Materials Selection (ID 2322 Week 3) PDF
Document Details
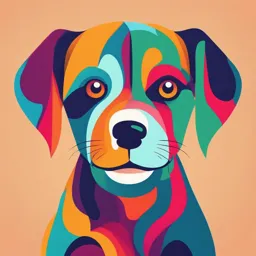
Uploaded by ObtainableChlorine1747
National University of Singapore
Tags
Summary
These notes cover materials selection, focusing on material properties, fabrication techniques, service conditions, and economic considerations. The document includes examples, definitions, and tables related to the topics.
Full Transcript
ID 2322 Materials & Production Week 3 national university of singapore DIVISION OF INDUSTRIAL DESIGN College of Design and Engineering BA(ID) - Level 2 SEMESTER 1 Module Leader - Kouo Wai Chiau...
ID 2322 Materials & Production Week 3 national university of singapore DIVISION OF INDUSTRIAL DESIGN College of Design and Engineering BA(ID) - Level 2 SEMESTER 1 Module Leader - Kouo Wai Chiau email : [email protected] Properties of Materials (Week 3) Physical properties Mechanical properties Technological properties Chemical properties Economic properties Aesthetic properties Properties of Materials Different engineering materials have different properties. In selecting a material for a particular application, consideration has to be made to match the properties of the materials to the required service conditions. For example, copper is used for electrical wires because of its excellent electrical conductivity. Likewise, polyethylene is used as wire coating material because of its good electrical insulation and flexibility. Properties of Materials In general, there are 6 major groups of properties that are to be considered when selecting a material for a specific application. These are: a) Physical properties e.g. density, melting point, thermal conductivity, thermal coefficient of expansion, electrical resistivity, etc. b) Mechanical properties e.g. strength, hardness, toughness, creep resistance, fatigue resistance, etc. Properties of Materials c) Technological properties e.g. machinability, castability, forgeability, weldability, formability, brazeability, etc. d) Chemical properties e.g. resistance to oxidation, corrosion and solvents. e) Economic properties e.g. cost of raw materials and manufacturing, time for fabrication, availability of materials, etc. f) Aesthetic properties e.g. appearance, feature, surface texture and ability to accept special finishing, etc ENGINEERING PROPERTIES OF MATERIALS Traditionally engineers are responsible for the material behavior and its properties. However, it is important for the industrial designer to know at least the basic terms for describing materials. Why? Some of the reasons are: Talking the same language with engineers. Able to work alone if needed when designing a simple product. Understanding basics about materials’ properties and how materials behave will give the designer some insight into why certain shapes work best for a given application and how to get the best performance out of a material. PHYSICAL PROPERTIES Brief Definitions of Physical Properties Physical properties are inherent aspects of a material that are generally not easily altered. Physical properties generally remain intact, whereas mechanical properties can be changed through heat treatment. DENSITY ρ (rho) Density = Mass/Volume, is a characteristic of a given material. It has the unit of g/cm3 or kg/m3. The density of a material is related to the structure or the packing arrangement of the atoms. OPACITY / TRANSPARENCY Ability to transmit light. COLOUR Colour: Inherent reflected wavelength. PHYSICAL PROPERTIES MELTING POINT Melting point of a material refers to the temperature at which the material changes from solid to liquid states. It can be expressed either in terms of °C, °F or °K. THERMAL CONDUCTIVITY The ability of a material to conduct heat within and through a material is called thermal conductivity. It may be expressed in terms of W/(m°K). Copper is said to possess high thermal conductivity (401 W/mK) as it offers little resistance to the passage of heat while wood (with conductivity in the region of 0.15-0.04 W/mK) resists the passage of heat and is said to be a poor heat conductor. Thermal conductivity Material Thermal conductivity(W/mK) at 25°C Silver 429 Copper 401 Aluminum 205 Copper CPU Cork board 0.07 Heat Sink Air 0.024 PHYSICAL PROPERTIES ELECTRICAL CONDUCTIVITY σ sigma(S/m) The ability of a material to conduct current is called electrical conductivity. It is expressed as siemens per meter (S/m) in SI units. For example, copper has an electrical conductivity of 5.96 x 107 S/m while PVC has 1 x 10-12 S/m. The electrical conductivity is closely related to thermal conductivity. For example, silver is both a good heat and electrical conductor. Electrical conductivity Silver Relative Conductivity = 106 Copper( Annealed) Relative Conductivity = 100 Gold Relative Conductivity = 65 Aluminium Relative Conductivity = 63 Steel Relative Conductivity = 3 -15 Copper, for example, is used to make the wire which goes inside electrical cables. Copper was chosen because it can be drawn into long thin wires very easily (it is ductile) and because it is a good conductor of electricity. Thermal expansion Thermal