Platelet Innate Immune Interactions and Thromboinflammation PDF
Document Details
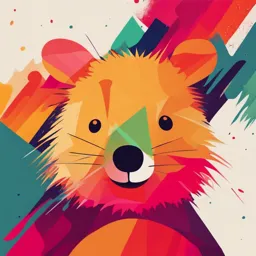
Uploaded by ClearerGenre
Universitätsmedizin der Johannes Gutenberg-Universität
2022
Jonathan Mandel,Martina Casari,Maria Stepanyan,Alexey Martyanov,Carsten Deppermann
Tags
Summary
This review explores the multifaceted roles of platelets beyond hemostasis, focusing on their interactions with innate immune cells and their involvement in inflammatory processes. Specifically, it examines platelet activation mechanisms, including receptor interactions (GPIb, αIIbβ3, etc.), and granule secretions in various diseases, such as atherosclerosis and COVID-19. The article also discusses platelet clearance and interactions with different immune cell types in diseases from cancer metastasis to sepsis.
Full Transcript
International Journal of Molecular Sciences Review Beyond Hemostasis: Platelet Innate Immune Interactions and Thromboinflammation Jonathan Mandel 1 , Martina Casari 1 , Maria Stepanyan 1,2,3,4 , Alexey Martyanov 2,4,5 and Carsten Deppermann 1, *...
International Journal of Molecular Sciences Review Beyond Hemostasis: Platelet Innate Immune Interactions and Thromboinflammation Jonathan Mandel 1 , Martina Casari 1 , Maria Stepanyan 1,2,3,4 , Alexey Martyanov 2,4,5 and Carsten Deppermann 1, * 1 Center for Thrombosis and Hemostasis, University Medical Center of the Johannes Gutenberg-University, 55131 Mainz, Germany; [email protected] (J.M.); [email protected] (M.C.); [email protected] (M.S.) 2 Center For Theoretical Problems of Physico-Chemical Pharmacology, 109029 Moscow, Russia; [email protected] 3 Physics Faculty, Lomonosov Moscow State University, 119991 Moscow, Russia 4 Dmitriy Rogachev National Medical Research Center of Pediatric Hematology, Oncology Immunology Ministry of Healthcare of Russian Federation, 117198 Moscow, Russia 5 N.M. Emanuel Institute of Biochemical Physics RAS (IBCP RAS), 119334 Moscow, Russia * Correspondence: [email protected] Abstract: There is accumulating evidence that platelets play roles beyond their traditional functions in thrombosis and hemostasis, e.g., in inflammatory processes, infection and cancer, and that they interact, stimulate and regulate cells of the innate immune system such as neutrophils, monocytes and macrophages. In this review, we will focus on platelet activation in hemostatic and inflammatory processes, as well as platelet interactions with neutrophils and monocytes/macrophages. We take a closer look at the contributions of major platelet receptors GPIb, αIIb β3 , TLT-1, CLEC-2 and Toll-like receptors (TLRs) as well as secretions from platelet granules on platelet–neutrophil aggregate and Citation: Mandel, J.; Casari, M.; neutrophil extracellular trap (NET) formation in atherosclerosis, transfusion-related acute lung injury Stepanyan, M.; Martyanov, A.; (TRALI) and COVID-19. Further, we will address platelet–monocyte and macrophage interactions Deppermann, C. Beyond Hemostasis: during cancer metastasis, infection, sepsis and platelet clearance. Platelet Innate Immune Interactions and Thromboinflammation. Int. J. Keywords: platelets; hemostasis; thrombosis; neutrophils; monocytes; macrophages; inflammation; Mol. Sci. 2022, 23, 3868. https:// NETs; COVID-19; atherosclerosis; cancer doi.org/10.3390/ijms23073868 Academic Editors: Kerstin Jurk, Marijke J.E. Kuijpers and Johan W. M. Heemskerk 1. Platelets Platelets are key drivers of hemostasis and thrombosis. Dysfunction in platelet ad- Received: 2 March 2022 Accepted: 29 March 2022 hesion, production and clearance lead to cardiovascular diseases (CVD). According to Published: 31 March 2022 the World Health Organization (WHO), CVDs are the leading cause of death worldwide, with an estimated 17.9 million deaths in 2021. After vessel injury, platelets adhere Publisher’s Note: MDPI stays neutral to the endothelial matrix and become activated, which leads to thrombus formation and with regard to jurisdictional claims in restoration of vascular integrity. In recent years, there has been accumulating evidence that published maps and institutional affil- platelets play roles beyond thrombosis and hemostasis, e.g., in inflammatory processes, iations. infection and cancer. In this review, we will focus on the role of platelet activation in hemostatic and inflammatory processes, as well as their interactions with innate immune cells (neutrophils and macrophages) in disease. Copyright: © 2022 by the authors. 1.1. Platelet Adhesion Licensee MDPI, Basel, Switzerland. This article is an open access article After being released from megakaryocytes in the bone marrow, platelets circulate for distributed under the terms and 7–10 days in the vasculature before they are cleared, mainly by macrophages in the liver. conditions of the Creative Commons Their adhesion receptors and biomechanical properties make platelets efficient guardians Attribution (CC BY) license (https:// of hemostasis. creativecommons.org/licenses/by/ Platelets possess three different types of storage granules (α-granules, δ-granules and 4.0/). lysosomes), which contain receptors and soluble proteins as well as bioactive molecules, Int. J. Mol. Sci. 2022, 23, 3868. https://doi.org/10.3390/ijms23073868 https://www.mdpi.com/journal/ijms Int. J. Mol. Sci. 2022, 23, 3868 2 of 30 which play important roles not only in hemostasis, but also during inflammation and immunity. Hydrodynamic shear forces generated by the blood flow cause erythrocytes to flow in the center of the vessel and push platelets to the vessel wall. After vascu- lar injury, interactions between adhesion receptors on platelets and extracellular matrix (ECM) proteins exposed by the vascular injury decrease the velocity of platelets and allow thrombus formation. Under low shear conditions present in the venous circulation, integrin α2 β1 , α5 β1 and α6 β1 mediate platelet binding to the ECM proteins collagen, fibronectin and laminin. At higher shear rates present in arteries and arterioles, efficient platelet adhesion depends on the von Willebrand factor (vWF). The vWF is a large glycoprotein produced by megakaryocytes and endothelial cells that circulates in the vasculature in a complex with coagulation factor VIII and is stored in the α-granules of platelets or Weibel–Palade bodies of endothelial cells [6,7]. A shear-induced conformational change of vWF leads to exposure of the A1, A2 and A3 domains and initiates binding of collagen to the A3 domain. Subsequent binding of A3 to collagen further triggers the exposure of the A1 domain. Under lower shear rates, adhesion is mediated through integrin αIIb β3 on the surface of platelets binding to immobilized vWF on collagen via its C1 domain [9–11]. Under higher shear rates, the adhesion of platelets is mainly mediated by vWF binding to glycoprotein Ib (GPIb). The A1 domain on vWF binds to a leucine-rich repeat on the N-terminal domain of GPIb. After vWF-GPIb binding, the stalk region of GPIα unfolds, tensile stress is generated and downstream signaling is initiated. Intracellular signaling leads to granule exocytosis and the activation of αIIb β3 -integrin. vWF binding is characterized by a rapid on–off binding to αIIb β3 and GPIb, allowing transient interactions [12–14]. A stable connection between platelets and collagen is achieved by collagen–GPVI binding, leading to full platelet activation. Soluble agonists, for instance, fibrinogen, ADP, thromboxane A2 (TxA2 ) and thrombin, further support platelet activation and aggregation [9,15]. 