Farm Irrigation and Drainage PDF
Document Details
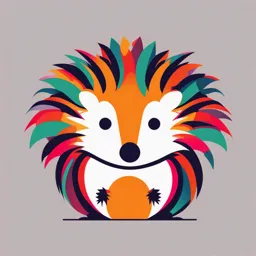
Uploaded by AdroitBoron
University of the Philippines Los Baños
Engr. Leila T. Dominguez
Tags
Summary
This document provides an overview of farm irrigation and drainage, including basic soil and water relations. It details concepts like soil texture, porosity, moisture content, and densities. It also explains various types of irrigation methods.
Full Transcript
PSAE Region IV - Agricultural Engineering Board Review Materials I-1 Farm Irrigation and Drainage Engr. Leila T. Dominguez Instructor III Land and Water Resources Di...
PSAE Region IV - Agricultural Engineering Board Review Materials I-1 Farm Irrigation and Drainage Engr. Leila T. Dominguez Instructor III Land and Water Resources Division Institute of Agricultural Engineering College of Engineering and Agro-Industrial Technology University of the Philippines Los Baños College, Laguna I. Introduction Irrigation is the application of water to create a condition in the soil that is favorable for plant growth. It provides the moisture needed by the crop that is not satisfied by rainfall and refers to the controlled application of water fro agriculture through man-made systems. Drainage, on the other hand, is the removal of excess water from the soil with the same aim of creating conditions favorable for the growth of crops. II. OBJECTIVE OF THE TOPIC Knowledge on farm irrigation and drainage will equip the individual with the understanding of the relation between crops and the amount and timing of both irrigation and drainage. III. DISCUSSION OF TOPICS A. Basic Soil and Water Relations In a soil system of total volume VT and total weight or mass WT: VT = Va +Vw +Vs V V = Va + V w WT = Wa +Ww + Ws where: VT = total or bulk volume of the soil (volume units: cm3, m3) Va = volume occupied by air Vw= volume occupied by water Vs= volume occupied by soil particles VV =volume occupied by voids or void volume WT = total wet weight of the soil (weight units: g, kg) Wa = weight of air (negligible, assumed 0) Ww= weight of water Ws= dry weight of the soil particles Irrigation and Drainage PSAE Region IV - Agricultural Engineering Board Review Materials I-2 For the given soil system, the following properties can be defined: 1. Soil Texture - the relative proportion of primary particles (sand, silt and clay) in the soil 2. Soil Structure - the arrangements of primary particles in the soil into units or peds 3. Porosity (n) – the ratio of the void volume to the total soil volume (unitless) 4. Moisture contents (unitless) a. on a dry weight basis (mcw) – the ratio of the weight of water to the dry weight of the soil b. on a volume basis or volumetric moisture content (mc v) – the ratio of the volume of water to the total soil volume 5. Densities (units: g/cm3, kg/m3) a. Bulk density (ρB) – the ratio of the dry weight of the soil to the total soil volume b. Particle density (ρP) - the ratio of the dry weight of the soil to the volume of the soil particles 6. Specific Gravities (unitless) a. Apparent Specific Gravity (As) – ratio of the bulk density of the soil with the density of water; it is the ratio of the weight of soil to the weight of water with volume equal to the total soil volume b. Real Specific Gravity (Rs) – ratio of the particle density of the soil with the density of water; it is the ratio of the weight of soil to the weight of water with volume equal to the volume of the soil particles alone 7. Depth of water (units: mm, cm, m, inch) a. present in the soil (dw)- the equivalent depth of water in the soil at a given condition dw = mcv x D dw = mcw x As x D Irrigation and Drainage PSAE Region IV - Agricultural Engineering Board Review Materials I-3 where: D – depth of crop root zone or depth or height of soil column under consideration b. needed (dwn) - to increase the moisture content from an initial value (mci) to a final value (mcf) dwn = (mcvf – mcvi) x D dwn = (mcwf-mcwi) x As x D where : mcvf - final soil volumetric moisture content mcvi - initial soil volumetric moisture content mcwf - final soil moisture content on dry weight basis mcwi - initial soil volumetric on dry weight basis 8. Volume of irrigation water (V iw) – volume of water to be applied to increase a the soil moisture content from an initial to final value (units: liters, cm3, m3) Viw = dwn x A where: A – area of the land in consideration NOTE: The density of water, ρw = 1 g/cm3 or 1,000 kg/m3 The following relationships hold true: mcv = mcw x As As = Rs ( 