Illumination PDF
Document Details
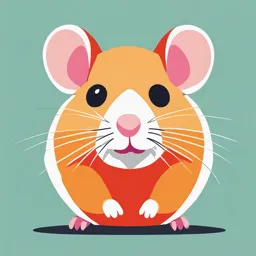
Uploaded by WellInformedPersonification
Tags
Summary
This document is a textbook on the topic of illumination. It introduces various important concepts in the field and explains different lighting technologies. It also details calculations and methods related to illumination and light.
Full Transcript
EEE-202 Module – 4: Illumination Introduction: Advantages of artificial lighting produced electrically: o Cleanliness o Ease of control o Reliability o Steady output o Low cost...
EEE-202 Module – 4: Illumination Introduction: Advantages of artificial lighting produced electrically: o Cleanliness o Ease of control o Reliability o Steady output o Low cost o Aesthetic aspects Significance of artificial lighting produced electrically: o Increasing production o Reducing workers’ fatigue, protecting their health, eyes, and nervous system o Reducing accidents Nature of light o Light is a form of radiant energy. o Various forms of incandescent bodies are the sources of light and the light emitted by such bodies depends upon the temperature of bodies. o Visible light can have a wavelength between 4000 Å and 7500 Å. o Variation of colour: o Figure: Wavelength and colour Illumination: Illumination differs from light very much, though generally these terms are used more or less synonymously. Strictly speaking, light is the cause and illumination is the result of that light on surfaces on which it falls. Thus, the illumination makes the surface look more or less bright with a certain colour and it is this brightness and colour that the eye sees and interprets as something useful, or pleasant or otherwise. Important terms: Light: It is defined as the radiant energy from a hot body which produces the visual sensation upon the human eye. It is usually denoted by Q, expressed in lumen-hours and is analogous to watt-hours. Luminous flux: It is defined as the total quantity of light energy emitted per second from a luminous body. It is represented by symbol F and is measured in lumens (or cd-sr). The concept of luminous flux helps us to specify the output and efficiency of a given light source. Luminous intensity: Luminous intensity in any given direction is the luminous flux emitted by the source per unit solid angle, measured in the direction in which the intensity is required. It is denoted by the symbol I and is measured in candela (cd) or lumens per steradian. If F is the luminous flux radiated out by source within a solid angle of 𝜔 steradians in any particular direction then I=F/𝜔 lumens/steradian or candela (cd). Lumen: The lumen is the unit of luminous flux and is defined as the amount of luminous flux given out in a space represented by one unit of solid angle by a source having an intensity of one candle power in all directions; i.e., Lumens=Candle power × solid angle = CP× 𝜔. Candle power: Candle power is the light radiating capacity of a source in a given direction and is defined as the number of lumens given out by the source in a unit solid angle in a given direction. It is denoted by symbol CP. CP=Lumens/𝜔 Illumination: When the light falls upon any surface, the phenomenon is called illumination. It is defined as the number of lumens, falling on the surface, per unit area. It is denoted by symbol E and is measured in lumens per square metre or lux or metre-candle. If a flux of F lumens falls on a surface of area A, then the illumination of that surface is E=F/A lumens/m 2 or lux (lx). Lux or metre-candle: It is the unit of illumination and is defined as the luminous flux falling per square metre on the surface which is everywhere perpendicular to the rays of light from a source of one candle power and one metre away from it. Foot-candle: It is also the unit of illumination and is defined as the luminous flux falling per square foot on the surface which is everywhere perpendicular to the rays of light from a source of one candle power and one foot away from it; i.e., 1 foot-candle = 1 lumen/ft2 = 10.76 metre-candle or lux. Candela: It is the unit of luminous intensity. It is defined as 1/60 th of the luminous intensity per cm2 of a black body radiator at the temperature of the solidification of platinum (2043 K). Mean horizontal candle power (MHCP): It is defined as the mean of candle power in all directions in the horizontal plane containing the source of light. Mean spherical candle power (MSCP): It is defined as the mean of candle powers in all directions and in all planes from the source of light. Mean hemispherical candle power (MHSCP): It is defined as the mean of candle power in all directions above or below the horizontal plane passing through the source of light. Reduction factor: Reduction factor of a source of light is the ratio of its mean spherical candle power to its mean horizontal candle power; i.e., reduction factor = MSCP/MHCP. Lamp efficiency: It is the ratio of the luminous flux to the power input. It is expressed in lumens per watt. Specific consumption: It is defined as the ratio of the power input to the average candle power. It is expressed in watts per candela. Brightness or luminance: Brightness or luminance is defined as the luminous intensity per unit projected area of either a surface source of light or a reflecting surface and is denoted by L. If a surface of area A has an effective luminous intensity of I candelas in a direction 𝜃 to the normal, then the luminance (brightness) of that surface is L=I/(A cos 𝜃) candela/m2. Lambert is also the unit of brightness which is lumens/cm2. Glare: Glare may be defined as the brightness within the field of vision of such a character as to cause annoyance, discomfort, interference with vision