1.2. GPIb Is a Key Receptor for Platelet Activation and Aggregation GPIb is exclusively expressed on the surface of platelets and megakaryocytes and is part of the GPIb-IX-V complex, which consists of four type-I transmembrane polypeptides: GPIbα, GPIbβ, GPIX and GPV. GPIb-IX-V transduces signals via interactions with the cy- toskeleton and without a catalytic intracellular domain. Using an optical tweezer setup with single-molecule force measurement, Zhang and colleagues discovered a mechanosen- sitive domain in GPIbα, which might be responsible for shear force sensing. Down- stream of GPIb, Src/Syk kinases are activated as well as phospholipase C (PLCγ2). This leads to Ca2+ release, phosphoinositide 3-kinase (PI3K) and protein kinase C (PKC) ac- tivation [14,18]. Consequently, the binding of the tyrosine kinase Syk leads to the phos- phorylation of the linker for activation of T cells (LAT) and the further recruitment of phosphoinositide 3-kinase (PI3K). Phosphatidylinositol (4,5)-bisphosphate (PIP2) is con- verted by PI3K into phosphatidylinositol (3,4,5)-triphosphate (PIP3). Subsequently, PLCγ2 cleaves PIP3, which generates inositol-1,4,5-triphosphate (IP3) and diacylglycerol (DAG). DAG initiates Ca2+ release, which, in turn, activates PKC. This ultimately results in granule secretion and the upregulation of activated integrin with increased ligand affinity to further enhance platelet aggregation [18,19]. In 1985, Harmon and Jamieson found that thrombin can bind to platelets via GPIb. A study by Dörmann, Clemetson and Kehrel showed that, together with protease-activated receptor-1 (PAR-1) activation, platelet–platelet interaction and αIIb β3 -fibrinogen binding, GPIb binding to thrombin is an essential part in platelet pro-coagulation activity. An analysis of the protein crystal structure revealed that thrombin shares a binding site with vWF [22,23]. It was hypothesized that thrombin interferes with vWF–GPIb binding, thereby also contributing to an anti-coagulant activity, but studies providing definitive proof are missing. P-selectin is a transmembrane protein stored in α-granules and Weibel–Palade bodies within platelets and endothelial cells, respectively. The translocation of P-selectin to the Int. J. Mol. Sci. 2022, 23, 3868 3 of 30 surface of activated platelets mediates inflammatory processes by interacting with P-selectin glycoprotein ligand-1 (PSGL-1) on leukocytes and endothelial cells, but also on platelets. Interestingly, PSGL-1 and GPIb share structural similarities. Both are sialomucins with a cluster of O-linked carbohydrates and express a cluster of anionic amino acids with sulfated tyrosine. Studies suggest that P-selectin on platelets binds to PSGL-1 on endothelial cells after vessel destruction and promotes adhesion. Filamin A is an actin-binding protein and is essential in platelet adhesion and aggre- gation. It modulates vWF–GPIb-IX-V interactions by binding to the cytoplasmic tail of GPIbα and supporting the interaction between GPIb-IX-V and actin. Experiments using platelets from mice with a transgenic GPIbα containing a defective filamin A binding site revealed impaired platelet adhesion and loss of membrane integrity under high shear conditions. Interactions between GPIb and coagulation factors such as factor XI, factor XII, factor IX, factor VII, kininogen, protein C and vitamin K have been described. It is assumed that GPIb localizes factor IX to the platelet surface to support thrombin formation. These factors not only support platelet aggregation but are essential in hemostasis. 1.3. CLEC-2 in Platelet Activation and Immunity C-type lectin-like receptor 2 (CLEC-2) is a type-II transmembrane receptor expressed as a dimer on platelets, neutrophils, dendritic cells (DCs) and Kupffer cells with an extra- cellular lectin-like domain. CLEC-2 contains an immunoreceptor tyrosine-based activation motif (ITAM) with a single YXXL motif (hemITAM) necessary for direct signal transduction through the tyrosine kinase Syk [19,32]. Podoplanin is the main binding partner of CLEC-2, expressed on lymphatic endothelial cells and also on inflammatory macrophages. The snake venom rhodocytin is another ligand for CLEC-2 and is frequently used to study its signaling. CLEC-2–podoplanin binding initiates platelet activation and aggregation similar to GPVI through Src, LAT, PLCγ2 and DAG signaling [34,35]. Experiments with CLEC-2-deficient mice showed defective thrombus formation as well as prolonged tail bleeding time in vivo, indicating that CLEC-2 plays an essential role in hemostasis and thrombosis. CLEC-2 also contributes to thromboinflammation; e.g., podoplanin–CLEC-2 inter- actions on neutrophils or DCs lead to increased protrusions and motility. CLEC-2 activation leads to DC migration to the lymph node and T cell zone to initiate an immune response. Studies showed that CLEC-2 is also involved in hematogenous tumor metas- tasis by mediating platelet aggregation at tumor sites. Platelet aggregates protect cancer cells and secrete factors to promote tumor growth and proliferation [38,39]. 1.4. TLT1 The TREM-like transcript 1 (TLT-1) is part of the triggering receptor expressed on the myeloid cells (TREM) protein family. It has a single Ig superfamily V-set domain and signals downstream via two immunoreceptor tyrosine-based inhibitory motifs (ITIM). During platelet production, TLT-1 is stored in α-granules. After platelet activation, it is translocated to the plasma membrane and also released in soluble form. Soluble TLT-1 (sTLT-1) supports the aggregation of platelets during vascular injury and is also involved in inflammatory diseases and sepsis where sTLT-1 levels are increased. 1.5. Toll-like Receptors (TLRs) and Thromboinflammation Through their Toll-like receptors (TLRs), platelets can sense pathogens such as bacteria and viruses. For instance, lipopolysaccharides (LPS) or viral infections can trigger a pro- inflammatory platelet response with the release TNF and IL-1 [43,44]. Platelets express all nine TLRs. Platelets TLR2 and TLR4 play a major role by binding gram-positive and gram-negative bacteria, respectively. TLR4 is expressed as a homodimer, whereas TLR2 forms heterodimers with TLR1 or TLR6. To a lower extent, platelets also express endosomal TLRs (TLR3, TLR7 and TLR9), which allow for the recognition of viruses [2,45]. The Int. J. Mol. Sci. 2022, 23, 3868 4 of 30 activation of platelet TLRs leads to aggregation but also a pro-inflammatory response. TLR1/TLR2 stimulation with a synthetic agonist (Pam3CSK4), for instance, triggers platelet aggregation. Moreover, the secretion of pro-inflammatory cytokines and chemokines such as RANTES (regulated upon activation, normal T cell expressed and presumably secreted), PDGF, PF4 and neutrophil extracellular trap (NET) formation was observed. The TLR4 response to LPS is well established and leads to the release of cytokines, IL-1β synthesis, platelet–neutrophil aggregate formation and NET formation [45,48]. TLR4 stimulation also potentiates platelet activation in response to hemostatic agonists such as collagen through Akt and ERK signaling. In conclusion, TLRs on platelets have an impact on platelet aggregation but also contribute to thromboinflammation. 