Helicopters PDF
Document Details
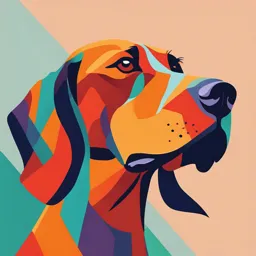
Uploaded by DaringDream
Tags
Summary
This document provides a detailed description of different aspects of helicopter design and function, covering topics like flight mechanics. It explains the concepts of Mach numbers and compressibility. The summary covers the assembly and rigging details of rotary-wing aircraft.
Full Transcript
As the speed of the object approaches the speed of molecules of the air. At hypersonic speeds, the sound, the flight Mach number is nearly equal to chemistry of the air must be considered when one, M = 1 (350–760 mph), and the flow is said...
As the speed of the object approaches the speed of molecules of the air. At hypersonic speeds, the sound, the flight Mach number is nearly equal to chemistry of the air must be considered when one, M = 1 (350–760 mph), and the flow is said determining forces on the object. When the space to be transonic. At some locations on the object, shuttle re-enters the atmosphere at high hypersonic the local speed of air exceeds the speed of sound. speeds, close to Mach 25, the heated air becomes an Compressibility effects are most important in ionized plasma of gas, and the spacecraft must be transonic flows and lead to the early belief in a sound insulated from the extremely high temperatures. barrier. Flight faster than sound was thought to be impossible. In fact, the sound barrier was only an Additional technical information pertaining to high-speed increase in the drag near sonic conditions because aerodynamics can be found at bookstores, libraries, and of compressibility effects. Because of the high drag numerous sources on the Internet. As the design of aircraft associated with compressibility effects, aircraft are evolves and the speeds of aircraft continue to increase into not operated in cruise conditions near Mach 1. the hypersonic range, new materials and propulsion systems Supersonic conditions occur for numbers greater will need to be developed. This is the challenge for engineers, than Mach 1, but less then Mach 3 (760–2,280 physicists, and designers of aircraft in the future. mph). Compressibility effects of gas are important in the design of supersonic aircraft because of the Rotary-Wing Aircraft Assembly & Rigging shockwaves that are generated by the surface of the The flight control units located in the flight deck of all object. For high supersonic speeds, between Mach 3 helicopters are very nearly the same. All helicopters have and Mach 5 (2,280–3,600 mph), aerodynamic heating either one or two of each of the following: collective pitch becomes a very important factor in aircraft design. control, throttle grip, cyclic pitch control, and directional control pedals. [Figure 2-22] Basically, these units do the For speeds greater than Mach 5, the flow is said to same things, regardless of the type of helicopter on which be hypersonic. At these speeds, some of the energy they are installed; however, the operation of the control of the object now goes into exciting the chemical system varies greatly by helicopter model. bonds which hold together the nitrogen and oxygen Cyclic control stick Controls attitude and direction of flight Throttle Controls rpm Collective pitch stick Controls altitude Pedals Maintain heading Figure 2-22. Controls of a helicopter and the principal function of each. 2-15 Rigging the helicopter coordinates the movements of the 2. The operation of interconnected control systems (engine flight controls and establishes the relationship between the throttle and collective pitch) is properly coordinated. main rotor and its controls, and between the tail rotor and its 3. The range of movement and neutral position of the controls. Rigging is not a difficult job, but it requires great pilot’s controls are correct. precision and attention to detail. Strict adherence to rigging procedures described in the manufacturer’s maintenance 4. The maximum and minimum pitch angles of the main manuals and service instructions is a must. Adjustments, rotor blades are within specified limits. This includes clearances, and tolerances must be exact. checking the fore-and-aft and lateral cyclic pitch and collective pitch blade angles. Rigging of the various flight control systems can be broken 5. The tracking of the main rotor blades is correct. down into the following three major steps: 6. In the case of multirotor aircraft, the rigging and 1. Placing the control system in a specific position— movement of the rotor blades are synchronized. holding it in position with pins, clamps, or jigs, then 7. When tabs are provided on main rotor blades, they are adjusting the various linkages to fit the immobilized correctly set. control component. 8. The neutral, maximum, and minimum pitch angles 2. Placing the control surfaces in a specific reference and coning angles of the tail rotor blades are correct. position—using a rigging jig, a precision bubble protractor, or a spirit level to check the angular 9. When dual controls are provided, they function difference between the control surface and some fixed correctly and in synchronization. surface on the aircraft. [Figure 2-23] Upon completion of rigging, a thorough check should be made 3. Setting the maximum range of travel of the various of all attaching, securing, and pivot points. All bolts, nuts, and components—this adjustment limits the physical rod ends should be properly secured and safetied as specified movement of the control system. in the manufacturers’ maintenance and service instructions. After completion of the static rigging, a functional check of Configurations of Rotary-Wing Aircraft the flight control system must be accomplished. The nature of the functional check varies with the type of helicopter and Autogyro system concerned, but usually includes determining that: An autogyro is an aircraft with a free-spinning horizontal rotor that turns due to passage of air upward through the 1. The direction of movement of the main and tail rotor rotor. This air motion is created from forward motion of the blades is correct in relation to movement of the pilot’s aircraft resulting from either a tractor or pusher configured controls. engine/propeller design. [Figure 2-24] Single Rotor Helicopter An aircraft with a single horizontal main rotor that provides Main rotor rigging protractor both lift and direction of travel is a single rotor helicopter. A secondary rotor mounted vertically on the tail counteracts CAUTION Make sure blade dampers are positioned against auto-rotation inboard stops 25° 20° 15° 10° 5° 0° 5° 10° 15° Figure 2-23. A typical rigging protractor. Figure 2-24. An autogyro. 