expansion: Expressed in units of l / F or l / C. Generally, the coefficient of thermal expansion is inversely proportional to the melting point of a material – higher melt- temperature materials have less expansion. Steel is a significant exception. Magnetic/nonmagnetic/ferromagnetism: Alignment of iron, nickel, and cobalt atoms into domains. Corrosion resistance: Ability to resist surface deterioration caused primarily by oxygen, chemicals, or other agents. (Degradation in plastics can also be caused by ultraviolet light, moisture, and so on.) MECHANICAL PROPERTIES MECHANICAL PROPERTIES STRENGTH In materials, there are 3 common forms of strength: tensile, compressive and shear. The strength of materials is expressed in terms of kgf/mm2 or N/ mm2 (Mpa). This is called unit stress (symbolized as σ Sigma) and is the applied load per unit area. MECHANICAL PROPERTIES STRENGTH When stress is applied to a material, it changes shape. For example, a metal in compression will be shortened but stretched longer while in tension. This change in shape is called strain (symbolized as ε epsilon) and is expressed as millimetre of deformation per millimetre of material length. Strain Strain is the change a material undergoes during elongation or contraction. It is given as a measure of deformation under load. Elasticity Elasticity describes the recovery of a material back to its original shape and size after being deformed, when a stress is removed. The deformation of a good elastic material is not permanent. Rubber is an elastic material. A rubber band can be stretched and return to its original length after pulling force is removed. But if the force exceeds its elastic limit, then the rubber band snaps or ruptures. But there is no change in the cross section of the rubber band-there is no plastic deformation. Plastic behavior Plastic behavior is quite different from elastic behavior. A good example of pure plastic deformation can be demonstrated by bubble gum. When gum is stretched, it deforms and the cross section changes - it thins out. This is called necking. When the force is relaxed, the gum does not return to its original shape. This is called plastic deformation (Figure 3-3). MECHANICAL PROPERTIES Steel stress strain curve TENSILE PROPERTIES The behaviour of materials subjected to tensile load can be described in terms of their stress-strain graphs. Fig.1 shows a typical stress-strain graph ( for a ductile material.) Universal tensile tester Material test pieces MECHANICAL PROPERTIES Steel stress strain curve With reference to Fig.1, an increase in stress causes a corresponding linear increase in strain until point A (named as proportional or elastic limit). In other words, when a material is stressed within this elastic region, the material will recover its deformation and return to its original shape if the stress is removed. MECHANICAL PROPERTIES As the applied stress is increased to beyond point A, the linear line changes into a curve. This change occurs at the yield stress, point B. Here, the material exhibits permanent deformation. Beyond point B, the material continues to deform to point C even where there is no appreciable increase in stress. Points B and C are called upper and lower yield points respectively. In practice, the yield point refers to upper yield point. MECHANICAL PROPERTIES Further increase in the applied stress leads to point D, the maximum allowable stress before fracturing occurs at point E. The point D is known as maximum tensile stress or simply the tensile strength. MECHANICAL PROPERTIES Different materials will exhibit different tensile behaviour. Fig.2 shows the tensile curves for a series of common engineering materials. It is seen that apart from the ductile material such as the mild steel, many materials do not exhibit a marked discontinuity called yield point. In fact, their tensile curves merely show a smooth transition from elastic to plastic regions. The cast iron and aluminium, as shown in Fig.2, exhibit this form of behaviour. Fig. 2 The following terminology are commonly encountered in the study of materials: a) Yield strength (y) The stress level at which large strains take place without further increase in stress. It is also the maximum stress at which a material can with stand before plastic flow (permanent deformation) sets in. b) Proof stress Aluminum stress strain curve Somewhat equivalent to yield strength. In fact, this is the data that is quoted to denote yield strength since the latter is always not apparent from actual practical testing. Fig. 3. MECHANICAL PROPERTIES c) Elasticity The ability of a material to undergo elastic deformation and then recover upon removal of stress. d) Plasticity The ability of a material to undergo permanent deformation without rupture. e) Modulus of elasticity (E) This is a measure of the stiffness of a material. It is the ratio of stress to strain at elastic limit. In terms of the stress-strain graph, the modulus of elasticity is the slope of the stress-strain curve in the range of linear proportionality of stress to strain. MECHANICAL PROPERTIES f) Tensile strength Also