1.6. Platelet Granules Platelets communicate with their environment not only via membrane receptors but also via soluble factors released from granules, which also contain receptors that are recruited to the platelet surface upon stimulation. Human platelets contain around 40–80 α-granules, 4–8 dense granules and a few lysosomes. α-granules contain more than 300 different proteins, whereas dense granules contain bioactive amines and nu- cleotides, such as ADP and ATP, as well as magnesium and calcium (Table 1). Granule development starts during the maturation of platelets by megakaryocytes within the bone marrow [50,51]. Table 1. Properties of α-granules and dense granules [42,50–53]. α-Granules Dense Granules Peripheral membrane with an electron-dense nucleoid (chemokines and Peripherally distributed and proteoglycans), an adjacent zone with less electron-dense fibrinogen and a Structure electron-dense spherical bodies peripheral zone with vWF stored in tubular structures 150 nm in diameter Ovoid 250 nm × 300 nm Membrane proteins: integrins (αIIb, α6, β3), immunoglobulin family receptors (GPVI, Fc receptors, PECAM), leucine-rich family receptors (GPIb-IX-V), tetraspanins, TREM-like transcript-1 (TLT-1), fibrocystin L, Soluble proteins: Bioactive amines CD109, P-selectin and other (CD36, Glut-3) (serotonin and histamine), adenine Content Soluble proteins: platelet factor 4 (PF4), fibrinogen, vWF, factor V, factor XI, nucleotides (ADP and ATP), Ca2+ , factor XIII, plasminogen activator inhibitor-1 (PAI-1), α2 -antiplasmin, Mg2+ and polyphosphates chemokines, VEGF, endostatin, FGF, EGF, HGF, IGF, TSP-1, PDWHF, antithrombin, tissue factor inhibitor (TFPI), protein S, protease nexin-2 and pro-inflammatory cytokines Multi-vesicular bodies (MVBs) derived from the endosomal system Multi-vesicular bodies (MVBs) derived from the trans-Golgi network Molecules involved: Adapter Biogenesis Molecules involved: clathrin, COPII, Adapter proteins (AP-1-3), SNAREs protein 3 (AP-3) and biogenesis of and GTPases lysosome-related organelles (BLOC 1-3) Proteins produced in ER of MKs and sorted via trans-Golgi Sorting varies between molecules Transport of molecules via Mechanisms involved: Signal sequence (e.g., Chemokines), membrane pumps (e.g., vesicular Protein glycosaminoglycans (e.g., soluble proteins), aggregation of protein nucleotide transporter (VNUT) and sorting monomers (e.g., vWF), sorting receptors, endocytosis (e.g., plasma proteins multidrug resistance-associated such as fibrinogen) and pinocytosis (e.g., plasma proteins such as albumin or protein 4 (MRP4)) immunoglobulin) Transport Move along microtubules Near the plasma membrane Int. J. Mol. Sci. 2022, 23, 3868 5 of 30 Table 1. Cont. α-Granules Dense Granules Fusing with the plasma membrane Fusing with the plasma membrane by SNAREs (vesicular SNAREs and by SNAREs (vesicular SNAREs and Stimulation target SNAREs) target SNAREs) and release Regulated by Sec1/Munc, CDCrel-1, ATPases, SNAPs and Rab proteins Regulated by Sec1/Munc, CDCrel-1, ATPases, SNAPs and Rab proteins adhesion and coagulation: fibrinogen, vWF, GPIbα-IX-V, integrin αIIb β3 , GPVI, coagulation factors (V, XI, XIII), precursors of thrombin, prothrombin and kininogen as well as inhibitory proteases (plasminogen activator inhibitor-1 (PAI-1) and α-antiplasmin hemostasis: antithrombin, C1-inhibitor, tissue factor pathway inhibitor (TFPI), protein S, protease nexin-2 and proteinase (plasmin and plasminogen) inflammation: P-selectin, pro-inflammatory factors and chemokines (platelet factor 4 (PF-4), CD40, CD154, CXCL1, CXCL4, CXCL5, CXCL7, CXCL8, CXCL12, CCL2, CCL3 and CCL5) Function antimicrobial host defense: CXCL4, thymosin-β4, CXCL7, CCL5 and Potentiate platelet activation complement factors (C1q, C3 and C4 precursors, C8 and C9) angiogenesis: vascular endothelium growth factor (VEGF), platelet-derived growth factor (PDGF), fibroblast growth factor (FGF), epidermal growth factor (EGF), hepatocyte growth factor (HGF) and insulin-like growth factor (IGF) inhibitors of angiogenesis: thrombospondin-1 (TSP-1) and CXCL-4 wound healing: platelet derived wound healing factor (PDWHF) malignancy: VEGF and adhesive proteins such as P-selectin, vitronectin and fibronectin The packaging of α-granules takes place after protein synthesis in megakaryocytes but also mature platelets in the multivesicular bodies that are derived from the trans-Golgi network. In addition, platelets are also able to pick up plasma proteins and pack them into granules by endocytosis. These processes are mediated by adapter proteins (AP1, AP2 and AP3), soluble N-ethylmaleimide-sensitive factor (NSF) attachment protein receptor (SNARE) proteins and regulators, clathrin molecules, biogenesis of lysosome- related organelles complex (BLOC-1 and BLOC-2) as well as vacuolar protein sorting- associated proteins (VPS33B and VPS16B). A summary of major proteins involved in packaging as well as the factors stored in α-granules can be found in Table 1, as discussing all of them would go beyond the scope of this review [42,50,51,55–57]. Upon platelet activation and following Src kinase and PKC signaling, dense granules release soluble factors such as calcium, ATP, ADP and 5-hydroxytryptophan (5-HT). These factors directly activate platelets via receptors such as the purinergic receptors P2Yx in an autocrine and paracrine way [2,50,52]. Defects in platelet granules can lead to serious diseases. One example for an α-granule defect is the gray platelet syndrome (GPS). Mutations in NBEAL2 cause GPS, leading to impaired granule packaging in megakaryocytes and the absence of α-granules in platelets. Symptoms range from a reduced platelet count to bruising susceptibility, myelofibrosis and epistaxis [57–59]. One example of a defect in dense granules is the Hermansky–Pudlak syndrome. This is caused by the mutation of HPS and CHS proteins, such as the AP-3 and Rab38, and leads to a bleeding disorder, recurrent infections and ceroid disposition. 2. Platelets and Neutrophils Interactions in Homeostasis and Pathology Neutrophils are granulocytic cells that are part of the innate immune system and are the first leukocytes to infiltrate the wound site. Interactions between platelets and neutrophils are crucial for inflammation and immunity. Currently, the platelet– neutrophil crosstalk is well characterized (Figure 1), both in physiologic and pathologic conditions, where the thromboinflammatory response is often described as “a vicious Int. J. Mol. Sci. 2022, 23, 3868 6 of 30 cycle”. Upon vessel wall damage, platelets are activated, adhere, change their shape, secrete their granule contents and start to form aggregates. Activated platelets are Int. J. Mol. Sci. 2022, 23, x FOR PEER REVIEW 7 of 32 characterized by P-selectin exposure, which is critical for both hemostasis and platelet– immune cell interactions. Figure 1. Mechanisms of platelet–neutrophil complex formation. Upon endothelial cell disruption, Figure 1. Mechanisms of platelet–neutrophil complex formation. Upon endothelial cell disruption, platelets and neutrophils become activated due to exposure to collagen and activated endothelial platelets and neutrophils become activated due to exposure to collagen and activated endothelial cells. Platelet activation results in P-selectin exposure and subsequent neutrophil activation via P- cells. Platelet activation selectin–PSGL1 results interaction. in