Hydrology Notes 2024 PDF
Document Details
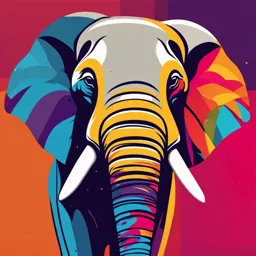
Uploaded by ExaltedPelican
University of Mines and Technology (UMaT), Tarkwa
2024
Eunice E. Attafuah
Tags
Summary
This document provides notes for a hydrology course at the University of Mines and Technology (UMaT). It covers the hydrologic cycle, including precipitation, evaporation, infiltration, and groundwater flow. The course also explores human interaction with the cycle and methods for measuring and estimating hydrologic processes.
Full Transcript
Hydrology University of Mines and Technology (UMaT), Tarkwa School of Railway and Infrastructure Development (SRID) Faculty of Geosciences and Environmental Studies Eunice E. Attafuah (PE-GhIE) 6/1/24 0 | P ES a g...
Hydrology University of Mines and Technology (UMaT), Tarkwa School of Railway and Infrastructure Development (SRID) Faculty of Geosciences and Environmental Studies Eunice E. Attafuah (PE-GhIE) 6/1/24 0 | P ES a g372 e Course Description Water is the most abundant substance on the earth and the principal constituents of all living things. Hydrology, which treats all the phases of the earth’s water is a subject of great importance for people and the environment. In this course we will explore the components of the hydrologic cycle including processes of precipitation, evaporation, transpiration, infiltration, ground-water flow, surface runoff and streamflow. We will study the physical processes and explore basic methods of measuring and estimating rates of hydrologic processes as well as explore our personal interaction with the hydrologic cycle. Course Objectives Upon successful completion of this course, you should be able to: define, explain, and correctly use terms and concepts used to describe basic physical hydrologic processes including evaporation, transpiration, precipitation, infiltration, ground-water flow and surface runoff. Explain the hydrologic cycle and the ways that human’s impact and interact with it. Solve basic hydrologic problems to estimate the magnitude and frequency of hydrologic events. Use basic accepted hydrologic modelling to quantitatively measure and estimate the role of physical processes in the hydrologic. References and Recommended Textbooks Te Chow, V., Maidment, D. R. and Mays, L. W. (2010) Applied Hydrology. Tata McGraw-Hill Education (McGraw-Hill series in water resources and environmental engineering). Available at: https://books.google.com.gh/books?id=RRwidSsBJrEC. Morrison, F. A. (2013) An Introduction to Fluid Mechanics. Cambridge: Cambridge University Press. doi: DOI: 10.1017/CBO9781139047463. Raghunath, H. M. (2006) Hydrology : Principles, Analysis And Design. New Age International (P) Limited. Available at: https://books.google.com.gh/books?id=9mdkJ0T2P30C. Subramanya, K. (2006) Engineering Hydrology. Tata McGraw Hill Publishing Company Limited. Available at: https://books.google.com.gh/books?id=_E7TwAEACAAJ. 1|Page Tentative Schedule Chapter Topic Week Date 1 Introduction 1st week 2 Hydrologic cycle, Water balance, Catchment 2nd & 3rd week 3 Precipitation 4th & 5th week 4 Interception and Depression 6th week Test 1 (0.5 hours) Week 6 5 Evaporation, and Infiltration 7th Week 5 Groundwater 8th & 9th week 6 Runoff and Surface water resources 9th &10th week 7 Hydrograph and Flood routing Week 10 8 Test 2 (0.5 hours) 11th week Revision / Exams Final Exams 2|Page Contents 1.0 INTRODUCTION TO HYDROLOGY................................................................................ 7 1.1 Introduction........................................................................................................................ 7 1.2 World’s Water Resources................................................................................................... 7 1.3 Interrelationship with other Sciences................................................................................. 8 1.4 Applications of hydrology................................................................................................. 8 2 Hydrological Cycle, Water Balance and Catchment Analysis............................................... 10 2.1 Hydrologic Cycle............................................................................................................. 10 2.1.1 Residence Time of Water.......................................................................................... 12 2.2 Hydrologic Continuity Equation and Water Balance....................................................... 13 2.2.1 Hydrologic Continuity Equation.............................................................................. 13 2.2.2 Water Balance........................................................................................................... 13 2.3 Catchment /Watershed..................................................................................................... 14 2.3.1 How to Delineate a Catchment Area........................................................................ 15 3 Precipitation............................................................................................................................ 18 3.1 Introduction to Precipitation............................................................................................ 18 3.2 Formation of Precipitation............................................................................................... 18 3.3 Factors that affect Precipitation....................................................................................... 21 3.4 Artificially Induced Precipitation.................................................................................... 21 3.5 Measurement of Rainfall (Precipitation)......................................................................... 21 3.5.1 Non–Recording Rain Gauges................................................................................... 22 3.5.2 Recoding Rain Gauges............................................................................................. 23 3.5.3 Radar Measurement & Satellite Measurement of Rainfall...................................... 25 3.6 Rain Gauge Network....................................................................................................... 25 3.7 Errors In Rainfall Measurement...................................................................................... 26 3.8 Analysis Of Precipitation................................................................................................. 26 3|Page 3.8.1 Rainfall Data, Extension and Interpretation............................................................. 26 3.9 Rainfall mass curve and rainfall hyetograph................................................................... 28 3.10 Intensity – Duration – Frequency Analysis Of Precipitation....................................... 29 3.11 Checking for Consistency of Data: Double-Mass Analysis............................................ 32 3.12 Point & Areal Measurements....................................................................................... 34 3.12.1 Point Measurement................................................................................................... 34 3.12.2 Areal Measurements................................................................................................. 35 3.13 Variations in Precipitation............................................................................................ 38 From Record of An Adjoining Station................................................................................... 38 Water Loses.................................................................................................................................... 41 4 Interception and Depression................................................................................................... 42 4.1 Interception...................................................................................................................... 42 4.1.1 Factors Affecting Interception.................................................................................. 42 4.1.2 Measurement of Interception.................................................................................... 42 4.1.3 Calculation of Interception Loss.............................................................................. 42 4.2 Depression Storage.......................................................................................................... 43 4.2.1 Calculation................................................................................................................ 43 5 Evaporation and Transpiration............................................................................................... 45 5.1 Evaporation...................................................................................................................... 45 5.2 Factors Affecting Evaporation from Free Water Surface................................................ 46 5.2.1 METEOROLOGICAL FACTORS........................................................................... 46 5.2.2 Geographical Factors................................................................................................ 47 5.2.3 Methods Of Estimating Evaporation from Free-Water Bodies................................ 48 5.2.4 Measures to Reduce Lake Evaporation.................................................................... 50 5.2.5 Analytical Methods.................................................................................................. 51 5.3 Transpiration.................................................................................................................... 53 4|Page 5.3.1 Factors affecting Transpiration................................................................................. 53 5.3.2 Determination of Transpiration................................................................................ 54 5.4 Evapotranspiration........................................................................................................... 54 5.4.1 Factors Affecting Evapotranspiration....................................................................... 55 5.4.2 Estimation of Evapotranspiration............................................................................. 55 6 Infiltration............................................................................................................................... 58 6.1 Factors affecting Infiltration............................................................................................ 