –O O O– O P O H2C O O Phosphodiester bond G C O O CH2 O P –O O O– O P O H2C O O C G O O CH2 O P O –O O– O P O H2C O O A T O CH2 OH 3’ end O O– P O O (b) Partial chemical structure Figure 16.7b 5’ end Nucleic Acids The two types of nucleic acids, deoxyribonucleic acid (DNA) and ribonucleic acid (RNA), enable living organisms to reproduce their complex components from one generation to the next. DNA provides directions for its own replication. DNA also directs RNA synthesis and, through RNA, controls protein synthesis. Nucleic Acids In a eukaryotic cell, ribosomes are in the cytoplasm, but DNA resides in the nucleus. Each gene along a DNA molecule directs synthesis of a type of RNA called messenger RNA (mRNA). Messenger RNA conveys genetic instructions for building proteins from the nucleus to the cytoplasm. The flow of genetic information: DNA RNA protein DNA 1 Synthesis of mRNA in the nucleus mRNA NUCLEUS CYTOPLASM mRNA 2 Movement of mRNA into cytoplasm Ribosome via nuclear pore 3 Synthesis of protein Amino Polypeptide acids RNA The primary structure of RNA is generally similar to that of DNA with two exceptions: the sugar component of RNA, ribose, has a hydroxyl group at the 2 position and thymine in DNA is replaced by uracil in RNA. Sugars Deoxyribose (in DNA) Ribose (in RNA) Nitrogenous bases Pyrimidines Cytosine (C) Thymine (T, in DNA) Uracil (U, in RNA) Purines Adenine (A) Guanine (G) (c) Nucleoside components: nitrogenous bases RNA Unlike DNA, most cellular RNAs are single-stranded and exhibit a variety of conformations. Differences in the sizes and conformations of the various types of RNA permit them to carry out specific functions in a cell. The simplest secondary structures in single-stranded RNAs are formed by pairing of complementary bases. “Hairpins” are formed by pairing of bases within ≈5–10 nucleotides of each other, and “stem-loops” by pairing of bases that are separated by >10 to several hundred nucleotides. These simple folds can cooperate to form more complicated tertiary structures, one of which is termed a “pseudoknot.” RNA RNA The folded domains of RNA molecules may have catalytic capacities. Such catalytic RNAs are called ribozymes. Although ribozymes usually are associated with proteins that stabilize the ribozyme structure, it is the RNA that acts as a catalyst. Some ribozymes can catalyze splicing, a remarkable process in which an internal RNA sequence is cut and removed, and the two resulting chains then ligated. RNA World Hypothesis The RNA world is a hypothetical stage in the evolutionary history of life on Earth, in which self- replicating RNA molecules proliferated before the evolution of DNA and proteins. Types of RNA The vast majority of genes carried in a cell’s DNA specify the amino acid sequences of proteins. Some genes code for RNA molecules! Important RNAs for Transcription: Types of RNA *Telomeres are transcribed generating long non-coding RNAs known as TERRA! DNA and RNA References Chapter 417 and 351 DNA and DNA Replication Dr. Hilal Eren Gözel I. Okan University DNA is the genetic material Hereditary information is encoded in the chemical language of DNA and reproduced in all the cells of our body. It is the DNA program that directs the development of many different types of traits. 5’ end O OH P Hydrogen bond –O 3’ end O OH O T A O O CH2 O P –O O O– O P O H2C O O Phosphodiester bond G C O O CH2 O P –O O O– O P O H2C O O C G O O CH2 O P O –O O– O P O H2C O O A T O CH2 OH 3’ end O O– P O O (b) Partial chemical structure Figure 16.7b 5’ end DNA Replication DNA replication in most eukaryotic cells occurs only during a specific part of the cell-division cycle, called the DNA synthesis phase or S phase. Since the two strands of DNA are complementary Each strand acts as a template for building a new strand in replication In DNA replication The parent molecule unwinds, and two new daughter strands are built based on base-pairing rules DNA Replication What is the purpose of this? First Second Parent cell replication replication Conservative model. The two parental strands reassociate after acting as templates for new strands, thus restoring the parental double helix. Semiconservative model. The two strands of the parental molecule DNA Replication separate, and each functions as a template for synthesis of a new, comple- mentary strand. Dispersive model. Each strand of both daughter mol- ecules contains a mixture of **Advanced Reading: old and newly synthesized Meselson–Stahl experiment DNA. Figure 16.10 a–c DNA Replication DNA replication is semiconservative Each of the two new daughter molecules will have one old strand, derived from the parent molecule, and one newly made strand Origins of Replication The replication of a DNA molecule begins at special sites called origins of replication, where the two strands are separated A eukaryotic chromosome may have hundreds or even thousands of replication origins However, bacterial genome is circular and has a single origin. Origins of Replication Initiator proteins that initiate DNA replication recognize this sequence and attach to the DNA, break the hydrogen bonds, separating the two strands and opening up a replication “bubble.” By binding and opening up the double helix, initiator proteins also attract a group of proteins that carry out DNA replication. These proteins form a replication machine, in which each protein carries out a specific function. Replication of DNA then proceeds in both directions until the entire molecule is copied. Origins of Replication Origins of Replication At each end of a replication bubble is a replication fork, a Y-shaped region where the parental strands of DNA are being unwound. At each fork, a replication machine moves along the DNA, opening up the two strands of the double helix and using each strand as a template to make a new daughter strand. Origins of Replication Origin of replication Parental (template) strand 0.25 µm Daughter (new) strand 1 Replication begins at specific sites where the two parental strands separate and form replication bubbles. Bubble Replication fork 2 The bubbles expand laterally, as DNA replication proceeds in both directions. 