1-n) 9. Infiltration Rate - the time rate at which water will percolate into the soil and can be expressed in terms of the following empirical equations a. Lewis-Kostiakov Equation F = ct ft = dF/dt = ct-1 where: F - cumulative infiltration (mm) t - cumulative time c, - constants ft - instantaneous infiltration (mm/hr or mm/min) b. Horton’s Equation ft = fc + (f0-fc) e –kt where: ft- instantaneous infiltration at time t fc- final or ultimate constant infiltration capacity f0- initial infiltration rate at the beginning of rain or a chosen moment constant c. Philip’s Method F = st1/2 +At ft = dF/dt = (st-1/2)/2 + A where: F - cumulative infiltration (mm) Irrigation and Drainage PSAE Region IV - Agricultural Engineering Board Review Materials I-4 ft - instantaneous infiltration (mm/hr or mm/min) s - soil sorptivity, measure of the capillary or removal of water in a homogeneous soil A - soil parameter that depends on the ability of the soil to transmit water 5. Intake Rate - the rate of infiltration from a furrow into the soil. 6. Permeability - the velocity of flow into the soil caused by a unit hydraulic gradient in which the driving force is one kilogram per kilogram of water. Sample problem #1: A sharp edged cylinder 7.5 cm in diameter is carefully driven into the soil so that negligible compaction occurs. A 12-cm column of soil is obtained. The fresh and dry masses of the soil sample are 910.6 and 736.3 grams respectively. Assume that the particle density of the soil is 2.60 g/cm3. Determine: a. Apparent specific gravity b. Percent porosity c. Soil moisture content by dry weight d. Soil moisture content by volume e. Depth of water present in the soil column Solution: a. b. c. d. e. B. Soil Moisture Constants Irrigation and Drainage PSAE Region IV - Agricultural Engineering Board Review Materials I-5 1. Saturation Point – the amount of water the soil profile will hold when all its pore spaces are filled up with water 2. Field Capacity - (1) the amount of water a soil profile will hold against drainage by gravity at a specified time (usually from 24 to 48 hours) after a thorough wetting. (2) the moisture content of the soil when gravitational water has been removed (after irrigation by flooding). It is usually determined few days after irrigation. The soil moisture tension at this point is normally between 1/10 to 1/3 atmosphere. 3. Permanent Wilting Point (or wilting coefficient) - the soil moisture content when plants permanently wilt. The soil moisture tension at this point is about 15 atmospheres. Permanent wilting percentage can be estimated by dividing the field capacity by a factor ranging form 2.0 top 2.4, with the value higher for soils with higher silt content. 4. Available Moisture (AM) - the difference in moisture content of the soil between field capacity and the permanent wilting point. 5. Readily Available Moisture (RAM) - that portion of the available moisture that is most easily extracted by plants; this is approximately 75% of the available moisture. 6. Computation of Moisture Content – the amount of moisture present in the soil (mc) given the percent AM content retained or used and the FC and PWP moisture contents of the soil can be computed using the equations: a. mc = FC – % AM used x (FC-PWP) b. mc = PWP + % AM retained x (FC-PWP) Sample problem #2: What is the present moisture content of a soil with 25% of its AM has been used if its FC is 24% and PWP is 16% by weight? Solution: mcw = FC – % AM used x (FC-PWP) = 0.24 - 0.25(0.24-0.16) = 0.24 - 0.25(0.08) = 0.24 - 0.02 = 0.22 = 22% by weight C. Irrigation Efficiencies 1. Water Conveyance Efficiency - the ratio between the water delivered to the farm and the water diverted from a river or reservoir expressed in percent. 2. Water Application Efficiency - the ratio between water stored in the soil root zone during irrigation and the water delivered to the farm expressed in percent. 3. Water-use Efficiency - the ratio of water beneficially used on the project, farm or field to the amount of water delivered to the farm expressed in percent. Irrigation and Drainage PSAE Region IV - Agricultural Engineering Board Review Materials I-6 4. Water Storage Efficiency - the ratio of water stored in the root zone during the irrigation to the water needed in the root zone prior to irrigation, expressed in percent. 5. Consumptive Use Efficiency - the ratio of the normal consumptive use of water to the net amount of water depleted from the root zone soil. D. Pump Irrigation 1. Water horsepower - the power theoretically required to lift a given quantity of water each second to specified height. 