60 6.2 Calculation of Infiltration Rate........................................................................................ 61 6.2.1 Horton infiltration equation...................................................................................... 61 6.3 Infiltration Indexes........................................................................................................... 62 6.3.1 The Φ - Index........................................................................................................... 62 6.3.2 The W – Index.......................................................................................................... 62 6.4 Measurement of Infiltration............................................................................................. 63 6.4.1 Infiltrometers............................................................................................................ 63 6.4.2 Sprinkling tests......................................................................................................... 66 7 Ground Water Hydrology....................................................................................................... 67 7.1 Introduction...................................................................................................................... 67 7.2 Basic concepts and definitions......................................................................................... 67 7.2.1 Aquifer, Aquitard, Aquiclude and Aquifuge............................................................. 67 7.3 Types of Aquifer.............................................................................................................. 68 7.3.1 Unconfined Aquifer.................................................................................................. 68 7.3.2 Confined Aquifers.................................................................................................... 69 7.3.3 Leaky Aquifer........................................................................................................... 71 7.4 Factors Of Importance in Groundwater........................................................................... 72 7.4.1 Porosity..................................................................................................................... 73 7.4.2 Permeability coefficient or hydraulic conductivity.................................................. 76 5|Page 7.5 Vertical Distribution of Groundwater.............................................................................. 76 7.5.1 Zone of Aeration....................................................................................................... 78 7.5.2 Zone of Saturation.................................................................................................... 79 7.6 Geologic Formations as Aquifers.................................................................................... 79 7.7 Groundwater Basins......................................................................................................... 81 7.8 Springs............................................................................................................................. 81 8 Runoff and Surface Water Resources..................................................................................... 84 8.1 Introduction...................................................................................................................... 84 8.2 Streamflow....................................................................................................................... 86 8.2.1 Stream Flow Components........................................................................................ 86 8.2.2 Streamflow Hydrographs:........................................................................................ 88 8.2.3 Factors affecting Streamflow.................................................................................... 89 8.3 Measurement of Streamflow............................................................................................ 89 8.3.1 Stage/Water Level Measurement.............................................................................. 90 8.3.2 Principles of measurement equipment..................................................................... 92 8.3.3 Streamflow measurements....................................................................................... 96 8.4 Hydrograph.................................................................................................................... 108 8.4.1 Factors affecting hydrograph shape.........................................................................112 6|Page 1.0 INTRODUCTION TO HYDROLOGY 1.1 Introduction Water is the most abundant substance on the earth and the principal constituents of all living things. It is a major force constantly shaping the surface of the earth. Water is a key factor in air- conditioning the earth for human existence and in influencing the progress of civilization. Hydrology, which treats all the phases of the earth’s water is a subject of great importance for people and the environment. The word Hydrology is made up of “hydro” and “logy” “Hydro” is a Greek word which means water and “logy” means subject of study. Therefore, hydrology literally means water science or the study of water. Hydrology is a multidisciplinary subject that deals with the study of water: its properties, distribution, and behaviour. It is the science that treats the properties, occurrence, circulation, and distribution of the waters of the earth and the interactions with the atmosphere, land surface and underlying soils and rocks. A knowledge of hydrology is fundamental to water and environmental professionals (engineers, scientists, and decision makers) in such tasks as the design and operation of water resources, wastewater treatment, irrigation, flood defense, navigation, pollution control, hydropower, ecosystem modelling, etc. 1.2 World’s Water Resources The world's total water resources are about 332.5 million cubic miles of water, over 96 percent is saline. And, of the total freshwater, over 68 percent is locked up in ice and glaciers. Another 30 percent of freshwater is in the ground. Fresh surface-water sources, such as rivers and lakes, only constitute about 22,300 cubic miles (93,100 cubic kilometers), which is about 1/150th of one percent of total water as shown in Figure 1.1. Yet, rivers and lakes are the sources of most of the water people use every day. 7|Page Figure 1-1: Distribution of Earth's Water Resources 1.3 Interrelationship with other Sciences Hydrology is a branch of scientific and engineering discipline. Fig.1.2 shows the position of hydrologic science in the spectrum of basic sciences to water resource management. Hydrology is an interdisciplinary geoscience built upon the basic sciences of mathematics, statistics, physics, chemistry, and biology. 1.4 Applications of hydrology Hydrology finds its greatest application in the design and operation of water resources engineering projects, such as: find out maximum probable flood at proposed sites e.g., Dams. The variation of water production from catchments find the relationship between a catchments' surface water and groundwater resources. know the required reservoir capacity to ensure adequate water for irrigation or municipal water supply in droughts condition. prediction of flood over a spillway, at highway culvert or in urban storm drainage. 8|Page assess the reservoir capacity required to assure adequate water for irrigation or municipal water supply during drought. water supply design, which is based on catchments area, amount of rainfall, dry period, storage capacity, runoff evaporation and transpiration. In all engineering projects, hydrological investigations for the proper assessment of the following factors are necessary: The capacity of storage structures such as reservoirs The magnitude of flood flows to enable safe disposal of the excess flow. The minimum flow and quantity of flow available at various seasons. The interaction of the flood wave and hydraulic structures, such as levees, reservoirs, barrages and bridges. Figure 1-2:Interrelationship of hydrologic science with other sciences 9|Page 2 Hydrological Cycle, Water Balance and Catchment Analysis 2.1 Hydrologic Cycle The hydrologic cycle, also known as the water cycle, is the central focus of hydrology. The cycle has no beginning or end, and its many processes occur continuously. The driving force of the circulation is derived from the radiant energy received from the Sun. As shown in Figure 2.1, water evaporates from the oceans and the land surface (the transfer of water from the liquid to the gaseous state) to become part of the atmosphere; water vapour is transported and lifted in the atmosphere (the water vapour changes back to the liquid state) until it condenses and precipitates (snow or rain) on land or the ocean; snow may accumulate in Polar regions or on high mountains and consolidate into ice, in which state water may be stored naturally for very long period or in temperate regions precipitated water may be intercepted by vegetation, become overland flow over the ground surface, infiltrate into the ground, flow through the soil as subsurface flow, and discharge into streams as surface runoff. Water intercepted by vegetation may be transpired back into the atmosphere. Much of the intercepted water and surface runoff returns to the atmosphere though evaporation. The infiltrated water may percolate deeper to recharge groundwater, later emerging in springs or seeping into streams to form stream flow, and finally flowing out to the sea or evaporating into the atmosphere as the hydrological cycle continues. The sequence of events above is a simplistic picture of a very complex cycle that has been taking place since the formation of earth. It is seen that the cycle is very vast and complicated with large number of paths of varying time scales. The hydrological cycle is a continuous recirculating cycle in the sense that there is neither a beginning nor an end or a pause. Each path of the cycle involves one or more of the following aspects: a) Transportation of water b) Temporary storage c) Change of state For example, the process of rainfall has the change of state and transportation, and groundwater path has storage and transportation aspects. However, the main components of the hydrologic cycle can be broadly classified as transportation (flow) components and storage components as shown in Table 2.1. The quantities of water going through various individual paths of the hydrological cycle in each system can be described by the continuity. 