known as ultimate tensile strength (UTS). It is the maximum stress in which a material can sustain without fracture and is determined by dividing the maximum load to the original cross- sectional area of the material under test. ( proof stress ) Modulus of elasticity (E) Stress-strain curve typical of a low carbon steel. Compression Compression is a measure of the extent to which a material deforms under a compressive load prior to rupture. Again, like a warm bubble gum, no matter how hard you squeeze it or step on it, it flattens but does not rupture. A building foundation requires a strong material with outstanding compressive strength - such as concrete. Bus stop is made of concrete. Road surface is asphalt. Concrete has good compressive strength, but under a very heavy load, it will crack. It is brittle. Bending Bending is characterized by the outside fibers of a beam being placed in tension and the inside fibers in compression. Torsion Torsion is the application of torque to a member to cause it to twist about its longitudinal axis. Crankshaft and propeller shaft must be made of metal with superior torsional strength or they will fail under the twisting stress they are subjected to. Shear strength Shear strength is the maximum load a material can withstand without rupture when subjected to a shearing action. Bubble gum has very little shear strength; it will shear very easily. Shear strength Ductility Ductility is the ability of a material to withstand plastic deformation by tension without rupture(or fracturing). The bubble gum is a good example. As it is chewed it does not break up but is molded by the teeth into a new shape, a new form. Ductility is important in discussing bendability and drawability in solid-state forming. Pull DUCTILITY Ductility refers to the ability of a material to undergo plastic deformation, by tension, without fracturing. Low carbon steels, aluminium, copper, gold, silver and nickel are typical examples of ductile metals. Ductility may be measured by means of a tensile test during the determination of other tensile parameters of a material. DUCTILITY The specimens are measured for length or cross-sectional area between gauge marks before and after the test. For instance, with reference to Fig. 4. A high calculated % from the expressions indicates a high ductility MALLEABILITY Both ductility and malleability are referred as the ability of a material to deform plastically without fracture. However, malleability is associated with compressive deformation while ductility, tensile. Deformation processes such as bending, forging, rolling and extrusion will rely on the malleability rather than the ductility of a material. On the other hand, drawing a wire will call upon the ductile property of a material. MALLEABILITY In general, the metals that are ductile are also malleable. However, some metals that are malleable may not be ductile. For example, tin, gold, silver, copper and pure iron are both ductile and malleable but lead is not ductile although it is malleable. Hardness Hardness: the ability of a material to withstand penetration, scratching or wear. Hardness and brittleness are related. Clay is not hard, a steel ball bearing dropped on it will penetrate into the clay. But a ball bearing will not penetrate the surface of glass. If the ball bearing hits the glass surface with great force, it will shatter the glass. HARDNESS If we hit a block of copper with a hammer, the block elongates and a dent of the shape of the hammer head can be seen on the copper block. The hammer is said to be harder than the copper block. HARDNESS The hardness values are generally expressed in terms of a scale associated with a particular test. There are few scales that are commonly used to measure the hardness values: 1) Brinell The first 3 are the common 2) Vickers scales that are often used 3) Rockwell to express the hardness 4) Knoop values of engineering 5) Scleroscope materials. Brittleness Brittleness is the opposite of ductility. If bubble gum is frozen, it becomes brittle and can break your teeth or shatter if you hit it with a hammer. Glass is a good example of a brittle material. BRITTLENESS Brittleness is the opposite of ductility. A brittle material is one that will be fracture with no plastic deformation. It is not related to tensile strength. Thus a high tensile steel is more brittle than a mild steel. In general, brittleness is associated with extreme high hardness. Typical examples of brittle materials are hardened steels, cast irons and ceramics. IMPACT STRENGTH This property is associated with the toughness of a material. Toughness depends mainly on the ductility and strength of a material. The property is commonly determined by impact test. There are 2 main forms of impact test - the Charpy and the Izod. These two forms of test differ from one another in the shape of the specimens and the way in which the specimens are held in the impact tester. IMPACT STRENGTH In Charpy test, the specimen is supported freely by its ends in a horizontal position. It is struck by a pendulum at a point behind the notch that is located