or eye fatigue. Space-height ratio: It is defined as the ratio of horizontal distance between adjacent lamps and height of their mountings. Utilization factor or coefficient of utilization: It is defined as the ratio of total lumens reaching the working plane to total lumens given out by the lamp. Maintenance factor: The ratio of illumination under normal working conditions to the illumination when the things are perfectly clean is known as maintenance factor. Depreciation factor: This is merely the inverse of the maintenance factor and is defined as the ratio of initial metre-candles to the ultimate maintained metre-candles on the working plane. Its value is more than unity. Waste light factor: Whenever a surface is illuminated by a number of sources of light, there is always a certain amount of waste of light on account of overlapping and falling of light outside the edges of the surface. The effect is taken into account by multiplying the theoretical value of lumens required by 1.2 for rectangular areas and 1.5 for irregular areas and objects such as statues, monuments, etc. Absorption factor: The ratio of total lumens available after absorption to the total lumens emitted by the source of light is called the absorption factor. Its value varies from unity for clean atmosphere to 0.5 for foundries. Beam factor: The ratio of lumens in the beam of a projector to the lumens given out by the lamps is called the beam factor. The factor takes into account the absorption of light by reflector and front glass of the projector lamp. Its value varies from 0.3 to 0.6. Reflection factor: When a ray of light impinges on a surface it is reflected from the surface at an angle of incidence. A certain portion of incident light is absorbed by the surface. The ratio of reflected light to the incident light is called reflection factor. It is always less than unity. Solid angle: Solid angle is the angle generated by the surface passing through the point in space and periphery of the area. Solid angle is denoted by 𝜔, expressed in steradians and is given by the ratio of the area of the surface to the square of the distance between the area and the point; i.e., 𝜔=Area/radius2. Steradian: It is the unit of solid angle and is defined as the solid angle that subtends a surface on the sphere equivalent to the square of the radius. Laws of illumination: Law of inverse squares: o If a source of light which emits light equally in all directions be placed at the centre of a hollow sphere, the light will fall uniformly on the inner surface of the sphere, that is to say, each square mm of the surface will receive the same amount of light. If the sphere be replaced by one of the larger radius, the same total amount of light is spread over a larger area proportional to the square of the radius. The amount which falls upon any square mm of such a surface will, therefore, diminish as the radius increases, and will be inversely proportional to the square of the distance. o A similar relation holds if we have to deal with a beam of light in the form of a cone or pyramid. If we consider parallel surfaces which cut the pyramid at different distances from the surface, the areas of these surfaces are proportional to the square of these distances, and, therefore, the amount of light which falls on one unit of the area of these surfaces, is inversely proportional to the square of the distance from the source. o This relationship is referred to as the law of inverse square. The illumination of a surface is inversely proportional to the square of the distance between the surfaces and the light source provided that the distance between the surface and the source is sufficiently large so that the source can be regarded as a single point. Lambert’s cosine law: o Important: Illumination at any point on a plane below the light source Example: Polar curves: None of the practical type of lamp provides light uniformly distributed in all directions because of un- symmetrical shape. It is often necessary to know the distribution of light in various directions to ascertain how the candle power of a light source varies in different directions. The luminous intensity in all the directions can be represented by polar curves. If the luminous intensity in a horizontal plane passing through the lamp is plotted against angular position, a curve known as horizontal polar curve is obtained. If the luminous intensity in a vertical plane is plotted against the angular position, a curve known as vertical polar curve is obtained. Figure: Typical polar curves for an ordinary filament lamp. The polar curves are used to determine the mean horizontal candle power (mhcp) and mean spherical candle power (mscp). These are also used to determine the actual illumination of a surface by employing the candle power in that direction as read from the vertical polar curve in illumination calculations. The mean horizontal candle power of a lamp can be determined from the horizontal polar curve by taking the mean value of the candle power in a horizontal direction. Mean spherical candle power can be determined from the vertical polar curve by Rousseau’s construction: o Figure: Rousseau’s construction: o A semicircle of any convenient radius is drawn with the pole of the polar diagram as centre. o The line CD is drawn equal and parallel to the vertical diameter YY’. o Now from this line CD ordinate equal to corresponding radius on the polar curve are set up such that DB=OK, GH=OF, and so on. o The curve obtained by joining the ends of these ordinates is known as Rousseau’s curve. o The mean ordinate of this curve gives the mscp of the lamp having polar curve as shown in the figure. o The mean ordinate of the curve = (Area CSTGDBHLC) / Length of CD. Photometry: Photometry: Measurement of candle power The candle power of a source in any given direction is measured by comparison with a standard or substandard source employing photometer bench and some form of photometer. The experiment is performed in dark room with dead black walls and ceiling in order to eliminate errors due to reflected light. The photometer bench consists essentially of two steel rods which carry the stands or saddles for holding the two sources, the carriage for the photometer head and for any other apparatus employed in making measurements. One of the bar carries a bar strip, graduated in centimetres and millimetres. The photometer head acts as a screen for comparison of illumination of the standard source and the source under test. The principle of most of the methods of measurement is based on inverse square law. The standard source, whose candle power is known (say S) and the other source under test whose candle power is to be determined, are set on a bench at a distance apart with some type of screen in line with, and between, them. The photometer head or screen is moved in between the two fixed sources until the illumination on both sides of the screen is the same. If the distance of the standard source S and source under test T from photometer head are 𝑙1 and 𝑙2 respectively then according to the inverse square law: 𝐶𝑎𝑛𝑑𝑙𝑒 𝑝𝑜𝑤𝑒𝑟 𝑜𝑓 𝑠𝑜𝑢𝑟𝑐𝑒 𝑢𝑛𝑑𝑒𝑟 𝑡𝑒𝑠𝑡 𝑙22 o = 𝐶𝑎𝑛𝑑𝑙𝑒 𝑝𝑜𝑤𝑒𝑟 𝑜𝑓 𝑠𝑡𝑎𝑛𝑑𝑎𝑟𝑑 𝑠𝑜𝑢𝑟𝑐𝑒 𝑙12 Sources of light: According to principle of operation the light sources may be grouped as follows: o Arc lamps: Electric discharge through air provides intense light. This principle is utilized in arc lamps. o High temperature lamps: Oil and gas lamps and incandescent filament type lamps, which emit light when heated to high temperature. o Gaseous discharge lamps: Under certain conditions, it is possible to pass electric current through a gas or metal vapour, which is accompanied by visible radiations. Sodium and mercury vapour lamps operate on this principle. o Flourescent type lamps: Certain materials, when exposed to ultraviolet rays, transform the absorbed energy into radiations of longer wave length lying within the visible range. This principle is employed in fluorescent lamps. Arc lamps: In an arc lamp electric current is made to flow through two electrodes in contact with each other which are drawn apart. This results in an arc being struck. The arc acts as a source of light. There are various forms of arc lamps such as carbon-arc, flame-arc or magnetic-arc lamps. Carbon-arc lamp: o This is the earliest type of lighting. o Figure: Carbon-arc lamp o When two hard carbon rods are placed end to end and connected to the terminals of DC supply mains of not less than 45 volts, the current flows through them and the ends of the carbon rods soon become incandescence due to high resistance. o If they slightly pulled (say about 2 or 3 mm) an arc will be formed between two carbon rods and white light will be produced. o The arc is maintained by transfer of carbon particles from one rod to another rod. It is found that these particles travel from the positive carbon rod to negative one. That is why, the positive rod after sometime of use becomes hollow. o Due to transfer of particles and oxidation both rods burn away. The rate of burning of positive rod is twice that of negative rod, that is why, the cross-section of the positive rod is made twice of that of negative one. o In case of AC supply, the rate of burning of both rods is same and, therefore, they are made of equal cross-section. o The voltage required for maintaining the arc is given approximately by 𝑉 = 39 + 2.8𝑙 volts where 𝑙 is the length of the arc in mm. o A series resistance is used to stabilize the arc. In case of AC supply an inductor can be used in place of series resistance. Flame-arc lamp: o Figure: Flame-arc lamp o The principle of operation is similar to that of carbon-arc lamp. o The electrodes of such a lamp have 5 to 15% fluoride (called the flame material) and 85 to 95% carbon. o The fluoride has a characteristic which radiates light energy efficiently from a very high heated arc stream. Fluoride turns into vapour along with the carbon and these fluoride vapours cause very high luminous intensities. o In addition to fluoride, there are also some other flame materials. o Different flame materials will produce different colours. o The arc can be drawn out to one side with the help of a magnet. o The luminous efficiency of such a lamp is 8 lumens per watt. Incandescent lamps: When an electric current is passed through a fine metallic wire, heat is produced and the temperature of the wire increases. At low temperatures, the wire radiates heat energy, as the temperature of the wire increases due to heating it radiates heat as well as light energy. The higher the temperature of the wire, the higher is the amount of light energy radiated. The incandescent or filament type lamp consists of a glass globe completely evacuated and a fine wire, known as filament within it. The glass globe is evacuated to prevent the oxidation and convection currents of the filament and also to prevent the temperature being lowered by radiation. The material, which can be used for the filaments of incandescent lamps, must possess the properties of high melting point, low vapour pressure, high resistivity, low temperature coefficient, ductility and sufficient mechanical strength to withstand vibrations during use. The materials which can be used for the filaments in incandescent lamps are carbon, osmium, tantalum and tungsten. Tungsten is most commonly used metal for filament due to its high melting point (3400 ℃), high resistivity, low