2-16 the rotational force (torque) of the main rotor to correct yaw of the fuselage. [Figure 2-25] Pitch change axis Dual Rotor Helicopter An aircraft with two horizontal rotors that provide both the lift and directional control is a dual rotor helicopter. The rotors are counterrotating to balance the aerodynamic torque and eliminate the need for a separate antitorque system. [Figure 2-26] Flipping hinge Drag hinge Types of Rotor Systems Fully Articulated Rotor A fully articulated rotor is found on aircraft with more than two blades and allows movement of each individual blade in three directions. In this design, each blade can rotate about the pitch axis to change lift; each blade can move back and forth in plane, lead and lag; and flap up and down through a hinge independent of the other blades. [Figure 2-27] Semirigid Rotor Figure 2-27. Articulated rotor head. The semirigid rotor design is found on aircraft with two rotor blades. The blades are connected in a manner such that as Rigid Rotor one blade flaps up, the opposite blade flaps down. The rigid rotor system is a rare design but potentially offers the best properties of both the fully articulated and semirigid rotors. In this design, the blade roots are rigidly attached to the rotor hub. The blades do not have hinges to allow lead-lag or flapping. Instead, the blades accommodate these motions by using elastomeric bearings. Elastomeric bearings are molded, rubber-like materials that are bonded to the appropriate parts. Instead of rotating like conventional bearings, they twist and flex to allow proper movement of the blades. Forces Acting on the Helicopter One of the differences between a helicopter and a fixed-wing aircraft is the main source of lift. The fixed-wing aircraft derives its lift from a fixed airfoil surface while the helicopter Figure 2-25. Single rotor helicopter. derives lift from a rotating airfoil called the rotor. During hovering flight in a no-wind condition, the tip-path plane is horizontal, that is, parallel to the ground. Lift and thrust act straight up; weight and drag act straight down. The sum of the lift and thrust forces must equal the sum of the weight and drag forces in order for the helicopter to hover. During vertical flight in a no-wind condition, the lift and thrust forces both act vertically upward. Weight and drag both act vertically downward. When lift and thrust equal weight and drag, the helicopter hovers; if lift and thrust are less than weight and drag, the helicopter descends vertically; if lift and thrust are greater than weight and drag, the helicopter rises vertically. Figure 2-26. Dual rotor helicopter. 2-17 For forward flight, the tip-path plane is tilted forward, thus Torque Compensation tilting the total lift-thrust force forward from the vertical. This Newton’s third law of motion states “To every action there resultant lift-thrust force can be resolved into two components: is an equal and opposite reaction.” As the main rotor of a lift acting vertically upward and thrust acting horizontally in helicopter turns in one direction, the fuselage tends to rotate the direction of flight. In addition to lift and thrust, there is in the opposite direction. This tendency for the fuselage to weight, the downward acting force, and drag, the rearward rotate is called torque. Since torque effect on the fuselage is acting or retarding force of inertia and wind resistance. a direct result of engine power supplied to the main rotor, any change in engine power brings about a corresponding In straight-and-level, unaccelerated forward flight, lift equals change in torque effect. The greater the engine power, the weight and thrust equals drag. (Straight-and-level flight is greater the torque effect. Since there is no engine power flight with a constant heading and at a constant altitude.) being supplied to the main rotor during autorotation, there If lift exceeds weight, the helicopter climbs; if lift is less is no torque reaction during autorotation. than weight, the helicopter descends. If thrust exceeds drag, the helicopter increases speed; if thrust is less than drag, it The force that compensates for torque and provides for decreases speed. directional control can be produced by various means. The defining factor is dictated by the design of the helicopter, In sideward flight, the tip-path plane is tilted sideward in the some of which do not have a torque issue. Single main direction that flight is desired, thus tilting the total lift-thrust rotor designs typically have an auxiliary rotor located on vector sideward. In this case, the vertical or lift component the end of the tail boom. This auxiliary rotor, generally is still straight up, weight straight down, but the horizontal referred to as a tail rotor, produces thrust in the direction or thrust component now acts sideward with drag acting to opposite the torque reaction developed by the main rotor. the opposite side. [Figure 2-25] Foot pedals in the flight deck permit the pilot to increase or decrease tail rotor thrust, as needed, to neutralize For rearward flight, the tip-path plane is tilted rearward and torque effect. tilts the lift-thrust vector rearward. The thrust is then rearward and the drag component is forward, opposite that for forward Other methods of compensating for torque and providing flight. The lift component in rearward flight is straight up; directional control include the Fenestron® tail rotor system, weight, straight down. an SUD Aviation design that employs a ducted fan enclosed by a shroud. Another design, called NOTAR®, a McDonnell Douglas design with no tail rotor, employs air directed through a series of slots in the tail boom, with the balance Figure 2-28. Aerospatiale Fenestron tail rotor system (left) and the McDonnell Douglas NOTAR® System (right). 