at the middle of the span. On the other hand, the specimen for the Izod test is gripped vertically in a vice and the specimen is struck just above the notch. Charpy test Izod test IMPACT STRENGTH In both tests, a heavy pendulum swings through an arc and strikes a notched test piece. After breaking the specimen, the pendulum continues its swing but as some energy is used to break the specimen, the pendulum does not swing back to the same height as that from which it is started. The difference in height is a measure of the energy absorbed in the breaking. IMPACT STRENGTH FATIGUE STRENGTH In service, many structural assemblies undergo thousands of changes of vibrational stresses. If these vibrations are of sufficient magnitude, fracture can still occur even when the stress executed is less than that determined by a tensile test. For example, when a wire is bent back and forth many times, it is subjected to repeated stresses. The wire breaks easily after a few times of bending. On the other hand, the wire will last for a long time under an applied load when there is no change in the direction of stress. This form of fracture which occurs as a result of repeated or cyclic stressing is called fatigue failure. FATIGUE STRENGTH Fatigue failures are common in many machine parts. Typical examples are shafts; connecting rods; gears; crane hooks; bolts and aircraft bodies. Fatigue test on chair at IKEA CREEP RESISTANCE When a material is loaded for a long period of time, it may gradually deform and fracture at a stress well below the tensile stress as determined by a tensile test. This form of plastic deformation of materials as a result of prolonged stressing, usually accompanied by high temperatures, is called creep. CREEP RESISTANCE Creep is common in materials that are required to function at high temperatures as in the case of the turbine blades and the boiler tubes. CREEP RESISTANCE CREEP RESISTANCE However, creep can also occur in structural beams after a prolonged period of service. The working table of a milling machine may creep under its weight if it is not brought back to its original position at the end of a day. FURTHER READINGS 1. W. Bolton, “Engineering Materials Technology”, Chaps 1 & 5. Heinemann Newnes, 1992. 2. R.L.,Timings, “Engineering Materials” v. 1, Chap. 11, Longman, 1989. Thank you Materials Selection OBJECTIVE: At the end of this lesson, the students should be able to: ❑ Understand how product design, material and manufacture are inter-depended during the course of materials selection. ❑ Consider the following criteria for selection: the performance of materials the response of materials towards fabrication the conditions in which the materials are put into service the economic influence Materials Selection 1) Introduction 2) Materials 3) Fabrication processes 4) Service conditions 5) Economic requirements 1) INTRODUCTION The selection of an engineering material for a specific application is an extremely difficult task. There are many thousands of different materials available for selection and with a conservative figure of 20 data per material, there may be between 3xl06 to 3xl07 pieces of material data to cope. In addition to this vast pool of data, the proper selection of a material to fit into a product design is not an exercise which can be carried out in isolation. How would you shop for a Laptop? You will likely to looking into areas like: size, appearance, Performance, after sale support and cost. You will likely to look at several brands and rate each in various categories and then make a selection. The goal is usually the computer that will provide the best service at an affordable price. Material selection should be approached in this same manner. 1) INTRODUCTION Selection of a material must involves consideration of several factors. To these, different views were proposed in textbooks and journals. Nevertheless, one can consider the selection of materials as a inter- dependent consideration between the 3 basic elements: product design, Fig.l material and manufacture, Fig. 1. Gold for a pair of shoe? Crafted for decorative purposes, this shoe is made from 680g of pure gold. This pair of gold shoes has been put on sale at a jewellery shop in Wuhu in east China’s Anhui province (June 9, 2007). The shoes were designed by Italian designer under the invitation from World Gold Council. China is one of the world’s biggest gold producers. It plans to boost its gold production to 260 tonnes this year (2007). 2) MATERIALS To design a product, there is a wide range of materials available to choose from and a correspondingly wide range of properties. It must be emphasized that although a material may be chosen because it satisfies the predominant requirement such as the strength, there can always be other additional backup properties such as corrosion resistance and machinability that have to be put into consideration. 