temperature coefficient, low vapour pressure, being ductile and mechanically strong to withstand vibration during use. The average efficiency of tungsten filament lamp is around 10 lumens per watt. The light spectrum of an incandescent lamp is continuous, i.e., it contains all the colours, but contains excess of red and yellow and less of blue and violet radiations. Aging effects: o As the tungsten lamp ages, its light output decreases for two reasons. ▪ Evaporation of the filament tends to cause the bulb to blacken. ▪ Also, evaporation makes the filament slowly decrease in diameter, which means that the resistance of filament increases. Therefore, an old filament draws less current and operates at a lower temperature, which reduces its light output. Effects of voltage variations: o Lumens output ∝ 𝑉 3.55 o Power consumption ∝ 𝑉 1.55 o Luminous efficiency ∝ 𝑉 2 o Life ∝ 𝑉 −13 for vacuum lamps and life ∝ 𝑉 −14 for gas filled lamps Advantages: o Operating power factor unity o Direct operation on standard distribution voltage o Availability in various shapes and shades o Good radiation characteristic in the luminous range o No effect of surrounding air temperatures Filament dimensions: o Depending upon the voltage and wattage the diameter of a tungsten lamp filament may be as small as 10 microns. o Assume heat loss by convection is negligible compared with that lost by radiation. 𝑙 𝑙 4𝜌𝑙 o The power absorbed by the filament = 𝐼 2 𝑅 = 𝐼 2 𝜌 = 𝐼 2 𝜌 𝜋 = 𝐼2 𝐴 𝑑2 𝜋𝑑 2 4 o The power radiated by the filament = 𝑒𝐾 × 𝑠𝑢𝑟𝑓𝑎𝑐𝑒 𝑎𝑟𝑒𝑎 × (𝑇1 4 − 𝑇0 4 ) = 𝑒𝐾 × 𝜋𝑑𝑙 × (𝑇1 4 − 𝑇0 4 ); where 𝑇0 is the temperature of the surrounding medium. 4𝜌𝑙 o Power absorbed = Power radiated; i.e., 𝐼 2 = 𝑒𝐾 × 𝜋𝑑𝑙 × (𝑇1 4 − 𝑇0 4 ) 𝜋𝑑 2 3 𝑒𝐾𝜋2 o Thus, 𝐼 = 𝑑 2 √ (𝑇1 4 − 𝑇0 4 ) 4𝜌 Gas filled lamps: o Figure: Gas filled lamp o A metal filament can work in an evacuated bulb up to 2000 ℃ without oxidation and if it is worked beyond this temperature, it vapourizes quickly and blacken the lamp. o For higher efficiency, it is necessary to use working temperature more than 2000 ℃ keeping down the evaporation, which is possible by filling the bulb with an inert gas-argon with a small percentage of nitrogen. Nitrogen is added to reduce the possibility of arcing. o However, due to presence of gas there is heat loss due to convection currents. o This loss depends on the surface area of the filament. o As such coiled coil filaments, which take much less space compared with coiled filaments are used with such lamps. o Helical filaments also have a slower rate of tungsten evaporation. o In a gas filled lamp, the hot gas carries the tungsten vapour upward. Therefore, a black spot forms at the top of the bulb instead of spreading over the entire inner surface, as in high-vacuum bulb. o Chemicals called “getters” are often placed inside the bulb to capture tungsten vapour and thereby reduce the rate of blackening. o Efficiency of coiled-coil lamp is about 12 lumens/watt. o For low wattage lamps, however, the heat loss due to introduction of gas is more than in medium wattage lamps, so for low wattage (up to 40 watts) vacuum type lamps are used. Halogen lamps: o The addition of a small amount of halogen vapour to the filling gas restores part of the evaporated tungsten vapour back to the filament by means of chemical reaction, i.e., there is a sort of ‘regenerative cycle’. o Advantages: ▪ No blackening of lamp, hence no depreciation of lumens output. ▪ High operating temperature wit increased luminous efficiency varying from 22 lumens per watt to 33 per watt. ▪ Reduced dimensions of lamps. ▪ Long life o Halogen lamps are suitable for outdoor illumination of buildings, playing fields, large gardens, fountains, car parks, airport runways, etc. Drawbacks of incandescent lamps: o Low efficiency o Colured light Gaseous discharge lamps: Gaseous discharge lamps have been developed to overcome the drawbacks of incandescent lamps. Figure: Gaseous discharge lamp Gases are normally poor conductors, specially at atmospheric and higher pressures, but application of suitable voltage, called the ignition voltage, across the two electrodes can result in a discharge through the gas, which is accompanied by electromagnetic radiation. The wavelength of this radiation depends upon the gas, its pressure, and the metal vapour used in lamp. Argon gas and sodium and mercury vapours are commonly employed in manufacturing of gaseous discharge lamps. Once the ionization has commenced in the gas, it has a tendency to increase continuously accompanied by a fall in the circuit resistance, i.e., gaseous discharge lamp possesses a negative resistance characteristic. In order to limit the current to a safe value use of a choke or a ballast is made. The choke provides the dual functions of providing the ignition voltage initially, and limiting the current subsequently. Since due to use of choke the power factor becomes poor (0.3-0.4), therefore, in order to improve the power factor of the gaseous discharge lamp use of a condenser is made. The light spectrum obtained is, however, discontinuous (i.e., it consists of one or more coloured lines). The colour of the light depends upon the nature of the gas or vapour used. Types: o Those which give the light of the same colour as produced by the discharge through the gas or vapour such as sodium vapour, mercury vapour, and neon gas lamps. o Those which use the phenomenon of fluorescence and are known as fluorescent lamps. In these lamps, the discharge through the vapour produces ultraviolet waves which cause fluorescence in certain materials called as phosphors. The inside of a fluorescent lamp is coated with a phosphor which absorbs invisible ultraviolet rays and radiates