2-18 Axis New axis Old axis 90 Upward force applied here Reaction occurs here Gyro tips down here Gyro tips up here Figure 2-29. Gyroscopic precession principle. exiting through a 90° duct located at the rear of the tail boom. [Figure 2-28] The movement of the cyclic pitch control in a two-bladed rotor system increases the AOA of one rotor blade with Gyroscopic Forces the result that a greater lifting force is applied at this point The spinning main rotor of a helicopter acts like a gyroscope. in the plane of rotation. This same control movement As such, it has the properties of gyroscopic action, one of simultaneously decreases the AOA of the other blade a like which is precession. Gyroscopic precession is the resultant amount, thus decreasing the lifting force applied at this point action or deflection of a spinning object when a force is in the plane of rotation. The blade with the increased AOA applied to this object. This action occurs approximately 90° tends to rise; the blade with the decreased AOA tends to in the direction of rotation from the point where the force lower. However, gyroscopic precession prevents the blades is applied. [Figure 2-29] Through the use of this principle, from rising or lowering to maximum deflection until a point the tip-path plane of the main rotor may be tilted from the approximately 90° later in the plane of rotation. horizontal. In a three-bladed rotor, the movement of the cyclic pitch Examine a two-bladed rotor system to see how gyroscopic control changes the AOA of each blade an appropriate precession affects the movement of the tip-path plane. Moving amount so that the end result is the same, a tipping forward the cyclic pitch control increases the AOA of one rotor blade of the tip-path plane when the maximum change in AOA with the result that a greater lifting force is applied at that is made as each blade passes the same points at which the point in the plane of rotation. This same control movement maximum increase and decrease are made for the two- simultaneously decreases the AOA of the other blade the same bladed rotor as shown in Figure 2-30. As each blade passes amount, thus decreasing the lifting force applied at that point the 90° position on the left, the maximum increase in AOA in the plane of rotation. The blade with the increased AOA occurs. As each blade passes the 90° position to the right, tends to flap up; the blade with the decreased AOA tends to the maximum decrease in AOA occurs. Maximum deflection flap down. Because the rotor disc acts like a gyro, the blades takes place 90° later, maximum upward deflection at the rear reach maximum deflection at a point approximately 90° and maximum downward deflection at the front; the tip-path later in the plane of rotation. As shown in Figure 2-30, the plane tips forward. retreating blade AOA is increased and the advancing blade AOA is decreased resulting in a tipping forward of the tip-path Helicopter Flight Conditions plane, since maximum deflection takes place 90° later when Hovering Flight the blades are at the rear and front, respectively. In a rotor During hovering flight, a helicopter maintains a constant system using three or more blades, the movement of the cyclic position over a selected point, usually a few feet above the pitch control changes the AOA of each blade an appropriate ground. For a helicopter to hover, the lift and thrust produced amount so that the end result is the same. by the rotor system act straight up and must equal the weight 2-19 Low pitch applied High flap result Blade rotation Low flap result Blade rotation High pitch applied Figure 2-30. Gyroscopic precession. and drag, which act straight down. [Figure 2-31] While the engine turns the main rotor system in a counterclockwise hovering, the amount of main rotor thrust can be changed direction, the helicopter fuselage tends to turn clockwise. The to maintain the desired hovering altitude. This is done by amount of torque is directly related to the amount of engine changing the angle of incidence (by moving the collective) of power being used to turn the main rotor system. Remember, the rotor blades and hence the AOA of the main rotor blades. as power changes, torque changes. Changing the AOA changes the drag on the rotor blades, and the power delivered by the engine must change as well to keep the rotor speed constant. The weight that must be supported is the total weight of the helicopter and its occupants. If the amount of lift is greater than the actual weight, the helicopter accelerates upwards until the lift force equals the weight gain altitude; if thrust is less than weight, the helicopter accelerates downward. When operating near the ground, the effect of the closeness to the ground changes this response. The drag of a hovering helicopter is mainly induced drag incurred while the blades are producing lift. There is, however, some profile drag on the blades as they rotate through the air. Throughout the rest of this discussion, the term drag includes both induced and profile drag. An important consequence of producing thrust is torque. As discussed earlier, Newton’s third law states that for every Figure 2-31. To maintain a hover at a constant altitude, enough lift action there is an equal and opposite reaction. Therefore, as and thrust must be generated to equal the weight of the helicopter and the drag produced by the rotor blades. 