P-selectin Neutrophil exposure activation and subsequent is further enhanced upon neutrophil plateletactivation via P- granule content selectin–PSGL1 release (CXCL4,interaction. Neutrophil CXCL7, CXCL1, activation HMGB1). is further enhanced The synergistic activationupon platelet granule of neutrophils content by all these release via agents (CXCL4, Mac-1,CXCL7, PSGL-1,CXCL1, SLC44A2 HMGB1). and CD40Thereceptors synergistic activation eventually of neutrophils results by all these in platelet–neutrophil complexes and neutrophil agents via Mac-1, PSGL-1, DNA-trap SLC44A2 andsecretion—NETosis. NETs contain CD40 receptors eventually the proteases results cathepsin G, in platelet–neutrophil neutrophil complexes elastase and myeloperoxidase, and neutrophil which can both activate DNA-trap secretion—NETosis. NETsplatelets contain and the coagulation the proteases cas- cathepsin cade. Multipleelastase G, neutrophil positiveand feedback loops are present myeloperoxidase, which at can all levels of this system both activate and, platelets thus, and theboth platelet coagulation and neutrophils become involved in immunothrombosis and subsequent thromboinflammation. cascade. Multiple positive feedback loops are present at all levels of this system and, thus, both platelet Cell activation is shown by yellow lightnings. Dotted lines represent interactions of soluble media- and neutrophils become involved in immunothrombosis and subsequent thromboinflammation. Cell tors and receptors. NET—neutrophil extracellular trap, PNC—platelet–neutrophil complex, Plt— activation platelet, is shown by yellow lightnings. Dotted lines represent interactions of soluble mediators Neu—neutrophil. and receptors. NET—neutrophil extracellular trap, PNC—platelet–neutrophil complex, Plt—platelet, Neu—neutrophil. 2.1. Platelet Activation in Thrombi and/or Circulation Can Cause Neutrophil Incorporation PlateletActivation 2.1. Platelet P-selectininisThrombi a ligandand/or for non-activated Circulation Canneutrophil PSGL1 and Cause Neutrophil the P-selectin– Incorporation PSGL1 bond enables neutrophil attraction to sites of thrombus formation under Platelet P-selectin is a ligand for non-activated neutrophil PSGL1 and the P-selectin–low shear stress PSGL1. bond PSGL1 enablesis neutrophil constantly attraction associatedtowith sitesthe ITAM-receptor of thrombus FcRɣ,under formation whichlow is phos- shear phorylated by SFK is stress. PSGL1 upon PSGL1 associated constantly associationwith withthe P-selectin [65,66]. This ITAM-receptor results FcRG, which inisSyk ty- phos- rosine kinase activation, which phosphorylates ADAP adaptor protein and phorylated by SFK upon PSGL1 association with P-selectin [65,66]. This results in Syk leads to the initiation of PLCɣ2-mediated tyrosine kinase activation, which IP3phosphorylates production andADAP calcium signaling adaptor. protein andOn the to leads other the hand, ADAP phosphorylation by Syk also results in PI3K activation, which produces PIP3. PIP3 and calcium synergistically activate the small GTPase Rap1 by inhibiting Rap1 GAP and activating Rap1 GEF, correspondingly. Rap1 activation is the key step in the “inside-out” activation of neutrophil β2-integrins Mac1 and LFA1. Mac1 and LFA1 Int. J. Mol. Sci. 2022, 23, 3868 7 of 30 initiation of PLCG2-mediated IP3 production and calcium signaling. On the other hand, ADAP phosphorylation by Syk also results in PI3K activation, which produces PIP3. PIP3 and calcium synergistically activate the small GTPase Rap1 by inhibit- ing Rap1 GAP and activating Rap1 GEF, correspondingly. Rap1 activation is the key step in the “inside-out” activation of neutrophil β2-integrins Mac1 and LFA1. Mac1 and LFA1 then reinforce neutrophil attachment to platelets upon interaction with JAM3 , ICAM-2 , GPIbA and fibrinogen, bound to platelet AIIb β3 integrin. Recently, a new player in platelet–neutrophil interactions, SLC44A2, was identified. This protein is found on neutrophils and can bind to platelet AIIb β3 integrins under flow. Neutrophil integrin activation, ligation and clustering results in “outside-in” signaling, possibly mediated by Syk and SFK tyrosine kinases, which enhances neutrophil activation [65,66]. The capability of platelets to induce neutrophil activation is not limited to P-selectin– PSGL1 interaction. Platelet A-granules contain a set of chemokines, which are potent stimulators of neutrophil activity. Among the most abundant are CXCL4 (PF4) and CXCL7 (NAP2). PF4 has been shown to mediate neutrophil Mac1 and LFA1 integrin activation [76,77]. However, no direct evidence for the presence of a PF4 receptor on the neutrophil surface has been presented to date. It has been proposed that chondroitin-sulfate proteoglycan could serve as a PF4 receptor; final proof, however, remains lacking. On the contrary, mechanisms of NAP-2-induced neutrophil activation are well understood. NAP-2 is produced from its precursor β-thromboglobulin upon release by neutrophil cathepsin-G. NAP-2 acts through CXCR1 and CXCR2—GPCR receptors which regu- late both neutrophil activation and chemotaxis [80,81]. It is noteworthy that NAP-2 can cause CXCR2 internalization upon activation, which attenuates neutrophil responses. In addition to NAP-2, platelets also contain CXCL1, which similarly activates CXCR1 and CXCR2. Both CXCR1 and CXCR2 cause the GβG -dependent initiation of PLCβ activation and further calcium signaling [84,85]. Interestingly, chemokines can form het- erodimers and, thus, can enhance and modulate the activity of each other. For example, PF4 can form pairs with RANTES (CCL5). Whether such pairing significantly affects platelet–neutrophil interactions has yet to be fully elucidated. Platelets also secrete HMGB1, which can activate both RAGE and TLR4 receptors on neutrophils and, thus, initiate NET formation [88,89]. Finally, upon platelet δ-granule secretion, ADP and ATP are released, which can activate neutrophil purinergic receptors. Other molecules which can mediate neutrophil activation upon platelet granule secretion include TGFβ, CD40L and IL-1β. However, these substances appear to be more potent activators of monocytes instead of neutrophils and their role in platelet–neutrophil interactions is minor. 2.2. Immunothrombosis Pathways Not only platelets can be the initiators of platelet–neutrophil association. Inflammation can cause endothelial dysfunction due to high levels of pro-inflammatory cytokines (IL-1, IL-6 and TNF) as well as ferritin. This results in the activation of endothelial cells and expression of P-selectin [92,93]. Neutrophils interact with endothelial P-selectin and become activated in the same manner as upon interaction with platelet P-selectin [65,66]. On the other hand, bacterial infection can activate neutrophils via PAMP/DAMP receptors TLR2, TLR4, Siglec-14, RAGE and CR3. Neutrophils can also trap viruses into their endosomes. This leads to the decapsulation of the virus and neutrophil TLR7 and TLR8 receptor activation. Activated neutrophils secrete contents of their azurophilic granules, including cathepsin G and NE. Cathepsin G can activate platelet PAR1 receptor in a thrombin-like manner. The same has been assumed for NE; however, no direct experimental evidence is available. Upon hetero-aggregate formation, platelets and neutrophils can activate each other reciprocally. One of the most elegant pathways of such mutual influence is the tran- scellular synthesis of eicosanoids. Arachidonic acid (AA) is a component of membrane phospholipids, which is released by cytosolic phospholipase 2 (PLA2) upon cell activa- Int. J. Mol. Sci. 2022, 23, 3868 8 of 30 tion. In platelets, AA metabolism by COX1 results in the formation of thromboxane A2 (TxA2)—an essential secondary mediator of platelet activation. However, upon AA release from platelet phospholipids, it can be transferred to neutrophils. Neu- trophils are abundant in 5-lipoxygenase (5LO), which is an integral nuclear membrane protein. 