visible rays. Example is fluorescent mercury vapour tube. The gaseous discharge lamps are, in general, considered superior to metal filament lamps. However, they suffer from the following drawbacks: o High initial cost and poor power factor o Starting is somewhat complicated requiring starters in some cases and transformer in others o These take time to attain full brilliancy o Ballasts are necessary for stabilizing the current since the lamps have negative resistance characteristic o Light output fluctuates at twice the supply frequency. The flicker causes stroboscopic effect. o These lamps are only used in particular position. Soldium vapour discharge lamp: o Figure: Sodium vapour discharge lamp ~ o Principally the sodium vapour discharge lamp consists of a bulb containing a small amount of metallic sodium, neon gas, and two sets of electrodes connected to a pin type base. o The presence of neon gas serves to start the discharge and to develop enough heat to vaporize the sodium. o The discharge envelope is usually bent into U shape. o The lamp operates at a temperature-like 300 ℃ and in order to conserve the heat generated and assure the lamp operating at normal air temperature, the discharge envelope is enclosed in a special vacuum envelope designed for this purpose. o Sodium vapour lamp is only suitable for alternating current, and therefore, requires choke control. This requirement is met by operating the lamp from a stray field, step-up, tapped auto- transformer with an open-circuit secondary voltage of 470 to 480 volts. The uncorrected power factor is low, about 0.3 and a capacitor must be used to improve the power factor to about 0.8. o When the lamp is not in operation, the sodium is usually in the form of solid deposited on the side walls of the tube, therefore, at first when it is connected to across the supply mains the discharge takes place in the neon gas and gives red-orange glow. The metallic sodium gradually vaporizes and the ionizes, thereby producing the monochromatic yellow light. o The lamp comes to its rated light output in approximately 15 minutes. o The efficiency of the sodium vapour lamp under practical conditions is 40-50 lumens/watt. o The major application of this type of lamp is for highway and general outdoor lighting such as street lighting, parks, rail yards, storage yards, etc. High pressure mercury vapour discharge lamp: o The mercury vapour discharge lamp is similar in construction to the sodium vapour discharge lamp. It consists of a discharge envelope enclosed in an outer bulb or ordinary glass. o The space between the bulb is partially or completely evacuated to prevent heat loss by convection from the inner bulb. o The outer bulb absorbs harmful ultraviolet rays. o The inner bulb contains argon and a certain quantity of mercury. o When the supply is switched ON, full mains voltage is applied between the auxiliary electrode and neighbouring main electrode; this breaks down the gap and a discharge through the argon gas takes place. This enables the main discharge to commence. As the lamp warms up, mercury is vapourised, increasing the vapour pressure and the luminous column becomes brighter and narrower. o The lamp requires 4 or 5 minutes to attain full brilliancy. o The choke is provided to limit the current to a safe value. This choke lowers the power factor, so a capacitor is connected across the circuit to improve the power factor. o The temperature of operation inside the inner bulb is about 600 ℃. o It gives greenish blue colour light. o The efficiency is about 30 to 40 lumens/watt. o Lamps of this type are used for general industrial lighting, railway yards, ports, shopping centres etc. Mercury iodide lamps: o These lamps are similar in construction to high pressure mercury vapour lamps but in addition to mercury, a number of iodides are added which fill the gap in the light spectrum, and thus, improve the colour characteristic of light. o The efficiency is also higher (75-90 lumens/watt). o A separate ignition device, in addition to the choke, is required for the mercury iodide lamp. o Such lamps are suitable for suitable for applications in the fields of floodlighting, industrial lighting, and public lighting. Fluorescent tubes: o The efficiency of fluorescent lamp is about 40 lumens per watt, about three times the efficiency of an equivalent tungsten filament. o The fluorescent tube consists of a glass tube 25 mm in diameter and 0.38-1.52 m in length. o The inside surface of the tube is coated with a thin layer of fluorescent material in the form of a powder. o The coating materials used depend upon the colour effect desired and may consist of zinc silicate, cadmium, silicate or calcium tungstate. o These organic chemicals are known as phosphors which transforms short-wave invisible radiations into visible light. o By mixing the various powders light of any desired colour including day light can be obtained. o The tube contains small quantity of argon gas at a pressure of 2.5 mm of mercury and one or two drops of mercury. o It is provided with two electrodes coated with electron emissive material. o A starting switch is provided in the circuit, which puts the electrodes directly across the supply mains at the time of starting, so that electrodes may get heated and emit sufficient electrons. o A stabilizing choke is connected in series with it, which acts as a ballast in running condition and provides a voltage impulse for starting. o A capacitor is connected across the circuit to improve the power factor. o The fluorescent lamp may cause a certain amount of radio interference. The radio interference effect may be reduced to a minimum by connecting a small capacitor (0.05 𝜇F) across