2-20 To counteract this torque-induced turning tendency, an antitorque rotor or tail rotor is incorporated into most o helicopter designs. A pilot can vary the amount of thrust produced by the tail rotor in relation to the amount of torque produced by the engine. As the engine supplies more power to the main rotor, the tail rotor must produce more thrust to overcome the increased torque effect. This is done through Drift the use of antitorque pedals. Translating Tendency or Drift During hovering flight, a single main rotor helicopter tends to drift or move in the direction of tail rotor thrust. This drifting l tendency is called translating tendency. [Figure 2-32] Tail rotor downwash Tail rotor thrust To counteract this drift, one or more of the following features may be used. All examples are for a counterclockwise rotating main rotor system. Figure 2-32. A tail rotor is designed to produce thrust in a direction opposite torque. The thrust produced by the tail rotor is sufficient The main transmission is mounted at a slight angle to to move the helicopter laterally. the left (when viewed from behind) so that the rotor mast has a built-in tilt to oppose the tail rotor thrust. which means a higher pitch angle, and more power is needed Flight controls can be rigged so that the rotor disc is to move the air down through the rotor. tilted to the right slightly when the cyclic is centered. Whichever method is used, the tip-path plane is tilted Ground effect is at its maximum in a no-wind condition over slightly to the left in the hover. a firm, smooth surface. Tall grass, rough terrain, and water If the transmission is mounted so that the rotor shaft surfaces alter the airflow pattern, causing an increase in rotor is vertical with respect to the fuselage, the helicopter tip vortices. [Figure 2-33] “hangs” left skid low in the hover. The opposite is true for rotor systems turning clockwise when viewed from Coriolis Effect (Law of Conservation of Angular above. Momentum) In forward flight, the tail rotor continues to push to The Coriolis effect is also referred to as the law of the right, and the helicopter makes a small angle with conservation of angular momentum. It states that the value the wind when the rotors are level and the slip ball is of angular momentum of a rotating body does not change in the middle. This is called inherent sideslip. unless an external force is applied. In other words, a rotating body continues to rotate with the same rotational velocity Ground Effect until some external force is applied to change the speed of When hovering near the ground, a phenomenon known rotation. Angular momentum is moment of inertia (mass as ground effect takes place. This effect usually occurs at times distance from the center of rotation squared) multiplied heights between the surface and approximately one rotor by speed of rotation. Changes in angular velocity, known as diameter above the surface. The friction of the ground angular acceleration and deceleration, take place as the mass causes the downwash from the rotor to move outwards from of a rotating body is moved closer to or further away from the helicopter. This changes the relative direction of the the axis of rotation. The speed of the rotating mass increases downwash from a purely vertical motion to a combination or decreases in proportion to the square of the radius. An of vertical and horizontal motion. As the induced airflow excellent example of this principle is a spinning ice skater. through the rotor disc is reduced by the surface friction, the The skater begins rotation on one foot, with the other leg lift vector increases. This allows a lower rotor blade angle for and both arms extended. The rotation of the skater’s body is the same amount of lift, which reduces induced drag. Ground relatively slow. When a skater draws both arms and one leg effect also restricts the generation of blade tip vortices due to inward, the moment of inertia (mass times radius squared) the downward and outward airflow making a larger portion becomes much smaller and the body is rotating almost faster of the blade produce lift. When the helicopter gains altitude than the eye can follow. Because the angular momentum vertically, with no forward airspeed, induced airflow is no must remain constant (no external force applied), the angular longer restricted, and the blade tip vortices increase with velocity must increase. The rotor blade rotating about the the decrease in outward airflow. As a result, drag increases rotor hub possesses angular momentum. As the rotor begins 2-21 to cone due to G-loading maneuvers, the diameter or the disc shrinks. Due to conservation of angular momentum, the blades continue to travel the same speed even though the blade tips have a shorter distance to travel due to Vertical ascent reduced disc diameter. The action results in an increase in rotor rpm. Most pilots arrest this increase with an increase in collective pitch. Conversely, as G-loading subsides and the rotor disc flattens out from the loss of G-load induced coning, the blade tips now have a longer distance to travel at the same tip speed. This action results in a reduction of rotor rpm. However, if this drop in the rotor rpm continues to the point at which it attempts to decrease below normal operating rpm, the engine control system adds more fuel/ power to maintain the specified engine rpm. If the pilot does not reduce collective pitch as the disc unloads, the combination of engine compensation for the rpm slow down and the additional pitch as G-loading increases may result in exceeding the torque limitations or power the engines Figure 2-34. To ascend vertically, more lift and thrust must be can produce. generated to overcome the forces of weight and drag. lift-thrust force can be resolved into two components—lift Vertical Flight acting vertically upward and thrust acting horizontally in the Hovering is actually an element of vertical flight. Increasing direction of flight. In addition to lift and thrust, there is weight the AOA of the rotor blades (pitch) while keeping their (the downward acting force) and drag (the force opposing the rotation speed constant generates additional lift and the motion of an airfoil through the air). [Figure 2-35] helicopter ascends. Decreasing the pitch causes the helicopter to descend. In a no wind condition, when lift and thrust are In straight-and-level (constant heading and at a constant less than weight and drag, the helicopter descends vertically. altitude), unaccelerated forward flight, lift equals weight If lift and thrust are greater than weight and drag, the and thrust equals drag. If lift exceeds weight, the helicopter helicopter ascends vertically. [Figure 2-34] accelerates vertically until the forces are in balance; if thrust is less than drag, the helicopter slows until the forces are in Forward Flight balance. As the helicopter moves forward, it begins to lose In steady forward flight with no change in airspeed or vertical altitude because lift is lost as thrust is diverted forward. speed, the four forces of lift, thrust, drag, and weight must However, as the helicopter begins to accelerate, the rotor be in balance. Once the tip-path plane is tilted forward, the system becomes more efficient due to the increased airflow. total lift-thrust force is also tilted forward. This resultant The result is excess power over that which is required to Out of Ground Effect (OGE) In Ground Effect (IGE) No Large blade tip vortex wind hover Blade tip vortex Downwash pattern equidistant 360° Figure 2-33. Air circulation patterns change when hovering out of ground effect (OGE) and when hovering in ground effect (IGE). 