5LO activity results in the transition of AA into leukotriene A4 (LTA4), which is further metabolized into leukotriene B4 (LTB4) [91,99]. Alternatively, it can be metabolized into leukotriene C4 (LTC4). Both LTB4 and LTA4 are important pro-inflammatory agents, capable of both activating neutrophils and causing smooth muscle cell contraction and edema. In both activated neutrophils and platelets, reactive oxygen species (ROS) are formed, which enhance the overall activation of both cell types. Additionally, ROS induce NFκB transcription factor activation in neutrophils. NFκB activation can also be triggered by prolonged interaction with platelets via PSGL1 and β2 integrins. This leads to the acquisition of a pro-inflammatory phenotype by neutrophils, characterized by pro-inflammatory cytokine production and, in some cases, tissue factor (TF) surface expression [102,103]. Neutrophils can also activate platelets by releasing antimicrobial cathelicidins via degranulation or as part of NETs. For example, cathelicidin LL-37 and its mouse homologue CRAMP can bind GPVI on the platelet surface, further stimulating platelet and neutrophil activation. In addition to direct platelet activation, activated neutrophils can trigger the activation of the plasma coagulation cascade via TF expression on their surface as well as the secretion of TF-positive microvesicles. This results in the production of thrombin and fibrin generation, which significantly enhances platelet–neutrophil bond formation and mutual activation. It has been suggested that TF-expressing neutrophils and monocytes are key drivers of immunothrombosis [92,103] underlying microvascular thromboinflammation in severe conditions such as ARDS [92,105]. Another distinct feature of platelet–neutrophil interactions in immunothrombotic conditions is the increased potency of neutrophils to form NETs, thus facilitating coagulation and forming a positive feedback loop. 2.3. Platelet–Neutrophil Interactions Result in NETosis Among the most fascinating features of neutrophils is their capability to release neu- trophil extracellular traps (NETs)—cobweb-like chromatin structures, whose prime function is to entangle pathogens (specifically gram-negative bacteria) and to remove them from the circulation. NETosis can be initiated upon neutrophil interaction with fungi, bacteria, immune complexes or activated platelets. The role of platelets in NETosis as well as the overall role of platelets in immunity has emerged only in recent years. Platelet P-selectin interaction with neutrophil PSGL-1 not only results in neutrophil integrin activation by PKC- dependent signaling in neutrophils [66,94]; activated platelets also release HMGB1—which activates the neutrophil RAGE receptors. In addition to HMGB1, activated platelets also secrete the C3a component of the complement system, which, in turn, activates neu- trophil C3a receptor. Combined, these stimuli significantly enhance PSGL-1-induced neutrophil activation and can result in the generation of ROS due to NADP oxidase activity in neutrophils [89,94]. ROS stimulate the myeloperoxidase (MPO)-mediated transition of NE from azurophilic granules to the nucleus. NE synergizes with MPO to initiate chromatin decondensation. The next step in NET formation is chromatin histone citrullina- tion through PAD4. PAD4 activation depends on ROS as well as the cytosolic calcium concentration. PAD4 is also activated downstream of PKC [110–112]. The type of NETosis inducer defines the histone citrullination pattern [110,112]. Thus, it can be speculated that different isoforms of PKC are activated downstream of different activators and differentially regulate PAD4 activation. As a consequence, citrullinated neutrophil chromatin, especially H3-histones, are secreted from neutrophils in complex with MPO and NE, and a NET is formed. Citrullinated H3 histones, MPO and NE triple staining have been accepted as the most reliable markers of ongoing NETosis. NETs are potent inducers of thrombus formation, serving as a scaffold for platelet and coagulation factor binding and activation. It has been shown that platelets directly Int. J. Mol. Sci. 2022, 23, 3868 9 of 30 interact with NETs via several ways. Platelets can be activated by H4 histones both in vitro and in vivo [115,116]. Furthermore, citrullinated H3 histones, one of the key markers of ongoing NETosis, have also been shown to activate platelets. Moreover, platelets can interact with C3b attached to NETs. In addition to NE and MPO, NETs also contain cathepsin G. On the other hand, because of their negative charge, NETs can also activate FXII and, thus, activate the contact pathway of coagulation. In line with this, FXII on NETs is able to activate kallikrein. These events manifest in thrombin generation and fibrin deposition among NETs, which attract new platelets from circulation. Finally, NE and other NET-associated proteins such as cathepsin G may also contribute to coagulation by degrading TFPI, which is the key negative regulator of blood coagulation [114,120]. In line with this, NET–fibrin structures are significantly more resistant to plasmin-induced fibrinolysis. Therefore, NETs can either initiate or contribute to the ongoing thrombosis as well as to the tissue damage due to NE and MPO activity [114,121]. It is assumed that the capability of NETs to induce thrombosis is an important mechanism required for pathogen containment. However, excessive NETosis is a potent driver of thromboinflammation and subsequent thromboembolic complications. 2.4. Endothelium An analysis of the platelet–neutrophil interactions cannot be complete without men- tioning the contribution of endothelial cells. Indeed, blood vessel endothelial cells orches- trate the activation of neutrophils, platelets and the coagulation cascade. Upon activation, endothelial cells secrete the content of their Weibel–Palade bodies including vWF. vWF interacts with platelet GPIbA, which also enables the interaction of platelets with neu- trophil β2 integrins [66,91], and it has been proposed that vWF potentiates this interaction. vWF can attract neutrophils to endothelium independently of platelets, and this process depends on the activity of ADAMTS-13—a metalloproteinase required for the conversion of ultra-large vWF multimers into functional vWF. Other contributions of endothelial cells to platelet–neutrophil heteroaggregate formation are based on their ability to bind pro-inflammatory chemokines to their surface. It has been demonstrated that CCL5 and CXCL4 become immobilized on the endothelium and thereby attract neutrophils and monocytes downstream of the site of ongoing thromboinflammation. Finally, upon neutrophil extravasation from the blood flow, a complex interplay between endothelial cells and platelets maintains blood vessel wall integrity, and this process is mediated by platelet CLEC-2 and GPVI receptors as well as granule release [125,126]. 