2) MATERIALS The desired cluster of properties will not necessarily be wide ranging. The exact combination required will depend on the given application of the product concerned. Table 1 categorized the elemental way to carry out the selection. Table 2 characterized the features of the 3 basic groups of materials. Plastics Metals Ceramics Weak Strong Strong Compliant Stiff Brittle Durable Tough Durable Temperature- Electrically Refractory sensitive conducting Electrically Electrically High thermal insulating insulating conductivity Low thermal conductivity 2) MATERIALS However, there are exceptions to the generalizations shown in these 2 tables. ❑ Plastics, for example, are indeed relatively durable but some of them are subject to degrading. ❑ Metals are generally tough. Although the widespread use of metallic materials for engineering purposes is due largely to the fact that they are mostly able to offer a combined set of strength and toughness property, they can be very brittle especially at low service temperatures. 2) MATERIALS ❑ Ceramics may be chosen for its high wear resistance, its brittle nature may rule out the consideration when toughness is needed. 2) MATERIALS At an early stage in the design process, it should become apparent that several different materials are capable of performing a particular function. It is then necessary to decide which one to choose from. This requires to measure the important properties in the unambiguous, rational manner. The task is easy if a property is well understood in terms of fundamental science, but not all material properties are of this sort. For example, if it is essential to measure the weld ability of metals, there is no single parameter can do this because weld ability measures the overall response of a material to a particular process. 2) MATERIALS In general, we can consider property parameters as two type: a) fundamental parameters - these parameters measure the basic properties of materials such as electrical resistively or weight. They generally have the advantage that they can be used directly in design calculations. b) ranking parameters - these generally do not measure single fundamental properties and can only be used to rank materials in order of superiority. They cannot be used directly in design calculations, but could be used in formalized selection procedures. Typical examples are corrosion resistance, machinabilility and formability. 3) FABRICATION PROCESSES In selection a material for a particular design, one cannot ignore the method of fabricating the material. In actual fact, there is a very strong inter- relationship between the fabricating processes and the structure, physical and mechanical properties of materials. The fabrication methods of materials can be grouped into two categories: a) Primary material processes b) Secondary processes 3) FABRICATION PROCESSES a) Primary material processes The casting process is probably the most widely applicable. On the metals, we can cast majority of the alloys in practically any shape, from a tiny 20g modelled toys made from zinc alloys to a 150 tons of ore crusher machine made from cast irons. A small 7 in. bore cupola furnace Tapping a ladle of cast iron in a 7 in. bore Cupola. Pouring a 9 lb. Iron Casting with 7 in. Cupola in background. A Cast Lamp Base (Gray cast iron?) 3) FABRICATION PROCESSES a) Primary material processes (casting process) In the case of polymeric materials, few really large castings are made but a very high tonnage of small parts is produced by injection moulding, which is essentially a die casting process. In the composites, the casting of metallic matrix composites and fibre-liquid polymer-mixtures by injection moulding is also important. 3) FABRICATION PROCESSES The deformation methods such as rolling and forging can be used for all metals and alloys that offer sufficient ductility. Glasses are uniquely suited to a great variety of deformation methods. However, oxide ceramic is brittle and must be formed by powder methods. Thermoplastics have excellent formablity. Powder methods applied to metals to produce items which are difficult to process by other methods. Typical case is the production of bearings. The methods are also employed to produce a great number of thermosets and composites. 3) FABRICATION PROCESSES b)Secondary processes Machining is a common process used for all materials. Different tool materials have been developed to cope with a wide of metallic and non-metallic materials. Although polymers and composites are machinable, several precautions are needed. Threads, for examples, have relatively low strength and wear resistance, and holes drilled through composite rupture and disturb the bonding structure near the hole. Machining is usually a secondary process that follows a forming process but normally precedes joining and finishing processes. 3) FABRICATION PROCESSES Thermal treatment involving heating and quenching operations to produce harder and stronger materials. Annealing is used to remove the effects of cold work and to soften a metal. These operations are restricted to metals. On the other hand, stress- relief treatment can be used for metallic, ceramic, polymeric and composite materials. Welding (joining) can be used readily for metals. The joining of ceramics is accomplished by sintering. Thermoplastics are very easy to weld. But for the thermosets, only those exhibit with small amount of cross-linking can be welded. 