10 | P a g e Figure 2-1: Hydrologic Cycle Table 2-1: Components of the Hydrological Cycle Transportation Components Storage Components Precipitation Storage on the land surface (Depression storage, ponds, lakes, reservoirs, etc) Evaporation Soil moisture storage Transpiration Groundwater storage Infiltration Runoff The transportation components can further be grouped into phases: liquid-transport phase and vapour-transport phase. Liquid-transport phases of the hydrologic cycle are: Precipitation from the atmosphere onto land surface Throughfall from vegetation onto land surface Surface runoff from land surface to streams, lakes, and rivers, and from streams, lakes, and rivers to oceans Infiltration from land surface to soil Interflow from soil to streams, lakes, and rivers and vice versa Percolation from 11 | P a g e soil to aquifers Capillary rise from aquifers to soil Groundwater flow from streams, lakes, and rivers to aquifers and vice versa and from aquifers to oceans and vice versa. The Vapour – transport phases of the hydrologic cycle are Evaporation from land surface, stream, lakes, river, and oceans to the atmosphere Evapotranspiration from vegetation to the atmosphere Sublimation from snowpack and icecaps to the atmosphere Vapour diffusion from soil to land surface. It should be realized that the hydrological cycle is much influenced by human activities, such as groundwater extraction, river diversion, construction of dams, implementation of irrigation schemes and drainage works, deforestation, etc. Nowadays it is difficult to find river basins with a hydrological system that is not affected by man. 2.1.1 Residence Time of Water Residence time is also known as turnover time, and it is the amount of time it takes for water to cycle through the system i.e., reservoir at equilibrium. Residence time is defined as the amount of water in a reservoir divided by either the rate of addition of water to the reservoir or the rate of loss from it. The resident times for different water resources are shown in Figure 2-2. Figure 2-2: Resident time for some water resources 12 | P a g e 2.2 Hydrologic Continuity Equation and Water Balance 2.2.1 Hydrologic Continuity Equation The principle of conservation of mass is one of the most useful physical principles in hydrologic analysis. The continuity equation states that during a given period, the change in storage must be equal to the total inflow into a given area minus the total outflow from the area. Figure2-3 shows the hydrological Equation. The equation for the various phases per unit time is given as seen in Eqn 2.1 Figure 2-3: Hydrologic Equation 𝑑𝑆 𝐶ℎ𝑎𝑛𝑔𝑒 𝑖𝑛 𝑆𝑡𝑜𝑟𝑎𝑔𝑒 ( ) = 𝑀𝑎𝑠𝑠 𝑖𝑛𝑓𝑙𝑜𝑤( 𝐼) − 𝑀𝑎𝑠𝑠 𝑂𝑢𝑡𝑓𝑙𝑜𝑤(𝑂) 2.1 𝑑𝑡 If the density remains constant at a given time dt, then the equation can be written as ∆𝑆 = 𝑉𝑖 − 𝑉𝑜 2.2 2.2.2 Water Balance The total number of water available on the earth is finite and conserved. Although the total volume on earth remains constant, the distribution of this water is continually changing on continents, in regions and local catchments. The continuity equation is applied in the water balance equation. Conceptually, it is the accounting of inputs and output of flows in a catchment. The equation holds for a specific period of time and may be applied to any given system provided that the boundaries are well defined. The global estimated world water quantities and annual water balance is shown in 13 | P a g e Table 2-2 and Table 2-3 respectively. Another name for this equation is Storage Equation or Water Budget Equation. The water balance equation can be applied to many different systems, such as: ❖ the (terrestrial and oceanic part of the) earth. ❖ an entire river basin. ❖ the root zone, interception reservoir, lake, etc. ❖ a local area like a city or a forest. The Water Budget Equation is given as 𝑉 = 𝑃 + 𝑄𝑖𝑛 + 𝐺𝑊𝑖𝑛 − 𝑄𝑜𝑢𝑡 − 𝐺𝑊𝑜𝑢𝑡 − 𝐸𝑇 2.3 Where 𝑉 is a control Volume (i. e watershed or lake) 𝑃 is Precipitation 𝑄𝑖𝑛 𝑖𝑠 Runoff or discharge in 𝐺𝑊𝑖𝑛 𝑖s Groundwater in 𝑄𝑜𝑢𝑡 𝑖𝑠 Runoff or discharge out 𝐺𝑊𝑜𝑢𝑡 𝑖𝑠 Groundwater out 𝐸𝑇 𝑖𝑠 𝐸𝑣𝑎𝑝𝑜𝑡𝑟𝑎𝑛𝑠𝑝𝑖𝑟𝑎𝑡𝑖𝑜𝑛 2.3 Catchment /Watershed A watershed/drainage basin/catchment (see figure 2-4) is a geographic area from which water drains toward a common watercourse (such as a lake, stream, and ocean) in a natural basin. In hydrology, catchment is a logical unit of focus for studying the movement of water within the hydrological cycle, because the majority of water that discharges from the catchment outlet originated as precipitation falling on the catchment. When considering a watershed, it’s not only the water which falls on the catchment that is to be considered but also land use, topography, geology etc. All of these components affect watersheds. Within each major watershed lie sub-watersheds or tributaries that drain into these larger watersheds. A boundary of a catchment is defined by the drainage divide. It must be noted that drainage basins do not follow administrative boundaries. In summary, the area of land where all of the water that falls on it, drains off to a common outlet is a watershed. NB. Research about topographic and phreatic water divide 14 | P a g e Figure 2-4: A watershed 2.3.1 How to Delineate a Catchment Area Drainage areas are determined by tracing all of the water bodies flowing into the stream or river upstream of the proposed site (Fig. 2-5A). A divide or ridge surrounds every drainage basin (Fig 2-5B). A divide is defined as “the line of separation or dividing ridge marking the boundary between two drainage systems.” To delineate a catchment area, you will need a Topographic map (paper or electronic), Pencil if using paper map and AutoCAD or ArcGIS. The following should be noted: ❖ Utilize a topographic map ❖ A stream or body of water should not be crossed ❖ A basin divide will not fall in a drainage swale ❖ All ridge lines should be followed ❖ When in doubt, consult someone with more experience ❖ After the area has been delineated, it should be reviewed for accuracy 15 | P a g e ❖ Watershed delineation lines should always intersect contour lines perpendicularly A catchment area can be delineated as shown in Fig. 2.5B using the following 7 steps: Step 1: Determine the discharge point and which part of the streams and tributaries that are upstream from that point. Step 2: Next, working upstream, trace all of the streams that drain into the water body on which your site rests. Remember that the tributaries point upstream. Figure 2-5: Delineating a catchment area: A. divisions of a river; B. a delineated catchment Note: Contour lines spaced far apart denote that the landscape is more level and gently sloping. This is an indication of flat land. Contour lines spaced very close together denote dramatic changes in elevation over a short distance. Close contour lines mean steep slopes. Step 3: After tracing the streams, you can delineate your drainage area by tracing the divide that encircles all of the tributaries draining into the main stream. Begin by recognizing valleys and ridges. Step 4: Starting at a designated downstream point, draw a line perpendicular to the nearest uphill contour line. Step 5: Repeat Step 5 continuing from contour to contour. Make sure to intersect the contours at break points. Break points are the highest elevations where half of the precipitation in that area would flow to one stream and half the precipitation would flow to another. 16 | P a g e Step 6: Connect all break points, making sure to hit all high points in the area. Also, make sure the line connects to the original discharge point. The completed line represents the drainage area boundary. The watershed is now delineated. Step 7: Picture a drop of rain falling on the surface of the map, inside of the drainage area boundary. Envision the water flowing down the slopes as it perpendicularly crosses contour lines. Trace its path to the nearest stream that flows to the discharge point you are studying. Repeat this process for different points on the watershed boundary to verify that the boundaries are correct. The applications are many; engineers use the delineated watershed boundary in conjunction with precipitation, infiltration, and evaporation rates to determine water balances in drainage areas as well as model hydrologic processes in the basin. The model can be used to predict changes in the basin as well as the effect of climate change on the water resources in the basin. These calculations can also be used for hydraulic modelling where stormwater management facilities like dams, bridges, culverts, drains, and ponds are sized. 17 | P a g e 3 Precipitation 3.1 Introduction to Precipitation Precipitation denotes all forms of water that reach the earth from the atmosphere. The usual forms are rainfall, snowfall, hail, frost and dew. The moisture in the atmosphere, although forming one of the smallest storages of the Earth’s water, is the most vital source of fresh water for mankind. Water is present in the air in its gaseous, liquid, and solid states as water vapour, cloud droplets and ice crystals, respectively. Precipitation occurs in liquid or solid forms. Liquid precipitation include rain, drizzle, dew. These have a rapid response to the hydrological cycle. The solid precipitation include snow, hail, sleet. Solid precipitation reacts slowly to the hydrologic cycle. In Ghana, the most common forms of precipitation are rainfall, drizzle and dew. 3.2 Formation of Precipitation For precipitation to occur, some conditions need to be satisfied. They are: a. A supply of moisture b. A means of lifting this air higher c. Condensation d. Condensation nuclei e. Growth of the small droplets by collision and coalescence. The supply of moisture is obtained through evaporation from wet surfaces, transpiration from vegetation or transport from elsewhere. The cooling of moist air may be through contact with a cold earth surface causing dew, white frost, mist or fog, and loss of heat through long wave radiation (fog patches). However, much more important is the lifting of air masses under adiabatic conditions (dynamic cooling) causing a fall of temperature to near its dew point. The precipitation may be due to 1. Thermal convection (convectional precipitation)—This type of precipitation is in the form of local whirling thunder storms and is typical of the tropics. The air close to the warm earth gets heated and rises due to its low density, cools adiabatically to form a cauliflower shaped 18 | P a g e cloud, which finally bursts into a thunder storm. When accompanied by destructive winds, they are called ‘tornados’. Rainfall intensities of convective storms can be very high locally; the duration, however, is generally short. 