2.5. Platelet–Neutrophil Interactions in Pathologic States Platelet–neutrophil interactions are crucial in host defense and hemostasis. The number of NETs or platelet–neutrophil complexes (PNCs) in blood has been used as a marker of disease severity [128–131]. Increased amounts of platelet–neutrophil complexes have been observed in autoimmune diseases [74,132,133], acute and chronic inflammatory conditions [134,135] and cardiovascular diseases [136,137]. Ongoing systemic inflammation in immunological diseases may result in increased platelet–neutrophil interactions and a “vicious cycle” of thromboinflammatory responses. For example, patients with ulcerative colitis show a significantly higher number of PNCs and percentage of activated platelets. Platelet activation was proposed to be associated with excessive NET formation in antineutrophil cytoplasmic antibody-associated vasculitis via TLR-CXCL4 signaling. In systemic sclerosis, platelets interact with neutrophils in vitro and in a mouse model and promote neutrophil autophagy via the HMGB1 pathway. Systemic lupus erythematosus (SLE), the most common type of lupus, is a systemic autoimmune disease characterized by a wide range of autoan- tibodies and clinical manifestations. It was shown that neutrophil-to-lymphocyte and platelet-to-lymphocyte ratios are significantly increased in case of SLE nephritis as well as neutrophil and platelet size and number. These indicate neutrophil and platelet involvement in the disease progress as well as platelet pre-activation and/or enhanced Int. J. Mol. Sci. 2022, 23, 3868 10 of 30 production [138,139]. NETs were also shown to mediate pathology in SLE and antiphos- pholipid syndrome. Thromboinflammation is also observed in acute inflammation. Early acute myocardial infarction (AMI) and unstable angina are associated with intense neutrophil activation, in contrast to systemic inflammatory syndromes (stable angina, giant cell arteritis, acute bone fracture). Furthermore, the highest amounts of degranulated neutrophils (measured by MPO presence in neutrophils) were observed 4 h post AMI. MPO depletion was associated with platelet activation and platelet–neutrophil complex formation, and a similar pattern was observed when activated platelets were injected in mice. In a transfusion-related acute lung injury (TRALI) mouse model, an increase in platelet– neutrophil interactions and NETs formation was shown, with less NETs present upon anti-platelet therapy. Increased amounts of NETs were observed in the vessels (post mortem) and plasma of patients with TRALI and ALI. In cases of severe sepsis, only 2% of patients showed neutrophils with unaltered MPO levels, suggesting widespread neutrophil activation. NETs are formed during sepsis and their levels correlate with the severity of organ damage and mortality. A mouse model of sepsis demonstrated that gram-positive bacteria can induce neutrophil activation, PNC formation and platelet activation and aggregation in the infected host. Cardiovascular diseases go hand-in-hand with platelet–neutrophil interactions. For example, high levels of circulating DNA and chromatin, which are evidence of NETosis, were shown to be associated with severe atherosclerosis. Composite biomarker score, calculated using both platelet activation and NETosis markers, was shown to be a risk predictor of acute myocardial infarction. HMGB1 was proposed to play a major role in NETosis during myocardial infarction. A review of platelet–neutrophil interactions in cardiovascular diseases was recently published, which provides more details of the mechanisms involved in different cardiovascular conditions. The authors highlight the importance of procoagulant platelets in ischemic stroke and discuss P-selectin and CD40 involvement in atherosclerosis [147,148] and platelet–neutrophil interplay in thrombus formation. A hyperinflammatory response of the body to infection (cytokine storm) and infiltra- tion of the lungs by neutrophils is observed in COVID-19 [105,121]. Increased NETosis in COVID-19 patients was thoroughly analyzed and the relative number of NETs per neu- trophil was calculated to exclude the influence of neutropenia [121,149]. Active COVID-19 virions as well as platelet-rich plasma of COVID-19 patients induced NETosis in neutrophils of healthy donors [149,150]. Microthrombosis was observed in the lungs of COVID-19 patients and NETs were often found in blood clots surrounded by platelets [151,152]. However, NETosis markers only marginally correlate with clinical markers of disease progression. Changes in D-dimer, fibrinogen and platelet count in COVID-19 indicate the activation of the coagulation cascade. Platelet–leukocyte complexes were elevated in COVID-19 patients, with even higher numbers observed in ICU patients. Platelets were pre-activated: changes in P-selectin exposure and GPIb shedding and elevated sizes and higher levels of sCD40L and sP-selectin were shown [156–158]. A recent review of COVID-19-associated thrombosis, focusing on all potential contributors, including platelets and neutrophils, was published. At present, the exact contribution of neutrophil– platelet interplay to COVID-19 pathogenesis remains to be studied more thoroughly. 3. Monocytes Monocytes are part of the “mononuclear phagocyte system” (MPS), together with macrophages and conventional dendritic cells (cDCs). Circulating monocytes consti- tute 10% of the total leukocyte population in humans and 4% in mice. Monocytes play key roles in tissue homeostasis and immunity, representing a versatile and dynamic cell population, composed of multiple subsets, which differ in phenotype, size, morphology and transcriptional profiles. In humans, three different monocyte populations were first identified by morphology and differential expressions of surface markers CD14 and Int. J. Mol. Sci. 2022, 23, 3868 11 of 30 CD16. The major monocyte subset, accounting for approximately 90% of the total monocyte population, expresses high levels of CD14 and no CD16 (CD14+ CD16− ) and are referred to as classical monocytes. The remaining 10% are shared by CD14+ CD16+ intermediate and CD14low CD16+ “non-classical” monocytes [163,164]. Similarly, in mice, two populations of monocytes can be described based on the expression of Ly6C, CCR2 and CX3CR1. In mice, “classical” monocytes are characterized by the surface marker com- bination Ly6CHi CX3CR1low CCR2+ (previously termed inflammatory monocytes), while Ly6Clow CX3CR1Hi CCR2− monocytes are defined as “non-classical” (also termed patrolling monocytes). Specific functions have been attributed to the different subsets: non-classical mono- cytes are thought to “patrol” the vessel walls and to have endothelial cell-supporting functions [166,167], while classical monocytes have the capability to cross the endothelium and enter tissues in response to appropriate signals, such as monocyte chemoattractant protein-1 and 3 (MCP-1/MCP-3), contributing to immunological responses [166,168–170]. Historically, monocytes were thought to be mainly precursor cells of macrophages and dendritic cells. However, currently, it is appreciated that in steady-state conditions, most of the tissue-resident macrophages undergo self-renewal [171,172] and, for this reason, the monocyte’s role has been reassessed. It has become clear that blood monocytes can develop in several ways, acquiring a particular antigen-presenting capability or maturing into macrophages. Even though these monocytes show a tissue-resident macrophage signature, it appears that they preserve a degree of their monocyte identity, e.g., Ms4a7 expression , and respond differently during inflammation. In addition, Ly6Chi monocytes can migrate into tissues and retain their monocyte-like state without differentiation into macrophages. These extravascular Ly6Chi monocytes constitute a local monocyte pool with minimal tran- scriptional alterations. Monocyte emigration takes place constitutively in steady-state conditions and is increased during inflammation, when they gain pro-inflammatory prop- erties. Under these circumstances, macrophages that develop from circulating monocytes exhibit first a pro-inflammatory and later an anti-inflammatory phenotype. 