3 Eventually, the replication bubbles fuse, and synthesis of the daughter strands is complete. Two daughter DNA molecules (a) In eukaryotes, DNA replication begins at many sites along the giant (b) In this micrograph, three replication DNA molecule of each chromosome. bubbles are visible along the DNA of Figure 16.12 a, b a cultured Chinese hamster cell (TEM). Problem #1 How to unzip entire DNA double helix and prevent them re-binding? DNA Replication Two types of replication proteins—DNA helicases and single-strand DNA-binding proteins—cooperate to carry out this task. For DNA replication to occur, the double helix must be unzipped ahead of the replication fork so that the incoming nucleoside triphosphates can form base pairs with each template strand. Helicases are enzymes that unzip the double helix at the replication forks, separating the two parental strands and making them available as template strands. DNA Replication The helicase sits at the very front of the replication machine where it uses the energy of ATP hydrolysis to propel itself forward, prying apart the double helix as it speeds along the DNA. DNA Replication Single-strand DNA-binding proteins bind tightly and cooperatively to the single-stranded DNA exposed by the helicase without covering the bases, transiently preventing the strands from re-forming base pairs and keeping them in an elongated form so that they can serve as efficient templates. Replication Machinery An additional replication protein, called a sliding clamp, keeps DNA polymerase firmly attached to the template while it is synthesizing new strands of DNA. Left on their own, most DNA polymerase molecules will synthesize only a short string of nucleotides before falling off the DNA template strand. The sliding clamp forms a ring around the newly formed DNA double helix and, by tightly gripping the polymerase, allows the enzyme to move along the template strand without falling off as it synthesizes new DNA. Replication Machinery Problem #2 Unzipping a helical molecule creates a tension and may break it!!! DNA Replication After the parental strands separate, single-strand binding proteins (SSBP) bind to the unpaired DNA strands, keeping them from re-pairing. Topoisomerase helps relieve this strain by breaking, swiveling, and rejoining DNA strands. These enzymes produce transient nicks in the DNA backbone, which temporarily release the tension; they then reseal the nick before falling off the DNA. DNA Replication https://www.youtube.com/watch?v=EYGrElVyHnU - Video 1 Problem #3 DNA polymerase cannot bind to the template by itself! Initiation of Replication The enzymes that synthesize DNA cannot initiate the synthesis of a polynucleotide; they can only add nucleotides to the end of an already existing chain that is base-paired with the template strand. The initial nucleotide chain that is produced during DNA synthesis is actually a short stretch of RNA, not DNA. This RNA chain is called a primer and is synthesized by the enzyme primase. Initiation of Replication Primase starts a complementary RNA chain from a single RNA nucleotide, adding RNA nucleotides one at a time, using the parental DNA strand as a template. The completed primer, generally 5–10 nucleotides long, is thus base-paired to the template strand. The new DNA strand will start from the 3 end of the RNA primer. Elongating a New DNA Strand Elongation of new DNA at a replication fork is catalyzed by enzymes called DNA polymerases, which add nucleotides to the 3 end of a growing strand. (Heart of replication machine!) New strand Template strand 5’ end 3’ end 5’ end 3’ end Sugar A T A T Phosphate Base C G C G G C G C A T A P P OH C Pyrophosphate 3 end C OH 2 P Figure 16.13 5’ end 5’ end Elongating a New DNA Strand The polymerization reaction involves the formation of a phosphodiester bond between the 3ʹ end of the growing DNA chain and the 5ʹ-phosphate group of the incoming nucleotide, which enters the reaction as a deoxyribonucleoside triphosphate. The energy for polymerization is provided by the incoming deoxyribonucleoside triphosphate itself: hydrolysis of one of its high-energy phosphate bonds fuels the reaction that links the nucleotide monomer to the chain, releasing pyrophosphate. Elongating a New DNA Strand deoxyribonucleoside triphosphate Elongating a New DNA Strand For replication in Eukaryotes, up to 15 different DNA polymerases discovered so far. Eukaryotic DNA pols are designated with greek letters (DNA pols α, β, γ, δ, ε, ζ, η, θ, ι, κ, λ, μ, ν), with the exception of terminal transferase and Rev1. Bacterial DNA pols are designated with roman numerals (DNA pol I, II, III, IV, and V). Antiparallel Elongation In eukaryotes, DNA replication requires three DNA pols: DNA pol α initiates the DNA Replication, DNA pol ε is required for the leading strand, and DNA pol α and DNA pol δ is required for replication of the lagging strand. Rest of the polymerases have important roles in DNA repair. Problem #4 Antiparallel Elongation creates fragments on the lagging strand! How does the antiparallel structure of the double helix affect replication? https://www.youtube.com/watch?v=TNKWgcFPHqw Video 2 Antiparallel Elongation DNA polymerases add nucleotides only to the free 3end of a growing strand. Along one template strand of DNA, the leading strand DNA polymerase can synthesize a complementary strand continuously, moving toward the replication fork. Antiparallel Elongation Antiparallel Elongation – Video 2