2. Conflict between two air masses (frontal precipitation)—When two air masses due to contrasting temperatures and densities clash with each other, condensation and precipitation occur at the surface of contact, Fig. 3-1. This surface of contact is called a ‘front’ or ‘frontal surface’. If a cold air mass drives out a warm air mass’ it is called a ‘cold front’ and if a warm air mass replaces the retreating cold air mass, it is called a ‘warm front’. On the other hand, if the two air masses are drawn simultaneously towards a low-pressure area, the front developed is stationary and is called a ‘stationary front’. Cold front causes intense precipitation on comparatively small areas, while the precipitation due to warm front is less intense but is spread over a comparatively larger area. Cold fronts move faster than warm fronts and usually overtake them, the frontal surfaces of cold and warm air sliding against each other. This phenomenon is called ‘occlusion’ and the resulting frontal surface is called an ‘occluded front’. 3. Orographic lifting (orographic precipitation)—The mechanical lifting of moist air over mountain barriers, causes heavy precipitation on the windward side (Fig. 3-2). For example Cherrapunji in the Himalayan range and Agumbe in the western Ghats of south India get very heavy orographic precipitation of 1250 cm and 900 cm (average annual rainfall), respectively. 4. Cyclonic (cyclonic precipitation)—This type of precipitation is due to lifting of moist air converging into a low-pressure belt, i.e., due to pressure differences created by the unequal heating of the earth’s surface. Here the winds blow spirally inward counterclockwise in the northern hemisphere and clockwise in the southern hemisphere. There are two main types of cyclones—tropical cyclone (also called hurricane or typhoon) of comparatively small diameter of 300-1500 km causing high wind velocity and heavy precipitation, and the extra- tropical cyclone of large diameter up to 3000 km causing wide spread frontal type precipitation. Condensation of water vapour into small droplets does not occur immediately when the air becomes saturated. It requires small airborne particles called aerosols, which act as nuclei for water vapour to condense. Nuclei are salt crystals from the oceans, combustion products, dust, ash, etc. In the absence of sufficient nuclei the air becomes supersaturated. When the temperature drops below 19 | P a g e zero, freezing nuclei with a structure similar to ice are needed for condensation of water vapour into ice crystals. These nuclei are often not available and the droplets become super-cooled to a temperature of -40oC. Cloud droplets with a diameter of 0.01 mm need an updraught of only 1 cm.s- 1 to prevent the droplet from falling. A growth of particles is necessary to produce precipitation. This growth may be achieved through coalescence, resulting from collisions of cloud droplets. Small droplets moving upwards through the clouds collide and unite with larger droplets which have a different velocity or move downwards. Another method of growth known as the Bergeron process is common in mixed clouds, which consist of super-cooled water droplets and ice crystals. Water vapour condenses on the ice crystals, the deficit being replenished by the evaporation of numerous droplets. In the temperature range from -12oC to -30oC the ice crystals grow rapidly and fall through the clouds. Aggregates of many ice crystals may form snowflakes. Depending on the temperature near the surface they may reach the ground as rain, snow, hail or glazed frost. Figure 3-1: Frontal Precipitation Figure 3-2: Orographic Precipitation 20 | P a g e 3.3 Factors that affect Precipitation The precipitation at a place and its form depends upon a number of meteorological and other factors, such as ❖ Latitude – precipitation decreases with increasing latitude because temperature reduces atmospheric moisture. ❖ Elevation – due to orographic cooling. ❖ Distance from moisture source. Precipitation is usually lower at greater distances from the ocean. ❖ Prevailing wind direction – if it is into the sea. ❖ Relation to mountain ranges – windward sides typically cloudy & rainy with leeward side typically dry and sunny. ❖ Relative temperatures of land and bordering oceans. 3.4 Artificially Induced Precipitation Condensation nuclei can be artificially supplied into the atmosphere to induce precipitation under certain conditions (Dry ice & silver iodide have been used as artificial nuclei). Condensation nuclei are spread from aircrafts in which silver iodide solution is evaporated with a propane flame to produce particles. Research is continuing in this phase of weather control and many legal and technical problems remain to be resolved regarding artificial inducement of precipitation. 3.5 Measurement of Rainfall (Precipitation) Rainfall is measured on the basis of the vertical depth of water accumulated on a level surface during an interval of time, if all the water remained where it fell. It is measured in millimeters. The most common instrument used for the measurement is the rain gauge see Figure 3-3. There are two major types of rain gauges namely non-recording and recording rain gauges. Radar and Satellites are also employed the measurement of rainfall. The requirements for gauge construction are: ❖ The rim of the collector should have a sharp edge. ❖ The area of the aperture should be known with an accuracy of 0.5 %. ❖ Design is such that rain is prevented from splashing in or out. ❖ The reservoir should be constructed so as to avoid evaporation. 21 | P a g e ❖ In some climates the collector should be deep enough to store one day's snowfall. Wind turbulence affects the catch of rainfall. Wind is one of the most important factors in rain- gauge accuracy. Updrafts resulting from air moving up and round the instrument reduce the rainfall catch. To reduce the effects of wind, rain gauges can be provided with windshields. Moreover, obstacles should be kept far from the rain gauge (distance at least twice the height of such an object) and the height of the gauge should be minimised (e.g. ground-level rain gauge with screen to prevent splashing). Figure 3-3: Rain gauge 3.5.1 Non–Recording Rain Gauges The standard gauge consists of a funnel 20.32cm in diameter discharging into a tube 6.43cm in diameter. A stick graduated in millimeters is used to measure the precipitation or a measuring cylinder is used to measure the volume of the water. Precipitation in excess of 5cm overtops the inner tube and collects in the overflow can. Large storage gauges are used in remote areas to store precipitation for periods of 30 days or more. Advantages 1. Initial cost is low 2. Usually, recording cannot be interrupted due to mechanical / electrical defect. Disadvantages 1. The non-recording gauge gives only the total depth of rainfall (for the previous 24 hours.) and does not give the intensity and duration of rainfall during different time intervals of the day. 22 | P a g e Figure 3-4: Non-recording Rain Gauge 3.5.2 Recoding Rain Gauges Recording gauges are of three different types: the weighing type, the tilting bucket type and the float type. The weighing type observes precipitation directly when it falls (including snow) by recording the weight of the reservoir. With the float type the rain is collected in a float chamber. The vertical movement of the float is recorded by pen on a chart. This type of recorder has now become obsolete. The weighing and float type recorders have to be emptied manually or by automatic means. The tilting or tipping bucket type (figure 3-3) is a rather simple recording rain gauge, but less accurate (only registering when bucket is full, losses during tipping and losses due to evaporation). The standard rain gauge (or storage gauge) for daily rainfall measurement is observed at a fixed time each morning. Recording gauges used to be equipped with charts that have to be replaced every day, week or month, depending on the clockwork. The rainfall is usually recorded cumulatively (mass curve) from which the hyetograph (a plot of the rainfall with time) is easily derived (see figure 3.4). However, in recent times, the recording rain gauges are equipped with an electronic device to continuously register the change in weight, the position of the float or the time of tipping. Tipping bucket: It consists of a pair of buckets pivoted under a funnel in such a way that when one bucket receives 0.25mm of precipitation, it tips, discharging its contents into a reservoir and bringing the other bucket under the funnel. The tipping of the bucket activates an electric circuit, which causes a pen to move on a chart wrapped around a drum, which revolves, by a clock mechanism. It consists of a cylindrical receiver 30cm diameter with a funnel inside. Weighing bucket: It is the most commonly used type of self-recording gauge (for recording rainfall). It consists of a receiving bucket supported by a spring or lever balance. The weight of rain 23 | P a g e collected in the bucket depresses the electric mechanism. The movement of the bucket (depression) is transmitted to a pen that traces a record of the increasing weight of the bucket and its contents on a clock-driven chart wrapped round a clock-driven drum. The rainfall is usually recorded cumulatively (mass curve) from which the hyetograph (a plot of the rainfall with time) is easily derived as shown in Figure 3-5. Figure 3-5: Hyetograph derived from a mass curve Advantages 1. Automatic recording obviates the necessity of an attendant. 2. It indicates intensity of rainfall at any time as well as the total rainfall while the non- recording gauge gives only the total rainfall. 3. It is the only means to get rainfall data from inaccessible places in hilly areas. 4. Element of human error is eliminated. 5. Capacity of the gauge is big. 6. It measures the time period also. 7. It can operate over extended periods without attention. Disadvantages 1. High initial cost 2. Recording may be interrupted due to mechanical or electrical defects. 3. For the tipping bucket intermittent tipping may cause loss of water. 