2. Brake Horsepower - water horsepower divided by pump efficiency, in decimal. 3. Static Head - the difference in elevation of the water surface in a pond, lake, or river from which pumped water is taken, and the water surface of the discharge canal into which the water flows from a submerged discharged pipe. In pumping from groundwater source, static head is the difference in elevation between the water surface in the well and the water surface of the discharged canal. 4. Total Dynamic Head - the sum of total static head, pressure head, velocity head and friction head. 5. Drawdown - (in a well) is the difference in elevation between the groundwater table and the water surface at the well when pumping. 6. Characteristic Curve - graphs that show interrelations between speed, head discharge, and horsepower of a pump. 7. Specific Speed - expresses the relationship between speed in rpm, discharge in gpm, and head in feet. E. Irrigation Principles 1. Evapotranspiration - is the sum of transpiration and water evaporated from the soil, or exterior portions of the plants where water may have accumulated from irrigation, rainfall, dew, or exudation from the interior of the plants. Consumptive use is identical with evapotranspiration, for practical purposes. Consumptive use only includes water retained in the plant tissue. 2. Transpiration - the process by which water vapor escapes from living plants, principally by leaves, and enters the atmosphere. 3. Canal Capacity The ability of the stream to provide water determines the extent of the total service area of a national irrigation system. To compute for the service area, the stream’s dependable flow (to a certain percent of dependability) is divided by the diversion water requirement. In equation form it can be stated as: Irrigation and Drainage PSAE Region IV - Agricultural Engineering Board Review Materials I-7 The design of irrigation canals, whether main, secondary or tertiary is primarily based on its expected capacity. The canal capacity (Q) refers to the amount of water it can deliver at a unit time and is dictated by total area it serves and the area water requirement. In equation form: Q (m3/s) = area (ha) x water requirement (m3/s-ha) The capacity of a main and secondary canal is computed using the diversion water requirement while that of a tertiary canal is based on the farm water requirement. Both capacities are reflective of the area they serve. 4. Leaching Requirement (LR) - the fraction of the irrigation water that must be leached through the root zone to control soil salinity at specified level. where: Ddw – depth of drainage water Diw - depth of irrigation water Drw - depth of rain water EC(iw+rw) - weighted average of the electrical conductivity of the irrigation and drainage water ECiw - electrical conductivity of irrigation water ECiw- electrical conductivity of rain water ECdw- electrical conductivity of drainage water 5. Crop Water Requirement (CWR) The crop water requirement of an area grown to a certain crop refers to the amount of water used for the non-consumptive demands such as land soaking and land preparation, and for the consumptive demands such as evapotranspiration requirements of the crop during its entire growth period. Non-consumptive uses The amount of water needed for the non-consumptive uses depends on the type of crop being grown, type of soil, climatic conditions and farming practices and techniques. The non-consumptive uses include seepage and percolation losses. Seepage refers to the lateral movement of water along the soil profile while percolation which is highly dependent on soil texture, refers to the downward movement of water through a depth of soil. Consumptive uses The consumptive use of crop includes evaporation and transpiration, lumped together as evapotranspiration. Evaporation accounts for he quantity of water evaporating from soil and water surfaces as well as that from plant part Irrigation and Drainage PSAE Region IV - Agricultural Engineering Board Review Materials I-8 surfaces. Transpiration the water absorbed by the plant through its roots and used in building plant tissues or released into the atmosphere. 