3.1. Platelet Interactions with Monocytes/Macrophages As mentioned before, platelets are equipped with receptors that enable them to sense vessel damage, inflammation or infection to become activated. Once activated, platelets can recruit and interact with leukocytes, including monocytes and macrophages, stimu- lating mutual activation and the release of cytokines (Figure 2). Direct platelet–monocyte interactions that lead to the formation of heterocellular complexes was discovered decades ago. Upon activation, P-selectin (CD62P) is exposed on the platelet surface, where it can bind to its counterreceptor on myeloid cells PSGL1. P-selectin/PSGL-1 interac- tions seem to be the first step in platelet–monocyte aggregation. In support of this, a study demonstrated that the conjugation between platelets and monocytes is abolished by block- ing P-selectin. This first association increases the expression of membrane-activated complex 1 (Mac-1) (CD11b/CD18 integrin αM β2 ) on the monocyte surface , further supporting their interactions with platelets, e.g., through the platelet receptor GPIb, thanks to its I domain, which is homologous to the vWF A1 domain and JAM-C. Platelets and monocytes also come in contact through CD40L–CD40 interactions and monocyte- triggering receptors expressed on myeloid cell 1 (TREM-1) to TLT-1 on platelets [183,184]. Upon activation, platelets adhere to monocytes through bridging molecules such as fibrino- gen and thrombospondin [185,186]. Fibrinogen, in this context, has been reported to assist with complex formation, linking Mac-1 on the monocyte surface and integrin αIIbβ3 on platelets [185,187]. In addition to direct interactions, platelet-derived chemokines influence monocyte recruitment and endothelial adhesion and behavior. Platelet CCL5 (RANTES) deposition on inflamed endothelium is relevant for monocyte recruitment. It was also shown experimentally that platelets release large amounts of CXCL4 (PF4) [51,189]. This cytokine enhances monocyte phagocytosis and triggers respiratory bursts via Int. J. Mol. Sci. 2022, 23, x FOR PEER REVIEW 13 of 32 Int. J. Mol. Sci. 2022, 23, 3868 12 of 30 amounts of CXCL4 (PF4) [51,189]. This cytokine enhances monocyte phagocytosis and triggers respiratory bursts via the activation of phosphoinositol-3-kinase PI3K, Syk the activation of phosphoinositol-3-kinase and p38 MAP kinase. Moreover,PI3K, SykCXCL4 platelet and p38induces MAP kinase. Moreover, extracellular signal kinase platelet CXCL4 induces extracellular signal kinase 1 and 2 (ERK1/2) phosphorylation, 1 and 2 (ERK1/2) phosphorylation, which mediates monocyte survival and differentiation which as mediates monocyte well as Janus kinasesurvival and differentiation (JNK) signaling, which leads asto well theas Janus kinase production and(JNK) releasesig- of cy- naling, tokines and chemokines. It was also observed that PF4, in collaboration withItIL-4, which leads to the production and release of cytokines and chemokines. was provokes also observed thatdifferentiation a rapid PF4, in collaboration with IL-4, of monocytes intoprovokes a rapid specialized differentiation cells antigen-presenting of monocytes into specialized antigen-presenting cells (APCs) with (APCs) with unique phenotypical and functional characteristics that are unique phenotypical different from and functional characteristics that are different from macrophages or cDCs macrophages or cDCs. These cells preferentially stimulated the proliferation. Theseof lym- cells phocytes preferentially stimulated the proliferation of lymphocytes and lytic natural killer and lytic natural killer (NK) cell activity, while they induced only moderate (NK) cy- cell activity, while theyRecently, tokine responses. inducedMac-1 only moderate cytokine responses. and proteoglycans Recently, were identified Mac-1the to mediate andbind- proteoglycans were identified to mediate the binding of PF4 to leukocytes. ing of PF4 to leukocytes. Figure 2. Platelet–monocyte interactions and platelet–monocyte complex (PMC) formation. Several Figure 2. Platelet–monocyte interactions and platelet–monocyte complex (PMC) formation. Several factors such as bacterial or viral infection (1) can contribute to the activation of both platelets and factors such as bacterial monocytes (2). Onceoractivated, viral infection (1) and platelets can contribute monocytesto theinteract can activation of both directly platelets through and ex- receptors monocytes pressed(2). Oncesurface, on their activated, andplatelets and monocytes soluble factors can interact released from plateletsdirectly through can further receptors modulate monocyte expressed on their activity surface, (3). This and soluble interaction can factors released from lead monocytes platelets can to extravasate further and modulatetowards differentiate monocyte macro- phages activity (4a)interaction (3). This or alternatively lead can lead to platelet–monocyte monocytes complex to extravasate and formation differentiate (4b).macrophages towards Cell activation is shown (4a) or by yellow alternatively lightnings. lead Plt—platelet,complex to platelet–monocyte Mon—monocyte, formationPMC—platelet–monocyte complex. (4b). Cell activation is shown by yellow lightnings. Plt—platelet, Mon—monocyte, PMC—platelet–monocyte complex. Once deposited, platelet chemokines can form homophilic as well as heterophilic ag- Once deposited, gregates, resulting platelet in the chemokines can formofhomophilic further stimulation as well their biological as heterophilic activity. For example, aggregates, resulting in the further stimulation of their biological activity. RANTES can increase PF4 binding to the monocyte surface, while PF4 drastically en- For example, RANTES canRANTES-induced hances increase PF4 binding to the monocyte monocyte surface, while arrest on endothelial PF4 cells drastically , enhances predominantly medi- RANTES-induced monocyte ated by CCR1. arrest Platelets onassociate also endothelialwithcells , predominantly neutrophils mediated recruit- to promote monocyte by CCR1. ment: Platelets platelet CCL5also associate can with neutrophils form heterodimers to promote with neutrophil monocyte HNP1 recruitment:stim- (alpha-defensin), platelet CCL5 ulating can formadhesion monocyte heterodimers withCCR5 through neutrophil HNP1 and. Alard (alpha-defensin), stimulating that colleagues demonstrated monocyte adhesion through CCR5. Alard and colleagues