24 | P a g e 3.5.3 Radar Measurement & Satellite Measurement of Rainfall Radars These are specially adapted to large and inaccessible catchment areas. One radar is capable of measuring rainfall to a range of 100km with the accuracy as is obtainable with 100 rain gauges. The radar provides instantaneous precipitation maps extending to over thousands of square kilometers. The data reflects the spatial variability accurately. Data superior to conventional methods of rain gauges. Radar measurements are more accurate when precipitation amounts are accumulated over a period of time and also average over an area rather than a point. Satellite The satellite sensors do not measure rain directly nor do they respond to liquid drops and ice crystals, but they record visible and infrared characteristics of clouds, which in turn can be correlated to gauge measured precipitation. The principal advantage of rainfall estimation from satellite observation is to give large spatial domain sensed from space satellites. Sensors carried on aircraft or satellite can map many ground surface characteristics that may be helpful to hydrologists. By studying the type and extent of cloud cover, estimates of annual precipitation in remote areas may be possible. Precise quantitative evaluation of precipitation or other hydrologic factors is not possible. Remotely sensed data are generally not a substitute for conventional data collection, but they can be an increasingly useful supplement to conventional data. 3.6 Rain Gauge Network The number of rain gauges necessary to ensure an accurate assessment of a catchment's rainfall depends on the purpose for which the data is intended to be used. An adequate number is required to represent a reliable picture of the intensity and duration of rainfall in the area. The aim of optimum network of rain gauges is that by interpolating between values of different stations, it is possible to determine with sufficient accuracy all quantitative data, averages and extremes that define the statistical distribution of the hydro-meteorological elements at any point in the region. The recommended optimum number of rain gauges required to gauge average rainfall within reasonable limit of error which could be used as a general guide for networking are given in Table 3-1 below: 25 | P a g e Table 3-1:Minimum Number of Rain Gauges for Average Rainfall Estimates a) By Bleasdale b) R.K. Sharma Area No of Rain Area No of Rain gauges gauges miles2 km2 km2 10 26 2 Up to 75 1 100 260 6 76-150 2 500 1300 12 151-300 3 1000 2600 15 301-550 4 2000 5200 20 551-800 5 3000 7800 24 801-1200 6 3.7 Errors In Rainfall Measurement The measured precipitation data are subject to errors due to: 1. Evaporation during precipitation and manual reading 2. Amount used up (about 0.25mm) in wetting the funnel and inside the surfaces of the gauge when it is dry 3. Loss due to rain drop splash from the collecting funnel 4. Height of the gauge above the ground 5. Errors in reading the scale of the gauge 6. Effects of the wind Studies indicate that wind is the major cause of error in precipitation measurement and so if site is well chosen then errors could be brought to the minimum especially in areas where the wind speed are low i.e. below 5-10km per hr. 3.8 Analysis Of Precipitation 3.8.1 Rainfall Data, Extension and Interpretation The annual rainfall suffers wide departure from the normal from year to year. Even in the rainy season, the rainfall in most part of a region is subject to uncertainty of occurrence marked by prolonged dry spells and aberration in time of commencement and withdrawal, and also the total amount received. Thus there is extreme spatial and temporal variation. For planning of water 26 | P a g e resources projects, the variability must be well understood to make the necessary engineering judgement. This calls for a long period of rainfall data or in their absence, methods of obtaining these data with certain reliability. For most stations, the total amount (either monthly or yearly) of rain falling at a point is the usual basic rainfall data available. However, for many purposes this is not adequate and information may be required under any or all of the following headings. 1. Intensity: is the rate at which rainfall occurs. It is expressed in depth per unit time, usually mm per hour. It is the ratio of total amount of rainfall to the duration of the rainfall. Rainfall intensity is classified into: i. Light intensity < 2.5mm per hour ii. Moderate intensity 2.5 – 7.5mm per hour iii. Heavy intensity > 7.5 mm per hour 2. Duration: This is the period of time during which rainfalls in seconds, minutes or hours. 3. Frequency: this refers to the expectation that a given depth of rainfall will fall in a given time , usually expressed by the 'return period' e.g. once in 10 years, once in 25 years, etc. 4. Areal extent: this concerns the area over which a point’s rainfall can be held to apply in hectares, square meters or square kilometres. 5. Intensity-Duration Relationship: the intensity of rainfall is inversely proportional to the duration. It is generally observed that intense rains are of shorter duration. A general formula expressing the relationship are 𝑎 𝑖= for duration less than two hours 3.1 𝑡+𝑏 𝑐 𝑖= 𝑛 for duration greater than two hours 3.2 𝑡 Where i - intensity (mm/hr) t – time(hr) a,b,c,n are locality constants. 6. Depth of precipitation expressed as the thickness of a water layer on the surface in mm or inches. 27 | P a g e 3.9 Rainfall mass curve and rainfall hyetograph The rainfall intensity during a storm can be determined only from recording rain gauge. As was defined, the rate at which rainfall is accumulating at any given instant of time is called the rainfall intensity. A graph showing the variation of rainfall intensity with time is called rainfall hyetograph. A graph showing the cumulative depth of rainfall against time is known as the rainfall mass curve. From the working principles of the float type and the weighing type recording rain gauges, they provide the cumulative values and therefore the necessary data for the rainfall mass curve. A typical rainfall mass curve is shown below. The difference in ordinates of the mass curve at any two points, A, and B gives the amount of rainfall occurring in the time interval. The quantity ∆P/∆t represent the average rainfall intensity in the time period of ∆t. In the limit when the two points become nearer and nearer or as ∆t→0, the line becomes a tangent to the mass curve and hence the slope of a tangent drawn to the rainfall mass curve at any point (eg point C) gives the instantaneous rainfall intensity at that time. 𝐿𝑖𝑚 𝛥𝑃 𝑑𝑃 𝑖= = 3.3 𝛥𝑡 → 0 𝛥𝑡 𝑑𝑡 B Rainfall /mm ∆P A ∆t C Time (in hr) Determination of rainfall intensity from rainfall mass curve If the instantaneous intensities are calculated by drawing tangents to the mass curve at different and numerous time points and measuring the corresponding slopes, the hyetograph of the storm is obtained by plotting these intensities against the corresponding times. 28 | P a g e For the construction of a rainfall hyetograph, it is not always necessary to determine the instantaneous intensities by drawing tangents to the mass curve. Usually a constant time interval (eg 3hrs) is selected and the average intensities in successive time periods equal to the selected time interval are computed and the hyetograph is constructed with these average intensities. A hyetograph with a uniform time interval of 3 hrs is shown below. As can be seen, both the mass curve and the hyetograph depict the time pattern of the storm. The interval used for the construction of a hyetograph depends on the sensitivity required. When the greatest possible sensitivity is required, the smallest interval (5 mins) over which the rainfall rate is constant is selected. The area under the hyetograph storm represents the total rainfall received during the period of that storm. R Rainfall intensity a hyetograph of a (mm/hr) 3 6 9 12 15 18 21 24 27 Rainfall hyetograph 3.10 Intensity – Duration – Frequency Analysis Of Precipitation Precipitation data serve the purpose of estimating the streamflow in many instances, therefore apart from the total quantity of precipitation, the rate known as the intensity and the duration are important in the consideration of peak flow study. A point observation can be considered to be representative of a 26km2 drainage area. Hence the studies of point – rainfall extremes are extensively used in the design of small area drainage systems comprising storm drains, culverts, etc. The intensity – duration – frequency analysis can be carried out only where the data form a recording rain gauge are available. The procedure of analysis is: 1. A specified duration of rainfall e.g. 5min is selected 29 | P a g e 2. From the records of rain gauge, which indicates the accumulated amount of precipitation with respect to time, the maximum rainfall of this duration in each year is noted. This is the maximum incremental precipitation (i.e. difference between accumulated precipitation values) for the selected (5mins) duration obtained from the gauge record. For a partial duration series, all values in the record that exceed a given level are noted. 3. The precipitation values are arranged in descending order and ranked. The return period (recurrence interval) which is the value which will be equalled or exceeded once in T years on an average is computed by the Weibull formula 𝑛+1 𝑇= 3.4 𝑚 where T – return period, m – rank of the data (event), n – total number of events (data). For partial duration series, adjustment in frequency values to change them to annual series is made by applying the following empirical multiplication factors. The conversion factors are presented in Table 3-2.. Table 3-2: Conversion Factor for Partial Duration to Annual Series Return Periods (Years) Conversion factor 2 0.88 5 0.96 10 0.99 >10 1.0 4. Similar analysis are carried out for other selected durations (10,15,20, 30, 60, … mins) 5. For each frequency level computed, the precipitation depths may be plotted for different durations to obtain the depth – duration – frequency curves. 