6. Irrigation Water Requirement (IWR) The irrigation water requirement is the amount of water to be applied to the field as irrigation. It can be computed by deducting the effective rainfall (ER) from the total crop water requirement. In equation form it is: IWR = CWR-ER 7. Farm Water Requirement (FWR) From the tertiary canal to the field, application losses are incurred. Application losses include the seepage and percolation losses along the canals as well as losses due to evaporation. To account for these losses, the design farm requirement is computed with the application efficiency in consideration. Application efficiency is the ratio of the amount of water entering the tertiary canal and the amount of water that reaches the field. With the losses, the application efficiency (ea) of the tertiary canals becomes less than one. In equation form: where: ea = application efficiency Qin = water entering the tertiary canal Qout = water exiting the tertiary canal or water entering the field S = seepage losses P = percolation losses E = evaporation losses The design farm water requirement can be computed with the equation: 8. Diversion Water Requirement (DWR) Similar to that of tertiary canals, the main systems (main and secondary canals) also incur losses termed as conveyance losses. Seepage and percolation losses and evaporation along the conveyance canals comprise the conveyance losses. To account for these losses, the design diversion water requirement is computed with the conveyance efficiency in consideration. Conveyance efficiency is the ratio of the amount of water entering the main canal and the amount of water that reaches the tertiary canal. With the Irrigation and Drainage PSAE Region IV - Agricultural Engineering Board Review Materials I-9 losses, the conveyance efficiency (e c) of the main systems becomes less than one. In equation form: where: ec = conveyance efficiency Qin = water entering the main canal Qout = water exiting the secondary canal or water entering the tertiary canal S = seepage losses P = percolation losses E = evaporation losses The design diversion water requirement, which is the quantity of water to be obtained from the source, can be computed by: where: DWR = design diversion water requirement FWR = design farm water requirement ec = conveyance efficiency F. Modes of Irrigation 1. National Irrigation Systems (NIS) – irrigation systems that have relatively large service areas and are managed by government agencies 2. Communal Irrigation Systems (CIS) – managed and operated by farmers’ or irrigators’ associations 3. Shallow Tubewell Irrigation Systems (STW) – pipes vertically set into the ground that abstract groundwater to be used for irrigation, usually owned and operated by individual farmers G. Methods of Irrigation Irrigation water can be applied to the uplands in any of the following general ways: 1. by overhead irrigation, wherein the soil is moistened in much the same way as rain a. Watering can. It is the simplest piece of overhead irrigation equipment and is commonly used in small-scale upland farming. Since the water is carried by hand, this method is limited to small plots with easily accessible source of water. The size of the plot depends largely upon its distance from the source, and the time that it takes to fill the can at the source. b. Hose pipe. This method can be used if there is a piped water- distribution system where a hose pipe can be connected to a tap or outlet and there is enough pressure in the water as it emerges from the hose pipe. A major disadvantage of this system is likely to be the cost of the water supply. Irrigation and Drainage PSAE Region IV - Agricultural Engineering Board Review Materials I-10 c. Sprinkler Irrigation. This method is the application of water to the surface of the soil in the form of spray, simulating that of rain. The spray is produced by the flow of water under pressure through small orifices or nozzles. Pumping usually provides the pressure. With careful selection of nozzle sizes, operating pressure, and sprinkler spacing, the amount of irrigation water required to refill the crop-root zone can be applied nearly uniformly at a rate to suit the infiltration rate of the soil to obtain efficient irrigation. 2. furrows, which wets only a part of the ground surface a. Furrow irrigation. It is accomplished by running water through small channels or furrows while it moves down or across the slope of the field. The water sips into the bottom and sides of t he furrows to provide the desired wetting. Careful land grading for uniform slopes is essential with this method. b. Corrugation irrigation. It is a variation of the furrow method and it uses small rills or corrugations for irrigating closely spaced crops, such as small grains and pastures. The water seeps laterally through the soil, wetting the area between the corrugations. 