demonstrated the disruption of HNP1–CCL5 interactions attenuated monocyte and macrophage that the recruit- disruption of HNP1–CCL5 interactions attenuated ment in a mouse model of myocardial infarction. monocyte and macrophage recruitment in a mouse model ofplatelets Activated myocardial wereinfarction. also observed to stimulate monocyte chemotactic protein-1 Activated platelets were also observed (MCP-1) secretion and intercellular to stimulate adhesion monocyte molecule-1 chemotactic (ICAM-1) protein-1 on surface expression (MCP-1) secretion and intercellular adhesion molecule-1 (ICAM-1) surface expression on Int. J. Mol. Sci. 2022, 23, 3868 13 of 30 endothelial cells by activating the NF-κB pathway, thereby indirectly causing monocyte recruitment. Thus, platelet adhesion to the endothelium and chemokines secreted by platelets greatly contributes to subsequent monocyte adhesion to the vascular wall. So far, we have provided a general overview of the events resulting in platelet mono- cyte aggregation, showing how this interaction can lead to changes in cell markers and phenotype and induce mutual cell activation and cytokine production. However, what are the roles of these aggregates? Multiple studies have investigated the mechanism and impact of platelet–monocyte aggregates in a variety of experimental and pathological contexts. Elevated numbers of circulating blood monocyte–platelet complexes were proposed to play a role in the pathogenesis of acute coronary syndromes and atherosclerosis [197,198] and were detected in different diseases, including rheumatoid arthritis and systemic lupus erythematosus , type 1 diabetes and end-stage renal disease suggesting an important role in the pathogenesis of inflammatory diseases. 3.2. Platelet–Monocyte/Macrophages Interaction in Atherosclerosis Atherosclerosis is a chronic inflammatory disease, comprising an intricated vascular in- jury, which represents the initial pathological event for several cardiovascular diseases. In this inflammatory environment, circulating lipoproteins, mainly low-density lipoproteins (LDL), become oxidized (oxLDL). Circulating monocytes are recruited to the atherosclerotic lesion site through P-selectin and E-selectin, intracellular adhesion molecule 1 (ICAM-1) and vascular cell adhesion molecule 1 (VCAM-1). Monocytes migrate into the subendothe- lial space under the influence of chemoattractant molecules, where they differentiate into macrophages in response to locally produced factors such as monocyte colony-stimulating factor (M-CSF). This differentiation includes the upregulation of scavenger receptor A (SR-A) and CD36, which can mediate the uptake of oxLDL. Once macrophages start to phagocytose oxLDL and minimally modified LDL (mmLDL), they transform into foam cells. Foam cells support smooth muscle proliferation, angiogenesis and plaque formation. Finally, the rupture or erosion of vulnerable atherosclerotic plaques leads to arterial thromboembolic events, which may lead to skeletal muscle ischemia or fatal outcomes such as myocardial infarction and stroke. Monocytes and platelets are key players in atherosclerosis. Platelets are consid- ered important drivers of pathogenesis and disease progression thanks to their ability to interact with immune and endothelial cells and through the uptake of LDL. At the site of atherosclerotic plaque formation, platelet activation can be triggered by DAMPs such as oxLDL or podoplanin upregulated on inflammatory macrophages, Th17 T cells and fibroblasts. Specifically, oxLDL has been observed to mediate platelet activation via several pathways, comprising scavenger receptors and platelet-activating factor receptor (PAFR) [208,210]. Platelet PAFR activation by oxLDL occurs simultane- ously with classical agonists, ADP and thrombin for example, initiating prothrombotic responses. It has been observed that platelet adhesion to the endothelium precedes leukocyte recruitment and that blocking platelet adhesion with anti-GPIb antibodies or through the genetic deletion of P-selectin reduces leukocyte recruitment to atherosclerotic lesions and plaque development [211,212]. CD40L-CD40 mediated platelet–monocyte interactions facilitate monocyte arrest on inflamed endothelium, accelerating atherosclerosis [147,213]. Platelets support the migra- tion and activation of monocytes, dendritic cells and neutrophils through the release of soluble inflammatory mediators such as CCL5 and PF4, contributing to the progression of atherosclerosis. CXCL4 promotes phenotypic changes in macrophages, resulting in a proatherogenic state with increased susceptibility to foam cell formation [215,216]. Secondly, PF4 may directly aggravate the atherogenic actions of hypercholesterolemia by promoting the retention of lipoproteins. Sachais and colleagues have recently shown that PF4 facilitates the retention of LDL on cell surfaces by inhibiting its degradation through the LDL receptor. Moreover, PF4 facilitates the esterification and uptake of oxLDL by macrophages contributing to foam cell development. Int. J. Mol. Sci. 2022, 23, 3868 14 of 30 3.3. Platelet–Monocyte/Macrophage Interaction during Infectious Diseases Platelets recognize molecular features of microbes, show bacteriocidic activity and con- tain immunomodulatory mediators essential for alerting and recruiting cells of the immune system. In the following paragraph, platelet–monocyte/macrophage interactions during viral and bacterial infections will be described with a specific focus on platelets and liver macrophages. Circulating platelet–monocyte and platelet–lymphocyte aggregates have been reported in increased numbers in HIV-infected subjects [220,221]. Liang and colleagues found that platelet aggregates with CD16+ inflammatory monocytes are more frequent in HIV- infected patients and associate with increased levels of sCD163, a marker of monocyte activation [222,223]. Platelet aggregates with monocytes, lymphocytes and neutrophils have also been observed during dengue virus infection [224,225]. In 2014, Hottz and colleagues observed that platelet–monocyte complexes correlate with increased vascular permeability in dengue patients. They detected that dengue-activated platelets were able to reprogram monocyte responses ex vivo, inducing the secretion of IL-1β, IL-10 and IL-8.. They also found that the interaction of monocytes with apoptotic platelets mediates IL-10 secretion through phosphatidylserine recognition in platelet–monocyte aggregates. Together, their results demonstrated that activated platelets aggregate with monocytes during dengue infection and signal specific cytokine responses that may contribute to the pathogenesis of dengue. Related to SARS-CoV-2, a study by Hottz and colleagues, performed in 2020, found increased platelet activation and platelet–monocyte aggregates formation in severe COVID-19 patients, compared to those with mild disease. They observed that, in COVID-19 patients admitted to the intensive care unit, platelet–monocyte interaction was strongly associated with tissue factor (TF) expression by monocytes as well as with markers of coagulation exacerbation, such as fibrinogen and D-dimers, and all together correlated with a worse outcome. An increasing number of studies confirms the role of interactions between platelets and monocytes/macrophages in immune responses to bacterial infections. Scull and colleagues