6. The precipitation depths are converted to intensities by 30 | P a g e 60 × 𝑃 𝑖= 3.5 𝑡 where t is the duration (eg. 5mins) and P is the precipitation corresponding to 5 mins duration of specific frequency. These values are plotted as intensity on the ordinate against duration on the abscissa for a particular frequency given. Example 1: The rainfall record contains 32 years of rainfall measurements at 5-minutes intervals. The maximum rainfall amounts for intervals of 5 min, 10min, 15 min, 20 min 25 min and 30 min have been calculated and ranked. The top three rainfall amounts, in mm for each time increment are given in the table below. Calculate the IDF curve for a return period of 20 years. Rank ∆t in minutes 5 10 15 20 25 30 1 12,1 18.5 24.2 28.3 29.5 31.5 2 11.0 17.9 22.1 26.0 28.4 30.2 3 10.7 17.5 21.9 25.2 27.6 29.9 Solution For each time interval, there are n = 32 ranked rainfall amounts of annual maxima. The relationship between the return period and the rank number is given by T = (n + 1)/m = (32 + 1 )/m The return periods can be used instead of the ranking and the given data can be put in the form Return Period T ∆t in minutes (years) 5 10 15 20 25 30 33 12,1 18.5 24.2 28.3 29.5 31.5 16.5 11.0 17.9 22.1 26.0 28.4 30.2 11.0 10.7 17.5 21.9 25.2 27.6 29.9 31 | P a g e The rainfall increments with return period, T = 20 years is obtained by linear interpolation between the rainfall increments corresponding to T = 33 years and T = 16.5 years to yield: Duration (min) 5 10 15 20 25 30 Rainfall (mm) 11.2 18.0 22.5 26.5 28.6 30.5 The average intensities for each duration is obtained by dividing by the duration as i = P/(t/60) to obtain IDF curve for a 20 year return period Duration (min) 5 10 15 20 25 30 Intensity (mm/hr) 11.2 18.0 22.5 26.5 28.6 30.5 3.11 Checking for Consistency of Data: Double-Mass Analysis Double-mass analysis is a consistency check used to detect whether the data at a site have been subjected to significant change in magnitude due to external factors such as: ▪ Change in location of rain gauge (i.e. shifting to new site) ▪ Changes in instrument or observational procedure ▪ Change in exposure such as erection of new buildings, growth of trees, which may change the wind pattern around the gauge The double – mass curve analysis is a consistency check used to detect whether the data at a site have been subjected to significant change in magnitude due external factors. A change due to meteorological factors will equally affect all stations involved in the test and thus will not cause a lack of consistency created by the external factors. Double-mass analysis provides a way to adjust inconsistent data.. In the analysis, a plot is made of accumulated annual or seasonal precipitation values at the site in question against the concurrent accumulation values of surrounding stations. Let xo be the rainfall values at the test site and Y the values at surrounding stations. The double mass curve procedure is done in tabular form as below. If a plot of accumulated values of test site (column 4) is plotted against accumulated values of surrounding stations (column 5), a double mass-plot will be obtained. If the data are consistent, the 32 | P a g e plot will be a straight line. On the other hand, an inconsistent data will exhibit a change in slope or break at a point where the inconsistency has occurred. 1 2 3 4 5 Year X Y X i Y i 1951 X1 Y1 X1 Y1 1952 X2 Y X1 + X2 Y1 + Y 2 1953 X3 Y X1 + X2 + X3 Y1 + Y2 + Y 3 ` ` ` ` ` ` ` ` ` ` ` ` ` ` ` 1985 Xn Yn X1 + X2 +……...+ Xn Y1 +Y2 +………+ Yn The inconsistency point is A in the Figure The point A can be located on the table to determine the time when the inconsistency began. If the slope of the line OA is a and that of the line AB is b, the adjustment of the inconsistent data is made by the ratio of the slopes of the two line segments. Two ways of adjustment are possible 1) The data are adjusted to reflect the conditions that existed prior to the indicated break. This is done by multiplying each recent precipitation value after the break point A of test site by the ratio a/b. 2) The data are adjusted to reflect recent conditions following the break. This is achieved by multiplying each value of the values of the test site before the breakpoint by the ratio of b/a. 33 | P a g e An adjustment of the second type is usually made. The double – mass curve technique should seldom be used in mountainous areas. It is also not suitable for adjustment of daily or storm precipitation. Figure 3-6: Double Mass Curve 3.12 Point & Areal Measurements Precipitation events are recorded by gauges at specific locations. It is important to have accurate rainfall information in a catchment for hydrological assessment. Rainfall varies in space and it is expensive to install and maintain a very dense rain gauge network to completely cover all the catchments. As a result, only a limited number of gauges are installed and there are large gaps between the gauges. Point precipitation data from a network of gaged stations within the area of analysis are used collectively to estimate areal variability of rain and snow. Rainfall data are usually represented as mm/hour, mm/day, etc. The density and arrangement of network as well as the method adopted for the areal analysis influence the estimate of the areal value of the rainfall from the data points. 3.12.1 Point Measurement Data from a single nearby rain gauge are often sufficiently representative to allow their use in the design of small projects. In the simplest and most common case there is only one rain gauge in or near the catchment of interest. Hence by necessity the spatial variability of precipitation within the 34 | P a g e catchment is ignored and the single point measure is assumed to represent the mean value, such that the rate is multiplied by the area to estimate the total precipitation over the catchment. Given the great spatial variability in actual precipitation, this approach is likely to yield large errors. 3.12.2 Areal Measurements In the areal measurements, several precipitation gauges are employed over very large catchments. Weighted average of these gauge measurements are used to estimate the precipitation. The average depth of precipitation over a specific area is often required to predict watershed hydrologic response or to develop frequency design storms. The three basic methods to derive areally averaged values are: 1. Arithmetic mean method 2. Thiessen polygon method 3. Isohyetal method 3.12.2.1 Average depth method. The arithmetic mean of the rainfall amounts measured in the area provides a satisfactory estimate for a relatively uniform rain. This is a simple method and can be used when the gauges are uniformly distributed and computed as shown in Eqn 3.1 𝑛 1 𝑅 = ∑ 𝑅𝑖 3.6 𝑛 𝑖=1 Where R is the rainfall from the various rain gauge stations (i…n) and n is the total number of stations. 3.12.2.2 Thiessen method Lines are drawn to connect reliable rainfall stations, including those just outside the area. The connecting lines are bisected perpendicularly to form a polygon around each station (see figure 3-5). The Thiessen Polygon method is the most popular method used in practical engineering problems. The polygons can be plotted by hand or with computer software (such as ARCView and Matlab). However, it does not consider the gradual change between the gauges and ignores the orographic influence on rainfall. To determine the mean, the rainfall amount of each station is multiplied by the area of its polygon and the sum of the products is divided by the total area. The relative weight for each gauge is determined from the corresponding area. If the area within the catchment assigned to each gauge is 𝐴𝑖 , and its rainfall is 𝑅𝑖 , the areal average rainfall for the 35 | P a g e catchment is 𝑛 1 𝑅̅ = ∑ 𝐴𝑖 𝑅𝑖 3.7 𝐴 𝑖=1 where A is the total catchment area. Advantages 1. More accurate method than arithmetic method. 2. Suitable for plain areas. Limitations 1. Inflexibility is the principal limitation. Thiessen polygon has to be redrawn whenever there is a change in the gauge network by abandonment or addition of one or more stations. 2. It does not allow for orographic influences. 3. Area is geometrically divided into polygons to contain one gauge in each but actual rainfall distribution, which is influenced by the presence of barriers, atmospheric pressure, wind velocity, etc. are not considered. Figure 3-7: Thiessen polygons Figure 3-8: Isohyetal Method 3.12.2.3 Isohyetal Method Rainfall observations for the considered period are plotted on the map and contours of equal precipitation depth (isohyets) are drawn (Fig. 3-7). The areal rainfall is determined by measuring the area between isohyets, multiplying this by the average precipitation between isohyets, and then by dividing the sum of these products by the total area. The isohyetal method is flexible and 36 | P a g e knowledge of the storm pattern can help the drawing of isohyets, but a fairly dense network of gauges is needed to correctly construct the isohyetal map from a complex storm. They are useful for graphical display of rainfall distribution but less popular in engineering applications. The average rainfall between the succesive isohyets taken as the average of the two isohyetal values are weighted with the area between the isohyets, added up and divided by the total area which gives the average depth of rainfall over the entire basin, i.e. ∑ 𝐴1−2 𝑃1−2 𝑃𝑎𝑣 = ∑ 𝐴1−2 3.9 where ∑ 𝐴1−2= area between the two successive isohyets 𝑃1 and 𝑃2 𝑃1 + 𝑃2 𝑃1−2 = 3.10 2 ∑ 𝐴1−2 =A= total area of the basin Advantages 1. Most accurate method. 2. Actual distribution pattern of rainfall is taken into consideration. 3. Weighted areas are determined more accurately. 4. Suitable for areas of over 5000km2 where differences in topography are likely to be pronounced. Limitations 1. Improper analysis can lead to serious error. 2. Accuracy of result depends on the skill of the analyst. 3.12.2.4 Geostatistics (Kriging Method) The conventional methods cannot estimate the uncertainty with the result. Geostatistical methods can be used to compute best estimates as well as error bands that describe the potential magnitude of the estimation error. The uncertainty information is useful for decision making (e.g., to add extra rain gauges if the uncertainties are large at certain points). Kriging is a typical method in 37 | P a g e this category. D.G. Krige, a mining expert, developed a method for interpolation and averaging of spatially varying information, which takes account of the spatial variability and which - unlike other methods - can also indicate the level of accuracy of the estimates made. The Kriging weights obtained are tailored to the variability of the phenomenon studied. 3.13 Variations in Precipitation From engineering point of viewpoint, time variation in precipitation may be more important than regional variations. The annual precipitation cycle is the most marked. For example, the dry harmattan irrigation becomes a necessity for many crops and emphasizes the importance of storage reservoirs. Variation in precipitation from year to year makes it important to design reservoir that are adequate during years of low rainfall. From Record of An Adjoining Station Rainfall values of two or even one adjoining station, where three adjoining stations are not available, may be used to estimate the missing rainfall record of a station. There are two methods namely the Graphical and the Ratio method. These methods hold well only if the two stations have similar meteorological conditions. a) Ratio Method Determine the rainfall, x for 1986 at Station B 38 | P a g e b) Long Term Average Rainfall for a Relatively New Station The long term average rainfall for a relatively new station having a short record is estimated by comparison with the average observed at a few adjacent stations with long records and noting how the averages compare during the short record common to all the stations. The stations should have similar meteorological conditions. For two stations, one of them (B) is an old station with available data for very long periods and the other station A which is relatively new with data available for only a few years. If we need the long term average for the new station we use the formula below. 