3. by flooding, which wets the entire land surface a. Ordinary flooding. Water is applied from field ditches to guide its flow and it is difficult to attain high irrigation efficiency using this method. The chief advantage of this method is its low initial cost of preparing the land. b. Border-strip flooding. A field is divided into a series of strips by borders or ridges running down the predominant slope or on the contour. To irrigate, water is released into the head of the border. The water, confined and guided by two adjacent borders, advances in a thin sheet toward the lower end of the strip. The objective is to allow a sheet of water to advance down the narrow strip of land, allowing it to enter the soil as the water advances. c. Level-border or basin irrigation. Water is supplied to level plots surrounded by dikes or levees. This method is particularly useful on fine-textured soils with low permeability, it is necessary to hold the water on the surface to secure adequate penetration. d. Contour-ditch irrigation. It involves controlled flooding from field ditches along the contour of the land, which allows the water to flood down the slope between field ditches without employing dikes or other means that guide or restrict its movement. 4. by drip or trickle irrigation wherein the water is directed to the base of the plant. Water is applied to the soil through small orifices. The small orifices, often called emitters, are designed to discharge water at rates of 1 to 8 liters per hour. Water is delivered to the orifices through plastic pipelines, which are generally laid on the soil surface or buried. The rate of discharge is determined by the size of the orifice and the pressure in the pipelines. This method is particularly beneficial for young orchards, vineyards, closed-spaced perennials, and other crops of high value and in areas where water is scarce or Irrigation and Drainage PSAE Region IV - Agricultural Engineering Board Review Materials I-11 has a high salt content. A highly efficient water utilization can be achieved with this method, bit it is very expensive. 5. by sub-irrigation, wherein the surface is rarely wet since the water is supplied from the soil underneath. This requires complete control of the water table so that the root zone is kept relatively free of excess water but is continually supplied with capillary moisture during the cropping season. H. Head computations for a Sprinkler Irrigation System The design capacity for sprinklers on a lateral with uniform spacing should be based on the operating pressure. From the chosen sprinkler operating pressure (from the catalog), the pressure or head Hn required at the junction of the lateral and the main can be computed using the equation: Hn = Ho + Hf ± He + Hrp Where: Hn = pressure or head required at the junction of the lateral and the main Ho= nozzle pressure at the farthest end of the line (sprinkler operating pressure) Hfl = friction head loss in the lateral He = maximum difference in elevation between the junction with the main and the farthest sprinkler on the lateral Hrp = riser height If the lateral is located downhill from the main, the elevation term is negative. If the lateral is located uphill from the main, the elevation term is positive. 1. Friction or head loss in pipes a. for main lines where: Hfm = total friction loss in the main line, in meters Ks = Scobey’s coefficient of retardation (0.32 for new Transite pipe, 0.40 for steel pipe or portable aluminum pipe and couplers, and 0.42 for portable galvanized steel pipe and couplers) L = length of the pipe in m Q = total discharge in L/s D = inside diameter of pipe in mm b. for laterals where: Hfl = total friction loss in the main line, in meters F = correction factor from Table 1 Irrigation and Drainage PSAE Region IV - Agricultural Engineering Board Review Materials I-12 Table 1. Correction factor F for Friction Losses in Aluminum Pipes with Multiple Outlets. Correction Factor, F Number of First Sprinkler One Sprinkler First Sprinkler One Sprinkler Sprinklers Interval from Main Interval from Main 1 1.00 1.00 2 0.63 0.51 4 0.48 0.41 6 0.43 0.38 8 0.41 0.37 12 0.39 0.36 16 0.38 0.36 20 0.37 0.35 30 0.36 0.35 Source: Schwab, G. O., et al.(1992) Soil and Water Conservation Engineering. 