starter terminals. o The starting switches are of two types: thermal type and glow type ▪ Thermal type: Figure: Thermal type starter The thermal starter is a current operated device and consists of two metallic strips and a heater coil. The bimetallic strips are in contact with each other when the lamp is not in operation. When the supply is switched ON, the two electrodes get connected in series through the thermal switch, the relatively larger current rising them to incandescence. The current also flows through the heater element as a result of which bimetallic strips break current. This causes interruption in the current flowing through the circuit, which further results in a high voltage surge across the electrodes of the tube, which is enough to strike the arc between the electrodes. The arc is then maintained by the normal lamp voltage. Bombardment of the electrode’s surface by the positive mercury ions maintains their temperature so that they continue to emit electrons. Thus, the tube is put in operation. The thermal switch is now generally obsolete because of its more complicated construction, greater cost and greater power loss. ▪ Glow type: The glow type starter is a voltage operated device and consists of two bimetallic electrodes enclosed in a glass bulb filled with a mixture of helium and hydrogen. Normally the contacts are open. When the supply is switched ON, the potential across bimetallic electrodes causes a small glow discharge at a small current not enough to heat up the tube electrodes (filament). This discharge is enough, however, to heat the bimetallic strips of the switch causing them to bend and make contact. This results in a large current through the electrodes, their temperature being raised to incandescence and the gas in the immediate neighbourhood is ionized. After one or two seconds the bimetallic strips cool down and contacts open. This opening of contacts in series with the choke causes a momentary high voltage, which is sufficient to start the discharge in the main tube. The starter ceases to glow as the voltage is now too low. A capacitor is placed inside the starter to suppress arcing and radio interference. Figure: Glow type starter o Stroboscopic effect: ▪ As the usual alternating current supply frequency of 50 Hz, a discharge lamp will be extinguished 100 times per second. ▪ Although this effect is seldom noticeable in normal conditions. It is possible that this may, in some instances, give rise to a stroboscopic effect, that is moving objects such as rotating parts of machinery, illuminated by this light, may appear to be moving in disunity or jerks, or rotating slowly than their normal speed. ▪ In actual application, however, where this effect might cause annoyance, it can be practically eliminated in a three lamp unit by connecting each lamp on a separate phase of 3-phase system and it can be greatly reduced in a two lamp unit by the use of a two lamp control unit, which employs a condenser in the ballast of one of the lamps. ▪ The current through the lamps is have a phase difference (close to 90°) and under these conditions light output of one of the lamps is at maximum. ▪ This method has an additional advantage of giving an overall power factor of nearly unity for the unity of two lamps. ▪ In this arrangement one circuit remains at a low power factor at about 0.5 lagging, while the other circuit incorporating a series capacitor remains at a power factor of about 0.5 leading. o Fluorescent lamp for DC source: ▪ The choke coil has a low impedance on DC and therefore, a ballast resistance is connected in series with the choke in order to limit the current. ▪ On systems below 220 V, starting becomes less certain on DC. Only thermal type starters should be used. ▪ The positive end becomes relatively dark on account of the tendency of the mercury vapour to migrate towards the negative end of the tube. In order to overcome this defect a reversing switch is included in the circuit between the supply and fitting. ▪ Figure: Operation of fluorescent tube on DC ▪ In DC operation of fluorescent tube there is no problem of power factor correction and no stroboscopic effect. ▪ Its disadvantages are: Low efficiency due to power loss in ballast series resistance Increased cost of the ballast resistance and reversing switch Less life of the tube (about 80% of that with AC operation) o Colour of fluorescent light: ▪ Colour of fluorescent light depends on the fluorescent powder used and the vapour pressure in the lamp. o Life: ▪ The normal life of a fluorescent lamp is 7500 hours. ▪ The average life is for three burning hours per switching operation. ▪ Frequent starting of lamps may take more life out of the electrodes than long hours of burning because momentarily there is a higher than normal voltage drop at the electrodes which causes the active material to sputter or evaporate off. o Merits: ▪ High luminous efficiency ▪ Long life ▪ Low running cost ▪ Low glare level ▪ Less heat output o Demerits: ▪ Stroboscopic effect ▪ Noise ▪ Complicated disposal due to mercury Compact fluorescent lamp (CFL) o CFL combines the energy efficiency of fluorescent lighting with the convenience and popularity of incandescent fixtures. o CFLs can replace incandescents that are roughly 3-4 times their wattage, saving up to 75% of the initial light energy. o CFLs cost 3-10 times more than comparable incandescent lamps, they last about 10 times as long (10000 hours). o Complicated disposal due to mercury o Operation: ▪ CFLs work much like standard fluorescent lamps. ▪ They consists of two components: a gas-filled tube, and a magnetic or electronic ballast. ▪ The gas in the tube glows with ultraviolet light when switched ON and