𝐴𝑎𝑣 𝑅𝐿 𝐵𝑎𝑣 𝑅𝐿 = 3.11 𝐴𝑎𝑣 𝑅𝑆 𝐵𝑎𝑣 𝑅𝑆 𝐴𝑎𝑣 𝑅𝑆 𝑥 𝐵𝑎𝑣 𝑅𝐿 = 3.12 𝐵𝑎𝑣 𝑅𝑆 39 | P a g e Example 40 | P a g e Water Loses The hydrologic equation states that 𝑅𝑎𝑖𝑛𝑓𝑎𝑙𝑙 − 𝐿𝑜𝑠𝑒𝑠 = 𝑅𝑢𝑛𝑜𝑓𝑓 4.1 Runoff can occur only when precipitation has satisfied the demands of evaporation, interception, infiltration, surface storage, and surface detention and channel detention. Runoff occurs only when the rate of precipitation exceeds the rate at which water may infiltrate into the soil. After infiltration rate is satisfied, water begins to fill the depressions on the soil surface. As the depressions are filled, overland flow begins. The depth of water builds up on the surface until the head is sufficient to result in runoff. The volume of water involved in the head build up is the surface detention. As flow moves into defined channels, there is similar build up of head with a volume of water in channel detention. The various forms of water loss are summerised below: i. Interception loss due to surface vegetation, i.e., held by plant leaves. ii. Evaporation: a. from water surface, i.e., reservoirs, lakes, ponds, river channels, etc. b. from soil surface, appreciably when the ground water table is very near the soil surface. iii. Transpiration—from plant leaves. iv. Evapotranspiration for consumptive use—from irrigated or cropped land. v. Infiltration—into the soil at the ground surface. vi. Watershed leakage—ground water movement from one basin to another or into the sea. The various water losses are discussed in the next chapters. 41 | P a g e 4 Interception and Depression 4.1 Interception The portion of precipitation that is temporarily stored by vegetation and other forms of cover, where it is subject to evaporation, is called INTERCEPTION. The process by which precipitation is caught and held temporarily by leaves and blades of grass and evaporation from their surfaces at a later time. Usually, during rain, about 10 to 20 % of the precipitation that falls is intercepted and returned to the hydrological cycle by evaporation. Water loss by interception is especially pronounced under dense closed forest stands – as much as 25% of total annual precipitation. Interception loss is high in the beginning of storms and gradually decreases; the loss is of the order of 0.5 to 2 mm per shower and it is greater in the case of light showers than when rain is continuous. The percentage of total precipitation loss is highest; nearly 100% in light showers when the total rainfall is less than the interception capacity. Effective rain = Rainfall – Interception loss 4.1 4.1.1 Factors Affecting Interception ❖ Character of storm ❖ Wind speed ❖ Species, age, and density of prevailing vegetation cover ❖ Season of the year 4.1.2 Measurement of Interception It is measured by placing on the ground one or more rain gauges (interceptometers) under vegetation or trees and comparing the amount of precipitation thus collected with that of similar gauges in the open area, either above or at one side of the vegetation. Water loss by vegetation can be reduced by thinning or changing the vegetation type. 4.1.3 Calculation of Interception Loss Most interception loss develops during the initial storm period and the rate of interception rapidly approaches zero thereafter. The total storm interception can be estimated by using the empirical formula. Figure 4-1 shows the Horton’s mean curve of interception loss for different showers. 𝐿𝑖 = 𝑆𝑖 + 𝑘𝐸𝑡 4.2 42 | P a g e Where 𝐿𝑖 = total interception (cm); 𝑆𝑖 = interception storage capacity (cm); t = duration of storm (hrs); E = rate of evaporation from intercepting surfaces (cm/hr), k= ratio of surface area of intercepting leaves to horizontal projection of this area. Figure 4-1: Horton’s mean curve of interception loss for different showers. 4.2 Depression Storage Precipitation reaching the ground continues to enter the soil until the ratio of falling is more than the soil can accept. Excess water then begins to fill surface depressions. The water trapped in these depressions only escape by evaporation or infiltration. Factors Influencing Depression Storage 1. Type of soil 2. The condition of the surface (amount and nature of depression 3. Slope of the catchment 4. The soil moisture 4.2.1 Calculation The volume of water stored by surface depressions at any given time can be estimated by 𝑉 = 𝑆𝑑 (1 − 𝑒 −𝑘𝑃𝑒 ) 4.3 Where 43 | P a g e 𝑉 = actual volume storage (cm3 ) {at time of interest} 𝑆𝑑 = maximum storage capacity of the depression 𝑃𝑒 = rainfall excess {gross rainfall – (evaporation + interception + infiltration)} k = a constant equivalent to 1/𝑆𝑑 Depression loss and surface storage: Because a given depression will hold its maximum volume if horizontally oriented. 44 | P a g e 5 Evaporation and Transpiration 5.1 Evaporation It is the process by which water in its liquid (or solid state - sublimation) is transformed into the gaseous state (below boiling point) and transferred into the atmosphere. Evaporation involves the transfer of water from land and water masses of the earth into the atmosphere. Evaporation from water area is the process under which a portion of water (or ice) near the surface below the boiling point is changed into a gaseous state through the transfer of heat energy. Water molecules are in constant motion both in air and in water. At any instant, some of these molecules have sufficient energy to break through the water surface and escape into the air as vapour. At the same instant, some of the water molecules in the air above the water surface penetrate the water and remain within the water. Evaporation occurs when the number of molecules breaking free from the water surface into the air is larger than the number that gets entrapped in the water from the air. Thus, evaporation is the net rate at which water is transferred into the atmosphere. There are three main processes involved in evaporation ❖ vapour emission ❖ vapour removal ❖ vapour return Vapour emission is directly proportional to the maximum vapour pressure corresponding to the water temperature. Vapour removal takes place by the processes of diffusion, convection and wind action. Diffusion continues with or without wind action but convection takes place in the absence of wind. Vapour removal increases with wind velocities. Vapour return occurs when actual vapour pressure in the air is greater than the maximum vapour pressure corresponding to water–surface temperature. In the immediate vicinity of the water surface, a thin film of air exists whose temperature is intermediate between the air temperature and water temperature. 45 | P a g e When the air is colder than water, this intermediate thin film of air is warmed by the water and becomes lighter than the air above, thereby producing instability and convective vapour removal. Evaporation thus continues, even though overlying air is saturated. However, when air is warmer than water, the air film is cooled and becomes heavy and stable with intermediate temperature between the air and water. Unless prevented by wind, maximum vapour pressure in the thin air film approaches maximum vapour pressure corresponding to the air temperature as limiting value. 5.2 Factors Affecting Evaporation from Free Water Surface Several factors, both meteorological and physical, affect the rate of free water evaporation although it is often difficult to assess the relative importance of each of them. 5.2.1 METEOROLOGICAL FACTORS Radiation: Since evaporating water molecules require energy input (590 calories per gram) which comes from solar radiation, it is evident that solar radiation will be a factor of considerable importance which will set the broad limits of the rate of evaporation. In fact, solar radiation is now regarded as by far, the most important single factor in the evaporation process. Humidity: As air’s humidity rises, its ability to absorb more water vapour decreases and evaporation slows. Evaporation is generally inversely proportional to humidity. Temperature: The rate of emission of molecules from water is a function of its temperature. The higher the temperature, the greater is the rate of emission because the molecules have greater energy to escape. Evaporation increases with temperature of water surface due to increase in vapour pressure with temperature. Wind: Wind velocity is important in varying the rate of evaporation. As water vaporizes into the air, the boundary layer between the air and water becomes saturated. This saturated (thin film) layer must be removed and replaced continually by drier air if evaporation is to proceed. This movement of the saturated layer depends on turbulence, which is a function of wind. A change in wind speed by 10 percent increases evaporation by 1 to 3 percent. However, the effect of increasing wind speed is considered to be decreased as some high value is approached. The effect probably depends on surface roughness and dimension of water body. 46 | P a g e Atmospheric Pressure: Evaporation decreases with increase in atmospheric pressure. This is due to the fact that, increase in atmospheric pressure increases the number of air molecules per unit volume, and there are more chances that, vapour molecules escaping from water surface collide with an air molecule and get rebound into the liquid thereby reducing the rate of evaporation. 