4th ed. 2. Pump head In selecting a suitable pump, it is necessary to determine the maximum total head against which the pump is working. Ht = Hn + Hfm + Hj + Hs Where: Ht = total design head against which the pump is working Hn = pressure or head required at the junction of the lateral and the main Hfm = total friction loss in the main line Hj = elevation difference between the pump and the junction of the lateral and the main Hs = elevation difference between the pump and the water supply after drawdown, all in m or kPa I. Drainage - removal of excess water in the soil to create conditions suitable for plant growth. Israelsen (1962) noted that adequate drainage: 1. improves soil structure 2. the productivity of soils 3. facilitates early plowing and planting 4. lengthens the crop growing season 5. increases the depth of root zone soil thereby provides more available soil moisture and plant food 6. improves soil ventilation 7. increases water infiltration into soils 8. favors growth of soil bacteria 9. leaches excess salts from the soil J. Sources/Causes of excess water: 1. rainfall 2. high water table 3. over-irrigation 4. runoff/seepage from adjacent farms Irrigation and Drainage PSAE Region IV - Agricultural Engineering Board Review Materials I-13 K. Components of a Drainage System: 1. field drainage system (quarternary and tertiary or collector drains) 2. secondary drains or laterals 3. outfall 4. recipient water L. Types of Surface Field Drainage System or Open Drains 1. Bedding System. The soil type largely influences the width of bed to be used. The furrows drain to collection ditches. 2. Random Ditch System. This system is adapted to areas that have depressions which are too deep or too large to fill by land leveling. The ditches meander from one low spot to another, collecting the water and carrying it to an outlet ditch. 3. Interception or Cross-slope System. This resembles terracing in that the drainage ditches are constructed around the slope on a uniform grade according to the land topography. The ditches should be constructed across the slope as straight and parallel s the topography permits. 4. Diversion or Parallel Ditch System. This is suitable on flat, poorly drained soils that have numerous shallow depresssions. In general, the ditches are 185 m to a maximum of 370 m apart (not necessarily equidistant) and the land in between the parallel ditches is sloped and smoothed to eliminate any minor depressions or obstructions to the overland flow of the water. M. Layout of a Tile-Drain System or Closed Drains 1. Natural System. This system is used in rolling topography where drainage is necessary only in small valleys. 2. Gridiron Layout. Used if the entire area is to be drained and is usually more economic. Laterals enter the submain from one side only to minimize the double drainage that occurs near the submain. 3. Herringbone Pattern. The submain is laid in a depression and the laterals join the submain from each side alternately. The land along the submain is double drained, but since it is in a depression, it probably requires more drainage. 4. Double-main System. This system is often used if the bottom of the depression is wide since it reduces the lengths of the laterals and eliminates the break in slope of the laterals at the edge of the depression. 5. Intercepting Drain. This is used if the main source of excess water is drainage from hill lands. The drains are placed along the toe of the slope to protect the bottom land. 6. Arrangement to avoid trees. This system is adopted to minimize the exposure of the laterals to the hazard posed by root of trees which easily enter the open joints of underdrains. Mains and laterals were kept well away from trees. N. Drain Depths and Spacing In highly permeable soils underlain by an impervious layer or compact clay of low permeability, the groundwater flow is essentially horizontal towards the Irrigation and Drainage PSAE Region IV - Agricultural Engineering Board Review Materials I-14 drains. To simplify the illustration, the source of water flowing toward the drain is considered a reservoir as shown in the following figure. Ground surface Drain open or closed Dwt Sandy soil Water Surface Saturated hf Sandy soil Water reservoir H (H+h) 2 h R Clay of low permeability Linear flow of groundwater (for sandy soils over clay) toward drains spaced 2R in which the water table midway between the drains is H-h above the water surface drain. Adapted from Irrigation Principles and Practices by Israelsen and Hansen The water surface is maintained in the reservoir and adjoining soil a distance of H above the clay. Flow from the reservoir to the drain is steady, and it is assumed that the reservoir is the only