electric current from the ballast flows through it. ▪ This is turn excites a white phosphor coating on the inside of the tube, which emits visible light throughout the tube surface. ▪ CFLs with magnetic ballasts flicker slightly at start. They are also heavier than those with electronic ballasts. ▪ Electronic ballasts are more expensive, but light immediately (especially at low temperature). They are also more efficient than magnetic ballasts. ▪ Most currently available CFLs have electronic ballasts. ▪ Electronic ballasts contain a small circuit board with rectifiers, a filter capacitor, and usually two switching transistors connected as a high-frequency resonant series AC to DC inverter. The resulting high frequency (about 40 kHz or higher) is applied to the lamp tube. Since the resonant converter tends to stabilize lamp current and so the light produced over a range of input voltage. ▪ The tube will last about 10000 hours and the ballast about 50000 hours. ▪ Advantages of CFLs over incandescent lamps: Life span: The average rated life of a CFL is about 10 times that of incandescent lamps. The life of a CFL is significantly reduced if it is turned ON and OFF frequently. Energy efficiency: For a given light output, CFLs consume 20 to 30 percent of the power of equivalent incandescent lamps. Efficiency: The typical luminous efficiency of CFLs is 60 to 72 lumens/watt, and that of normal domestic incandescent lamps is 13 to 18 lumens per watt. Embodied energy: CFL need more energy in manufacturing than the incandescent lamp but this embodied energy is offset by their longer life and small energy consumption than equivalent incandescent lamp. Cost: While the purchase price of an integrated CFL is typically 3 to 10 times higher than that of an equivalent incandescent lamp, the extended lifetime and lower energy consumption will more than compensate for the higher initial cost. Incandescent lamps attain full brilliancy a fraction of second after being switched ON, CFLs turn on within a second, but many still take time to warm up to full brilliancy. ▪ Disadvantages of CFLs: Health issues: If individuals are exposed to the light produced by some single- envelope CFLs for long periods of time at distances of less than 20 cm, it could lead to ultraviolet exposures approaching the current workplace limit set to protect workers from skin and retinal damage. Use of double-envelope CFL lamps largerly or entirely mitigates any other risks. Environmental issues: CFLs, like all fluorescent lamps, contain small amount of mercury as vapour inside the glass tubing. Because mercury is poisonous, even these small amounts are a concern for landfills and waste incinerators where the mercury from lamps may be released and contribute to air and water pollution. Basic principle of light control: With most light sources it is desirable to use some additional equipment to direct the whole of the light output on the surface to be illuminated, to diffuse the light in order to prevent glare or to change its colour. Four general methods of light control are available: o Reflection – For changing the direction of light through a large angle o Refraction – For changing the direction of light through a small angle o Diffusion – For scattering the rays in order to prevent glare o Absorption – For absorbing rays of certain wave-lengths in order to change the colour of light In many cases the fittings employed combine two or more of the above functions. Reflection: o When a ray of light impinges on a surface it is reflected from the surface at an angle equal to the angle of incidence. A certain proportion of the incident light will be absorbed by the surface, and the ratio of reflected light energy to incident light energy is known as reflection factor. o There are two types of reflection: (i) mirror or specular reflection and (ii) diffuse reflection. o Specular reflection: ▪ In case of specular reflection, a beam of light is reflected but not scattered, and unless the eye is placed in the path of the reflected beam, the viewer is unaware of the existence of light. ▪ Moreover, if his eye is placed in the path of the reflected beam, he sees, not the illuminated surface but the light source. ▪ Figure: Specular reflection ▪ Surface causing specular reflection are silvered mirrors, highly polished metals, etc. o Diffuse reflection: ▪ With diffuse reflection the reflected light is scattered in all directions, and the viewer sees the illuminated surface, not the light source. ▪ Figure: Diffuse reflection ▪ Surfaces causing diffuse reflection are paper, frosted glass, chalk, dry earth, plaster, etc. ▪ If a surface that is uniformly illuminated by a beam of light appears to be equally bright when viewed from all possible angles the reflection is said to be perfectly diffused. ▪ The reflection from any actual surface is partly specular and partly diffuse, the proportion varying widely. A surface that is almost free from mirror reflection is called a mat surface. Refraction: o When a beam of light is passed through a boundary between two transparent substances having different densities, its direction is changed. This phenomenon is known as refraction. o The ray will always have a smaller angle to the normal to the surface in the material of higher refractive index. o In ordinary lighting practice the materials concerned are nearly always glass and air, having refractive indices of 1.5 and 1 respectively. Diffusion: o To prevent glare form a light source, a diffusing-glass screen can be introduced between the observer and the light source or the light may be reflected from a diffusing surface. Types of lighting schemes: The interior lighting schemes may be classified as (i) direct