2-22 Resultant 800 Maximum continuous power available Lift Increasing power for decreasing airspeed 600 Thrust C A B 400 Drag Increasing power for Helicopter movement decreasing airspeed 200 Maximum Weight continuous level (horizontal) Resultant Minimum power flight for level flight (VY) airspeed (VH) 0 Figure 2-35. The power required to maintain a straight-and-level 20 40 60 80 100 120 flight and a stabilized airspeed. hover. Continued acceleration causes an even larger increase Figure 2-36. Changing force vectors results in aircraft movement. in airflow through the rotor disc and more excess power. In order to maintain unaccelerated flight, the pilot must not make any changes in power or in cyclic movement. Any Effective Translational Lift (ETL) such changes would cause the helicopter to climb or descend. While transitioning to forward flight at about 16–24 knots, Once straight-and-level flight is obtained, the pilot should the helicopter experiences effective translational lift (ETL). make note of the power (torque setting) required and not As mentioned earlier in the discussion on translational lift, make major adjustments to the flight controls. [Figure 2-36] the rotor blades become more efficient as forward airspeed increases. Between 16–24 knots, the rotor system completely Translational Lift outruns the recirculation of old vortices and begins to work Improved rotor efficiency resulting from directional flight is in relatively undisturbed air. The flow of air through the called translational lift. The efficiency of the hovering rotor rotor system is more horizontal, therefore induced flow system is greatly improved with each knot of incoming wind and induced drag are reduced. The AOA is subsequently gained by horizontal movement of the aircraft or surface increased, which makes the rotor system operate more wind. As incoming wind produced by aircraft movement or efficiently. This increased efficiency continues with increased surface wind enters the rotor system, turbulence and vortices airspeed until the best climb airspeed is reached, and total are left behind and the flow of air becomes more horizontal. drag is at its lowest point. In addition, the tail rotor becomes more aerodynamically efficient during the transition from hover to forward flight. As speed increases, translational lift becomes more effective, Translational thrust occurs when the tail rotor becomes more the nose rises or pitches up, and the aircraft rolls to the right. aerodynamically efficient during the transition from hover The combined effects of dissymmetry of lift, gyroscopic to forward flight. As the tail rotor works in progressively precession, and transverse flow effect cause this tendency. It is less turbulent air, this improved efficiency produces more important to understand these effects and anticipate correcting antitorque thrust, causing the nose of the aircraft to yaw left for them. Once the helicopter is transitioning through ETL, (with a main rotor turning counterclockwise) and forces the the pilot needs to apply forward and left lateral cyclic input to pilot to apply right pedal (decreasing the AOA in the tail maintain a constant rotor-disc attitude. [Figure 2-39] rotor blades) in response. In addition, during this period, the airflow affects the horizontal components of the stabilizer Dissymmetry of Lift found on most helicopters which tends to bring the nose Dissymmetry of lift is the differential (unequal) lift between of the helicopter to a more level attitude. Figure 2-37 and advancing and retreating halves of the rotor disc caused by the Figure 2-38 show airflow patterns at different speeds and different wind flow velocity across each half. This difference how airflow affects the efficiency of the tail rotor. in lift would cause the helicopter to be uncontrollable in any situation other than hovering in a calm wind. There must 2-23 1–5 knots Figure 2-37. The airflow pattern for 1–5 knots of forward airspeed. Note how the downwind vortex is beginning to dissipate and induced flow down through the rear of the rotor system is more horizontal. Airflow pattern just prior to effective translational lift 10–15 knots Figure 2-38. An airflow pattern at a speed of 10–15 knots. At this increased airspeed, the airflow continues to become more horizontal. The leading edge of the downwash pattern is being overrun and is well back under the nose of the helicopter. be a means of compensating, correcting, or eliminating this unequal lift to attain symmetry of lift. More horizontal No recirculation flow of air of air When the helicopter moves through the air, the relative airflow through the main rotor disc is different on the advancing side than on the retreating side. The relative wind encountered by the advancing blade is increased by the forward speed 16–24 knots of the helicopter; while the relative windspeed acting on the retreating blade is reduced by the helicopter’s forward airspeed. Therefore, as a result of the relative windspeed, the advancing blade side of the rotor disc produces more lift Reduced induced flow Tail rotor operates in increases angle of attack relatively clean air than the retreating blade side. [Figure 2-40] If this condition was allowed to exist, a helicopter with a Figure 2-39. Effective translational lift is easily recognized in actual flight by a transient induced aerodynamic vibration and increased counterclockwise main rotor blade rotation would roll to the performance of the helicopter. left because of the difference in lift. In reality, the main rotor blades flap and feather automatically to equalize lift across the rotor disc. Articulated