5.2.2 Geographical Factors Depth of Water body: The effect of water depth on the rate of evaporation may be quite considerable. There is relatively higher surface evaporation from shallow water depths as the amount of heat storage is negligible. Large, deep lakes, not only have a much higher capacity for heat storage than smaller water bodies, but in the middle and higher latitudes, experience marked thermal stratification, which also affects evaporation from their surfaces. Thus, during spring and summer, heat entering the water surface is mixed downward for only a limited distance by wind action. This results in the formation of an upper layer of water (the epilimnion), in which temperatures are relatively uniform and higher than those in the rest of the water body, and beneath which there is a thermocline, a water layer in which temperature decreases and density increases, rapidly with depth. With cooling of the surface waters (in winter) and their associated increase in density, the cooled surface water settles away from the surface and are replaced by warmer water from below. The turnover results in approximately uniform temperatures and densities throughout the depth of water, so that; turbulence resulting from wind action can easily activate deep mechanical mixing. There is, therefore, a slow release by the deep-water body of stored heat during autumn and winter, which is made available for evaporation. The net result of this heat storage on relative air and water temperatures is that water temperatures are lower than air temperatures during summer and higher than air temperatures during winter. This makes the rates of evaporation form water bodies higher in winters. Water surface area: The rate of evaporation shows an inverse proportionality to the surface area. The reason for this phenomenon is that, air moving across a large lake may have low water vapour content at the beginning but as it travels over the lake surface, its moisture content will gradually increase. Increasing moisture content means a decrease in the saturation deficit and hence a reciprocal decrease in the rate of evaporation. However, the total volume of water evaporated will almost cause an inevitably increase with size of the water surface. 47 | P a g e Water Quality: The rate of evaporation decreases by about 1% for every one percent (1%) increase in salinity. This reduction is brought about by the reduced vapour pressure if the saline water. The turbidity of water probably has little direct effect upon evaporation although by affecting the reflectivity of the water, and consequently its heat budget, and temperature, may have an indirect effect. 5.2.3 Methods Of Estimating Evaporation from Free-Water Bodies 5.2.3.1 Evaporation Using Pans The most common method of finding evaporation from a free – water body is by means of an evaporation pan, since there are no direct means to measure the exact quantum of evaporation from a water body. Evaporation pans are installed in the vicinity of the reservoir equipped with instruments to record meteorological data (such as wind speed, vapour pressure, temperature, humidity, precipitation). Observations are made regularly in the morning at 08:30hours, by measuring the difference in the observed levels adjusted for any precipitation during the interval. It has been observed that evaporation occurs more rapidly from pan than from larger bodies due to: i) heat storage ii) size iii) depth of water in pan iv) colour of pan This relationship holds for very long periods of observation. Estimates of reservoir evaporation based on short period pan observations (less than one year) may be seriously in error.Accordingly, a coefficient is applied to pan observation to derive the equivalent lake or reservoir evaporation given by: 𝐸𝐿 = 𝐾𝐸𝑃 5.1 Where 𝐸𝐿 – lake or reservoir evaporation 𝐸𝑃 – pan evaporation K – pan coefficient The pan coefficient k can be determined by a refined formula as: 𝑒 −𝑒 𝑘 = 𝐾′ 𝑒𝑠𝐿−𝑒𝑍 5.2 𝑠𝑝 𝑍 Where 48 | P a g e esL – saturation vapour pressure for maximum temperature just below the lake surface, esp – saturation vapour pressure for maximum temperature in evaporation pan, ez – mean vapour pressure t a height Z above the lake surface and K’ – a coefficient, mainly a function of the type of pan, equal to 1.5 for US Class A pan at Z=4m. 5.2.3.2 Types of Evaporimeters Evaporimeters are instruments for measuring the amount of water lost by evaporation per unit area in a given interval from a shallow container. Evaporimeter may be installed above or in the ground or may float on water surface of a reservoir. The most common types used are: Class A Land Pan This instrument is made of unpainted galvanized iron 1.2m in diameter and 25cm deep. The bottom, supported on wooden frame, is raised 15cm above the ground surface. The water surface, maintained between 5 and 8cm below the rim of the pan, is measured daily with a hook in a stilling well. The daily evaporation is computed as the difference between observed levels, corrected for any precipitation measured in an adjacent or nearby standard rain gauge. This pan has a pan coefficient of 0.6 to 0.8 with an average value of 0.7. Sunken Pan The pain is 1.8m in diameter and 0.6m deep, buried in the ground within 0cm of the top. The water surface is supposed to be not more than 1cm above the ground level or below it. Because of its size, this pan provides by far the best index to take evaporation. The pan coefficient is normally 0.95. Floating Pan The pan is a square of 0.9m side and 0.45m deep supported by drum floats in the centre of a raft 4.25 x 4.87m. The water level in the pan is the same as that of the surrounding water. The pan coefficient ranges from 0.70 to 0.82 with an average of 0.80. Advantages of the pan method 1. More data can be collected in a relatively short period. 2. Does not require sophisticated instrumentation. 3. Relatively cheap Disadvantages 1. Pan coefficient is difficult to determine accurately. 49 | P a g e 2. Often does not simulate conditions pertaining to reservoir. Piche Evaporimeter It is usually kept suspended in a Stevenson screen. It consists of a disc of filter paper kept constantly saturated with water from a graduated glass tube. The loss of water from the tube over a known period gives the average rate of evaporation. Though it is a simple instrument, the readings obtained are often more erratic than those from standard pans. 5.2.4 Measures to Reduce Lake Evaporation The following are some of the recommended measures to reduce evaporation from water surfaces: i. Storage reservoirs of more depth and less surface area, i.e., by choosing a cross section of the reservoir like a deep gorge Fig 5-1; while the surface water is exposed to temperature gradients the deeper waters are cool; from this standpoint a large reservoir is preferable to a number of small reservoirs (while it is the reverse from the point of flood control). ii. By growing tall trees like Causerina on the windward side of the reservoirs to act as wind breakers. iii. By spraying certain chemicals or fatty acids and formation of films. By spreading a manomolecular layer of acetyl alcohol (hexadecanol) C16H33OH over the reservoir surface (from boats)—a film is formed on the surface which is only 0.015 micron (approx.) in thickness. It is a polar compound, and it has great affinity for water on one side (hydrophylic) and repels water on the other side (hydrophobic). The film will only allow precipitation from the top into it but will not allow water molecules to escape from it. This method is readily effective when the wind velocities are less. If the wind velocity is more, it will sweep the film off the water surface and deposit it on the bank. However, the film is pervious to O2 and CO2. About 2.2 kg (22 N) of acetyl alcohol is required to cover an area of 1 ha of reservoir surface. It is best suited for small and medium-sized reservoirs. iv. By allowing the flow of water, temperature is reduced and evaporation is reduced; i.e., by designing the outlet works so that the warmer surface water can be released. v. By removing the water loving weeds and plants like Phreatophytes from the periphery of the reservoir. vi. By straightening the stream-channels the exposed area of the water surface (along the length) is reduced and hence evaporation is reduced. 50 | P a g e vii. By providing mechanical coverings like thin polythene sheets to small agricultural ponds and lakes. viii. By developing underground reservoirs, since the evaporation from a ground water table is very much less than the evaporation from a water surface. ix. If the reservoir is surrounded by huge trees and forest, the evaporation loss will be less due to cooler environment. 5.2.5 Analytical Methods 5.2.5.1 Water Budget (or Storage Equation) Approach This method is for reservoirs (e.g., lakes, pools etc.) and it is based on the hydrologic continuity equation. A balance sheet of all water entering and leaving a particular catchment is drawn. A close approximation to the amount of water arriving from the atmosphere can be made if rainfall is measured over the catchment on a regular and systematic basis. Regular stream gauging of streams draining the area will indicate the water leaving the area. The storage equation can be written as: 𝐸 = 𝑃 + 𝐼 − 𝑂 + 𝑈 + 𝛥𝑆 5.3 where E – evaporation from reservoir, P – Precipitation, I – surface inflow, O – surface outflow, U – (net) underground flow and ΔS – change in storage. Generally, the method is more likely to be used for estimating monthly or annual evaporation. Even though the water budget approach is simple in theory, reliable results are rarely obtained because it is always very difficult to make accurate estimate of the factors involved, especially the underground and storage components. 51 | P a g e 5.2.5.2 Energy Budget method This method, which is based on the conservation of heat energy, is rigorously correct in theory and most accurate in application for a period of a week or longer. However, the instruments required are very expensive, and observations and processing of the data are time consuming, and therefore makes it a specialist approach. In the method, the incoming and outgoing radiant energy, both long and short waves are accounted for in addition to changes in energy of the waterbody. The difference in energy contributes to evaporation, the amount of which is computed from the known latent heat of evaporation. The energy budget equation for evaporation from free water bodies can be expressed as: 𝑄𝑠 −𝑄𝑟 +𝑄𝑎 −𝑄𝑎𝑟 −𝑄𝑏𝑟 +𝑄𝑣 −𝑄𝑥 𝐸= 5.4 𝜌𝐿(1+𝐵) where Qs – incoming solar radiation, Qr – reflected solar radiation, Qa, - incoming long wave radiation, Qar – reflected long-wave radiation, Qbr – long-wave radiation from the water, Qv – net energy advected into the lake, Qx – increase in stored energy of water, L – latent heat of evaporation in energy per unit depth, B – Bowen ratio = Qh/Qe, Qh –energy exchanged from water to atmosphere as sensible heat, and Qe – energy lost by water by evaporation = density of water. The Bowen ratio B can be computed by: 0.61(𝑇𝑠 −𝑇𝑎 )𝑃 𝐵= (𝐸𝑠 −𝐸𝑎 )1000 5.5 where 52 | P a g e P –atmospheric pressure, Ta – temperature of air in oC, Ts – temperature of water in oC, Ea – pressure of air in millibar, Es – saturation vapour pressure corresponding to Ts in millibar. Values of B range from 0.58 to 0.66 depending on the stability of atmospheric conditions. Measurement required in this method include: i. incoming short-wave and long-wave radiation, ii. air and water temperature and dew point to compute the Bowen ratio and iii. temperature surveys