source of water. Groundwater flows to the drain from both sides. Let 2q be the flow into a drain in length L. Then the groundwater flow from one side to the drain is q = av and from Darcy’s Law, Consider the depth of saturated sand about midway between the reservoir and the drain as average; then the average area of saturated soil, in drain length L, through which the groundwater flows is: and the quantity of flow from the reservoir to the drain The quantity of flow to the drain from the reservoir on both sides would be: Irrigation and Drainage PSAE Region IV - Agricultural Engineering Board Review Materials I-15 from which: and since drain spacing S equals 2R Some Irrigation Terms as defined by the Republic Act 8435 or The Agriculture and Fisheries Modernization Act of 1997 1. Communal Irrigation System (CIS) - an irrigation system that is managed by a bonafide Irrigators’ Association 2. Headworks - the composite parts of the irrigation system that divert water from natural bodies of water such as rivers, streams, and lakes 3. Irrigable Lands - lands which display marked characteristics justifying the operation of an irrigation system 4. Irrigated Lands - lands serviced by natural irrigation or irrigation facilities. These include land where water is not readily available as existing irrigation facilities need rehabilitation or upgrading or where irrigation water is not available year-round 5. Irrigation System - a system of irrigation facilities covering contiguous areas 6. Irrigators’ Association (IA) - an association of farmers within a contiguous area served by a National Irrigation System or Communal Irrigation System 7. Main Canal - the channel where diverted water from a source flows to the intended area to be irrigated 8. National Irrigation System (NIS) - a major irrigation system managed by the National Irrigation Administration 9. On-Farm Irrigation Facilities - composite facilities that permit entry of water to paddy areas and consist of farm ditches and turnouts 10. Secondary Canal - the channel connected to the main canal which distributes irrigation to specific areas 11. Shallow Tubewell (STW) - a tube or shaft vertically set into the ground for the purpose of bringing groundwater to the soil surface from a depth of less than 20 meters by suction lifting DRAIN SPACING EQUATIONS Irrigation and Drainage PSAE Region IV - Agricultural Engineering Board Review Materials I-16 A. STEADY STATE: I. Drain Bottom Reaching Impermeable Layer (DBRIL) 1. with recharge, unconfined aquifer condition, drain levels of same elevation where: L – drain spacing k- soil hydraulic concudtivity H- depth of water midway between the drains D- depth of water inside the drains R- net recharge into the area to be drained 2. no recharge, unconfined aquifer condition, drain levels of unequal elevation (unidirectional flow to lower level) where: h1, h2 – water depths at the drains q- flow into the drain per unit drain width 3. no recharge, confined aquifer condition, drain levels of unequal elevation (unidirectional flow to lower level) where: b- thickness of confined auifer (land strip to be drained) 4. with recharge, unconfined aquifer condition, drain levels of equal elevation a. flow to left drain, qL b. flow to right drain, qR c. elevation of the water table (h) at any point x d. stagnation point , xs( x where dh/dx = 0, also x where h= hmax) Irrigation and Drainage PSAE Region IV - Agricultural Engineering Board Review Materials I-17 e. maximum elevation of the water table (hmax) II. Drain Bottom Not Reaching Impermeable Layer (DBNRIL) Hooghoudt’s Equation (PIPE DRAINS) where: k1- hydraulic conductivity of unsaturated soil k2 - hydraulic conductivity of unsaturated soil d – effective depth of pipe drains UNSTEADY STATE Glover-Dumm Formula reaction factor α has the following equation where : μ – drainable pore space (m3/m3) ho – height of water midway between the drains at time=0 ht- height of water midway between the drains at time t t – time to drain References: Israelsen, O.W and V.E Hansen (1962). Irrigation Principles and Practices.3rd ed. JohnWiley and Sons, Inc. New York, U.S.A. Linsley, R. K. et al. Hydrology for Engineers. Mc Graw-Hill, Inc. U.S.A. Irrigation and Drainage PSAE Region IV - Agricultural Engineering Board Review Materials I-18 Linsley, R.K. et al (1992). Water Resources Engineering.4th ed. Mc Graw- Hill, Inc. U.S.A. Schwab, G. O., et al.(1992) Soil and Water Conservation Engineering. 4th ed. JohnWiley and Sons, Inc. New York, U.S.A. Irrigation and Drainage