rotor systems, usually with three or 2-24 Direction of Flight As the rotor blade reaches the advancing side of the rotor disc, it reaches its maximum upward flapping velocity. Retreating Side Advancing Side [Figure 2-41A] When the blade flaps upward, the angle between the chord line and the resultant relative wind decreases. This decreases the AOA, which reduces the Blade tip Blade tip amount of lift produced by the blade. At position C, the rotor Relative wind Relative wind speed speed minus plus blade is at its maximum downward flapping velocity. Due helicopter helicopter to downward flapping, the angle between the chord line and speed speed the resultant relative wind increases. This increases the AOA (200 knots) (400 knots) and thus the amount of lift produced by the blade. The combination of blade flapping and slow relative wind Forward flight at 100 knots acting on the retreating blade normally limits the maximum forward speed of a helicopter. At a high forward speed, the Figure 2-40. The blade tip speed of this helicopter is approximately retreating blade stalls due to high AOA and slow relative 300 knots. If the helicopter is moving forward at 100 knots, the wind speed. This situation is called “retreating blade relative windspeed on the advancing side is 400 knots. On the stall” and is evidenced by a nose-up pitch, vibration, and retreating side, it is only 200 knots. This difference in speed causes a dissymmetry of lift. a rolling tendency—usually to the left in helicopters with counterclockwise blade rotation. Pilots can avoid retreating more blades, incorporate a horizontal hinge (flapping hinge) blade stall by not exceeding the never-exceed speed. This to allow the individual rotor blades to move, or flap up and speed is designated VNE and is indicated on a placard and down as they rotate. A semirigid rotor system (two blades) marked on the airspeed indicator by a red line. utilizes a teetering hinge, which allows the blades to flap as a unit. When one blade flaps up, the other blade flaps down. During aerodynamic flapping of the rotor blades as they B Angle of attack over nose Resultant relative wind B C Angle of attack at 9 o’clock position A Angle of attack at 3 o’clock position C A Downflap velocity Upflap velocity D D Angle of attack over tail Relative wind Angle of attack Resultant relative wind Figure 2-41. The combined upward flapping (reduced lift) of the advancing blade and downward flapping (increased lift) of the retreating blade equalizes lift across the main rotor disc counteracting dissymmetry of lift. 2-25 compensate for dissymmetry of lift, the advancing blade the rotor system must be tilted in the desired direction of achieves maximum upward flapping displacement over the movement. Cyclic feathering changes the angle of incidence nose and maximum downward flapping displacement over differentially around the rotor system. Forward cyclic the tail. This causes the tip-path plane to tilt to the rear and movements decrease the angle of incidence at one part on is referred to as blowback. Figure 2-42 shows how the rotor the rotor system while increasing the angle at another part. disc is originally oriented with the front down following Maximum downward flapping of the blade over the nose and the initial cyclic input. As airspeed is gained and flapping maximum upward flapping over the tail tilt both rotor disc and eliminates dissymmetry of lift, the front of the disc comes thrust vector forward. To prevent blowback from occurring, up, and the back of the disc goes down. This reorientation the pilot must continually move the cyclic forward as the of the rotor disc changes the direction in which total rotor velocity of the helicopter increases. Figure 2-42 illustrates thrust acts; the helicopter’s forward speed slows, but can be the changes in pitch angle as the cyclic is moved forward at corrected with cyclic input. The pilot uses cyclic feathering to increased airspeeds. At a hover, the cyclic is centered and compensate for dissymmetry of lift allowing them to control the pitch angle on the advancing and retreating blades is the the attitude of the rotor disc. same. At low forward speeds, moving the cyclic forward reduces pitch angle on the advancing blade and increases Cyclic feathering compensates for dissymmetry of lift pitch angle on the retreating blade. This causes a slight (changes the AOA) in the following way. At a hover, equal rotor tilt. At higher forward speeds, the pilot must continue lift is produced around the rotor system with equal pitch and to move the cyclic forward. This further reduces pitch angle AOA on all the blades and at all points in the rotor system on the advancing blade and further increases pitch angle on (disregarding compensation for translating tendency). The the retreating blade. As a result, there is even more tilt to the rotor disc is parallel to the horizon. To develop a thrust force, rotor than at lower speeds. This horizontal lift component (thrust) generates higher helicopter airspeed. The higher airspeed induces blade flapping to maintain symmetry of lift. The combination of flapping and cyclic feathering maintains symmetry of lift and desired attitude on the rotor system and helicopter. Autorotation Autorotation is the state of flight in which the main rotor system of a helicopter is being turned by the action of air moving up through the rotor rather than engine power driving the rotor. [Figure 2-43] In normal, powered flight, air is drawn into the main rotor system from above and exhausted downward, but Figure 2-42. To compensate for blowback, move the cyclic forward. during autorotation, air moves up into the rotor system from Blowback is more pronounced with higher airspeeds. below as the helicopter descends. Autorotation is permitted Normal Powered Flight Autorotation Direction of flight Figure 2-43. During an autorotation, the upward flow of relative wind permits the main rotor blades to rotate at their normal speed. In effect, the blades are “gliding” in their rotational plane. 