4) SERVICE CONDITIONS Service conditions may change the material properties which make the materials incapable of continuing their function. These changes can be caused by the chemical environment, heat or radiation. Chemical environment may cause surface damage to the material either in terms of material loss or surface quality. It can also affect other properties such as wear resistance and fatigue strength. Heat or radiation usually affect the bulk properties of the material. Consequently, in selecting a material for a given application, apart from considering its performance in mechanical or physical functions, the material should also resist harmful attack, by the service environment. 4) SERVICE CONDITIONS Metallic materials, especially the ferrous group, are prone to chemical attack. On the other hand, ceramic materials are more chemically stable. Porcelain, for example, resists almost all acids while glasses are resistant to attack by water, atmosphere and chemicals. Although polymeric materials are generally chemically stable, the absorbed water will change their properties and cause swelling of the products. 4) SERVICE CONDITIONS Metallic materials become substantially weaker as their service temperature increases. The loss of strength is a function of time so that useful strength is lower after longer service. Ceramic materials are very stable at high temperatures. Their use for prolonged service time at such temperatures presents no problem unless the service conditions involve rapid fluctuations in temperature. In this instance, ceramic materials can suffer spalling. 4) SERVICE CONDITIONS Polymeric materials are also prone to thermal damage. Heating activate depolymerization and reduce the performance of polymer. 4) SERVICE CONDITIONS Radiation damage in metallic materials usually increases their hardness and reduces their ductility in a similar way to cold working. Similarly, annealing will soften the material back to its original condition. In polymeric materials, commercial use has been made of the effect of ultra-violet radiation because it causes branching in polyethylene. In fact, radiation increases the stability of polyethylene at the temperature of boiling water. However, eletromagnetic radiation can degrade polymers and adversely affects their properties. 5) ECONOMIC REQUIREMENTS Except in certain military, research and similar applications, economical considerations often predominate the decision in the choice of the material for the product design. The economic requirements should take into account the total cost of the components. This cost will include the cost of raw material, the cost of fabrication and the availability of a material that can affect the completion date of the products. 5) ECONOMIC REQUIREMENTS For the cost of raw metallic material, it is not necessarily inexpensive because its ore is plentiful. The cost of a raw material is often associated with the complexity of the method that must be used to extract from its ore and prepare for the final application. In addition, those materials which are commonly used and are manufactured by large scale processes are normally cheaper and more competitive than those which are produced in order to meet the special requirements. 5) ECONOMIC REQUIREMENTS It is not always true that the use of cheaper raw material should have a significant effect on the final cost. In some case, the choice of a more expensive material may permit the use of relatively simple and low cost processing methods whereas a cheaper material may require lengthy, complex and expensive production methods. For example, the use of more expensive prehardened steels makes it possible for the users to eliminate heat treatment and the resulting component distortion. 5) ECONOMIC REQUIREMENTS The availability of material will influence the production cost. A material which can acquire within a shorter lapse time can influence the overall decision of the choice. When availability of specific wrought dimension is limited, a switch to an alternate material or size may be needed abide the increased cost of processing. When production involves more than one size, it may become necessary to buy base quantities of large stock size and use it for a variety of smaller size parts, even though the extra machining involved in reducing the large stock size is more costly than buying smaller quantities of the correct size. MATERIALS SELECTION Summery: ❑ We have discussed how product design, material and manufacture are inter-depended during the course of materials selection. ❑ Considerations of materials selection: 1) the performance of materials 2) the response of materials towards fabrication 3) the conditions in which the materials are put into service 4) the economic influence FURTHER READINGS 1) M.M.Farag, “Materials & Process Selection in Engineering” Applied Sc.Publisher, 1979. 2) F. A. A. Crane & J.A.Charles, “Selection & Use of Engineering Materials” Butterworths, 1984.