lighting, (ii) semi-direct lighting, (iii) semi- indirect lighting, (iv) indirect lighting, and (v) general lighting. Figure: General classification of luminaries Direct lighting: o In this lighting scheme more than 90 percent of total light flux is made to fall directly on the working plane with the help of deep reflectors. o Though it is most efficient but causes hard shadows and glare. Semi-direct lighting: o In this lighting scheme 60 to 90 percent of the total light flux is made to fall downwards directly with the help of semi-direct reflectors, remaining light is used to illuminate the ceilings and walls. o Such a lighting scheme is best suited to rooms with high ceilings where a high level of uniformly distributed illumination is desirable. o Glare in such units is avoided by employing diffusing globes which not only improve the brightness toward the eye but also improve the efficiency of the system with reference to the working plane. Semi-indirect lighting: o In this lighting scheme 60 to 90 percent of total light flus is thrown upwards to the ceiling for diffuse reflection and the rest reaches the working plane directly except for some absorption by the bowl. o This lighting scheme is with soft shadows and glare free. o It is mainly used for indoor light decoration purposes. Indirect lighting: o In this lighting scheme more than 90% of total light flux is thrown upwards to the ceiling for diffuse reflection by using inverted or bowl reflectors. o In such a system the ceiling acts as the light source, and the glare is reduced to minimum. o The resulting illumination is softer and more diffused, the shadows are less prominent and the appearance of the room is much improved over that which results from direct lighting. o It is used for decorative purposes in theatres, hotels, etc., and in workshops where large machines and other obstructions would cause troublesome shadows if direct lighting is employed. General lighting: o In this scheme lamps made of diffusing glass are used which give nearly equal illumination in all directions. Design of lighting schemes: The lighting scheme should be such that it may: o Provide adequate illumination o Provide light distribution all over the working place as uniform as possible o Provide light of suitable colour o Avoid glare and hard shadows as far as possible The following factors are required to be considered while designing the lighting system: o Illumination level o Uniformity of illumination o Colour of light o Shadows o Glare o Mounting height o Spacing of luminaries o Colour of surrounding walls Methods of light calculations: A number of methods have been employed for lighting calculations, among which may be mentioned: (i) watts per square metre method, (ii) lumen or light flux method, (iii) point to point or inverse-square law method. Watts per square metre method: o This is principally a rule of thumb method. o It consists in making an allowance of watts per square metre of area to be illuminated according to the illumination desired on the assumption of an average figure of overall efficiency of the system. Lumen or light flux method: o This method is applicable to those cases where the sources of light are such as to produce an approximate uniform illumination over the working plane or where the average value is required. o From the size of lamp or lamps employed and from their efficiency total lumen outputs are determined. o Multiplying the total lumens output from the source by the coefficient of utilization, the lumens received on the working plane are determined. o If the lamps and surroundings are not perfectly clean, then in determination of lumens received on working plane, the depreciation factor or maintenance factor should be included. o 𝐿𝑢𝑚𝑒𝑛𝑠 𝑟𝑒𝑐𝑒𝑖𝑣𝑒𝑑 𝑜𝑛 𝑤𝑜𝑟𝑘𝑖𝑛𝑔 𝑝𝑙𝑎𝑛𝑒 = 𝑁𝑢𝑚𝑏𝑒𝑟 𝑜𝑓 𝑙𝑎𝑚𝑝𝑠 × 𝑤𝑎𝑡𝑡𝑎𝑔𝑒 𝑜𝑓 𝑒𝑎𝑐ℎ 𝑙𝑎𝑚𝑝 × 𝑙𝑢𝑚𝑒𝑛𝑠 𝑒𝑓𝑓𝑖𝑐𝑖𝑒𝑛𝑐𝑦 𝑜𝑓 𝑒𝑎𝑐ℎ 𝑙𝑎𝑚𝑝 𝑖𝑛 𝑡𝑒𝑟𝑚𝑠 𝑜𝑓 ( ) × 𝑐𝑜𝑒𝑓𝑓𝑖𝑐𝑖𝑒𝑛𝑡 𝑜𝑓 𝑢𝑡𝑖𝑙𝑖𝑧𝑎𝑡𝑖𝑜𝑛 × 𝑤𝑎𝑡𝑡 𝑚𝑎𝑖𝑛𝑡𝑒𝑛𝑎𝑛𝑐𝑒 𝑓𝑎𝑐𝑡𝑜𝑟 o 𝐿𝑢𝑚𝑒𝑛𝑠 𝑟𝑒𝑐𝑒𝑖𝑣𝑒𝑑 𝑜𝑛 𝑤𝑜𝑟𝑘𝑖𝑛𝑔 𝑝𝑙𝑎𝑛𝑒 = 𝑁𝑢𝑚𝑏𝑒𝑟 𝑜𝑓 𝑙𝑎𝑚𝑝𝑠 × 𝑤𝑎𝑡𝑡𝑎𝑔𝑒 𝑜𝑓 𝑒𝑎𝑐ℎ 𝑙𝑎𝑚𝑝 × 𝑙𝑢𝑚𝑒𝑛𝑠 𝑒𝑓𝑓𝑖𝑐𝑖𝑒𝑛𝑐𝑦 𝑜𝑓 𝑒𝑎𝑐ℎ 𝑙𝑎𝑚𝑝 𝑖𝑛 𝑡𝑒𝑟𝑚𝑠 𝑜𝑓 ( ) × 𝑐𝑜𝑒𝑓𝑓𝑖𝑐𝑖𝑒𝑛𝑡 𝑜𝑓 𝑢𝑡𝑖𝑙𝑖𝑧𝑎𝑡𝑖𝑜𝑛 × 𝑤𝑎𝑡𝑡 1 ( ) 𝑑𝑒𝑝𝑟𝑒𝑐𝑖𝑎𝑡𝑖𝑜𝑛 𝑓𝑎𝑐𝑡𝑜𝑟 o If waste light factor is considered: 𝐿𝑢𝑚𝑒𝑛𝑠 𝑟𝑒𝑐𝑒𝑖𝑣𝑒𝑑 𝑜𝑛 𝑤𝑜𝑟𝑘𝑖𝑛𝑔 𝑝𝑙𝑎𝑛𝑒 = 𝑁𝑢𝑚𝑏𝑒𝑟 𝑜𝑓 𝑙𝑎𝑚𝑝𝑠 × 𝑤𝑎𝑡𝑡𝑎𝑔𝑒 𝑜𝑓 𝑒𝑎𝑐ℎ 𝑙𝑎𝑚𝑝 × 𝑙𝑢𝑚𝑒𝑛𝑠 𝑒𝑓𝑓𝑖𝑐𝑖𝑒𝑛𝑐𝑦 𝑜𝑓 𝑒𝑎𝑐ℎ 𝑙𝑎𝑚𝑝 𝑖𝑛 𝑡𝑒𝑟𝑚𝑠 𝑜𝑓 ( ) × 𝑐𝑜𝑒𝑓𝑓𝑖𝑐𝑖𝑒𝑛𝑡 𝑜𝑓 𝑢𝑡𝑖𝑙𝑖𝑧𝑎𝑡𝑖𝑜𝑛 × 𝑤𝑎𝑡𝑡 1 ( ) 𝑑𝑒𝑝𝑟𝑒𝑐𝑖𝑎𝑡𝑖𝑜𝑛 𝑓𝑎𝑐𝑡𝑜𝑟 × 𝑤𝑎𝑠𝑡𝑒 𝑙𝑖𝑔ℎ𝑡 𝑓𝑎𝑐𝑡𝑜𝑟 o Utilization factor (coefficient of utilization) depends upon: ▪ The mounting height of lamps ▪ Area to be illuminated ▪ Type of lighting ▪ Colours of surrounding walls o Maintenance factor considers loss in illumination due to aging of the lamps, and accumulation of dust on the lamps and on the reflecting and transmitting surfaces of the fixtures. Point to point or inverse square law method: o This method is applicable where the illumination at a point due to one or more sources of light is required, the candle powers of the sources in the particular direction under consideration being known. o If a polar curve of lamp and its reflector giving candle powers of the lamp in different directions is known, the illumination at any point within the range of the lamp can be calculated from the inverse square law. o If two or more than two lamps are illuminating the same working place, the illumination due to each can be calculated and added. o This method is not much used because of its complicated and cumbersome applications. o It is employed only in some special problems, such as flood lighting, yard lighting, etc. Self-study: Comparison between tungsten filament and fluorescent lamp Illumination level required, as per ISI, in various parts of a building Illumination level required, as per ISI, for various types of traffic routes