2-26 Pitch link The stationary swash plate is mounted around the main rotor mast and connected to the cyclic and collective controls Stationary swash plate by a series of pushrods. It is restrained from rotating by an antidrive link but is able to tilt in all directions and move vertically. The rotating swash plate is mounted to the stationary swash plate by a uniball sleeve. It is connected to the mast by drive links and is allowed to rotate with the main Rotating swash plate rotor mast. Both swash plates tilt and slide up and down as one unit. The rotating swash plate is connected to the pitch Control rod horns by the pitch links. Figure 2-44. Stationary and rotating swash plate. There are three major controls in a helicopter that the pilot must use during flight. They are the collective pitch control, mechanically by a freewheeling unit, which is a special clutch cyclic pitch control, and antitorque pedals or tail rotor control. mechanism that allows the main rotor to continue turning even In addition to these major controls, the pilot must also use the if the engine is not running. If the engine fails, the freewheeling throttle control, which is mounted directly to the collective unit automatically disengages the engine from the main rotor pitch control in order to fly the helicopter. allowing the main rotor to rotate freely. It is the means by which a helicopter can be landed safely in the event of an Collective Pitch Control engine failure; consequently, all helicopters must demonstrate The collective pitch control is located on the left side of the this capability in order to be certificated. pilot’s seat and is operated with the left hand. The collective is used to make changes to the pitch angle of all the main Rotorcraft Controls rotor blades simultaneously, or collectively, as the name Swash Plate Assembly implies. As the collective pitch control is raised, there is a The purpose of the swash plate is to transmit control inputs simultaneous and equal increase in pitch angle of all main from the collective and cyclic controls to the main rotor rotor blades; as it is lowered, there is a simultaneous and blades. It consists of two main parts: the stationary swash equal decrease in pitch angle. [Figure 2-45] This is done plate and the rotating swash plate. [Figure 2-44] through a series of mechanical linkages, and the amount Figure 2-45. Raising the collective pitch control increases the pitch angle by the same amount on all blades. 2-27 of movement in the collective lever determines the amount should be made with smooth pressure. of blade pitch change. An adjustable friction control helps prevent inadvertent collective pitch movement. In piston helicopters, the collective pitch is the primary control for manifold pressure, and the throttle is the primary Throttle Control control for rpm. However, the collective pitch control also The function of the throttle is to regulate engine rpm. If the influences rpm, and the throttle also influences manifold correlator or governor system does not maintain the desired pressure; therefore, each is considered to be a secondary rpm when the collective is raised or lowered, or if those control of the other’s function. Both the tachometer (rpm systems are not installed, the throttle must be moved manually indicator) and the manifold pressure gauge must be analyzed with the twist grip to maintain rpm. The throttle control is to determine which control to use. Figure 2-47 illustrates much like a motorcycle throttle, and works almost the same this relationship. way; twisting the throttle to the left increases rpm, twisting the throttle to the right decreases rpm. [Figure 2-46] Cyclic Pitch Control The cyclic pitch control is mounted vertically from the flight Governor/Correlator deck floor, between the pilot’s legs or, in some models, A governor is a sensing device that senses rotor and engine rpm between the two pilot seats. [Figure 2-48] This primary flight and makes the necessary adjustments in order to keep rotor rpm control allows the pilot to fly the helicopter in any horizontal constant. Once the rotor rpm is set in normal operations, the direction; fore, aft, and sideways. The total lift force is always governor keeps the rpm constant, and there is no need to make perpendicular to the tip-path place of the main rotor. The any throttle adjustments. Governors are common on all turbine purpose of the cyclic pitch control is to tilt the tip-path plane helicopters (as it is a function of the fuel control system of the in the direction of the desired horizontal direction. The cyclic turbine engine), and used on some piston-powered helicopters. control changes the direction of this force and controls the attitude and airspeed of the helicopter. A correlator is a mechanical connection between the collective lever and the engine throttle. When the collective The rotor disc tilts in the same direction the cyclic pitch lever is raised, power is automatically increased and when control is moved. If the cyclic is moved forward, the rotor lowered, power is decreased. This system maintains rpm disc tilts forward; if the cyclic is moved aft, the disc tilts close to the desired value, but still requires adjustment of aft, and so on. Because the rotor disc acts like a gyro, the the throttle for fine tuning. mechanical linkages for the cyclic control rods are rigged in such a way that they decrease the pitch angle of the rotor Some helicopters do not have correlators or governors and blade approximately 90° before it reaches the direction of require coordination of all collective and throttle movements. cyclic displacement, and increase the pitch angle of the When the collective is raised, the throttle must be increased; rotor blade approximately 90° after it passes the direction of when the collective is lowered, the throttle must be decreased. displacement. An increase in pitch angle increases AOA; a As with any aircraft control, large adjustments of either decrease in pitch angle decreases AOA. For example, if the collective pitch or throttle should be avoided. All corrections cyclic is moved forward, the AOA decreases as the rotor blade passes the right side of the helicopter and increases on the left side. This results in maximum downward deflection of the If manifold and rpm is Solution pressure is Twist grip throttle Increasing the throttle increases LOW LOW manifold pressure and rpm Lowering the collective pitch HIGH LOW decreases manifold pressure and increases rpm Raising the collective pitch LOW HIGH increases manifold pressure and decreases rpm Reducing the throttle decreases HIGH HIGH manifold pressure and rpm Figure 2-46. A twist grip throttle is usually mounted on the end of the collective lever. The throttles on some turbine helicopters are Figure 2-47. Relationship between manifold pressure, rpm, mounted on the overhead panel or on the floor in the flight deck. collective, and throttle. 2-28 Cyclic pitch control Cyclic pitch control Figure 2-49. Antitorque pedals compensate for changes in torque and control heading in a hover. flight, as well as while taxiing with the forward gear off the ground. With the right pedal displaced forward, the forward rotor disc tilts to the right, while the aft rotor disc tilts to the left. The opposite occurs when the left pedal is pushed forward; the forward rotor disc inclines to the left, and the aft rotor disc tilts to the right. Differing combinations of pedal Figure 2-48. The cyclic pitch control may be mounted vertically and cyclic application can allow the tandem rotor helicopter between the pilot’s knees or on a teetering bar from a single cyclic to pivot about the aft or forward vertical axis, as well as located in the center of the helicopter. The cyclic can pivot in all pivoting about the center of mass. directions. Stabilizer Systems rotor blade in front of the helicopter and maximum upward deflection behind it, causing the rotor disc to tilt forward. Bell Stabilizer Bar System Arthur M. Young discovered that stability could be increased Antitorque Pedals significantly with the addition of a stabilizer bar perpendicular The antitorque pedals are located on the cabin floor by the to the two blades. The stabilizer bar has weighted ends, which pilot’s feet. They control the pitch and, therefore, the thrust of cause it to stay relatively stable in the plane of rotation. The the tail rotor blades. [Figure 2-49] Newton’s third law applies stabilizer bar is linked with the swash plate in a manner that to the helicopter fuselage and how it rotates in the opposite reduces the pitch rate. The two blades can flap as a unit and, direction of the main rotor blades unless counteracted and therefore, do not require lag-lead hinges (the whole rotor slows controlled. To make flight possible and to compensate for this down and accelerates per turn). Two-bladed systems require torque, most helicopter designs incorporate an antitorque rotor a single teetering hinge and two coning hinges to permit or tail rotor. The antitorque pedals allow the pilot to control modest coning of the rotor disc as thrust is increased. The the pitch angle of the tail rotor blades which in forward flight configuration is known under multiple names, including Hiller puts the helicopter in longitudinal trim and while at a hover, panels, Hiller system, Bell-Hiller system, and flybar system. enables the pilot to turn the helicopter 360°. The antitorque pedals are connected to the pitch change mechanism on the Offset Flapping Hinge tail rotor gearbox and allow the pitch angle on the tail rotor The offset flapping hinge is offset from the center of the rotor blades to be increased or decreased. hub and can produce powerful moments useful for controlling the helicopter. The distance of the hinge from the hub (the Helicopters that are designed with tandem rotors do not have offset) multiplied by the force produced at the hinge produces an antitorque rotor. These helicopters are designed with both a moment at the hub. Obviously, the larger the offset, the rotor systems rotating in opposite directions to counteract the greater the moment for the same force produced by the blade. torque, rather than using a tail rotor. Directional antitorque pedals are used for directional control of the aircraft while in The flapping motion is the result of the constantly changing 2-29 balance between lift, centrifugal, and inertial forces. This or lateral. A 1/rev is caused simply by one blade developing rising and falling of the blades is characteristic of most more lift at a given point than the other blade develops at helicopters and has often been compared to the beating of the same point. a bird’s wing. The flapping hinge, together with the natural flexibility found in most blades, permits the blade to droop Medium Frequency Vibration considerably when the helicopter is at rest and the rotor is not Medium frequency vibration (4/rev and 6/rev) is another turning over. During flight, the necessary rigidity is provided vibration inherent in most rotors. An increase in the level of by the powerful centrifugal force that results from the rotation these vibrations is caused by a change in the capability of the of the blades. This force pulls outward from the tip, stiffening fuselage to absorb vibration, or a loose airframe component, the blade, and is the only factor that keeps it from folding up. such as the skids, vibrating at that frequency. Stability Augmentation Systems (SAS) High Frequency Vibration Some helicopters incorporate stability augmentation systems High frequency vibrations can be caused by anything in the (SAS) to help stabilize the helicopter in flight and in a hover. helicopter that rotates or vibrates at extremely high speeds. The simplest of these systems is a force trim system, which A high frequency vibration typically occurs when the tail uses a magnetic clutch and springs to hold the cyclic control in rotor gears, tail drive shaft or the tail rotor engine, fan or the position at which it was released. More advanced systems shaft assembly vibrates or rotates at an equal or greater speed use electric actuators that make inputs to the hydraulic servos. than the tail rotor. These servos receive control commands from a computer that senses helicopter attitude. Other inputs, such as heading, Rotor Blade Tracking speed, altitude, and navigation information may be supplied Blade tracking is the process of determining the positions to the computer to form a complete autopilot system. The of the tips of the rotor blade relative to each other while the SAS may be overridden or disconnected by the pilot at any rotor head is turning, and of determining the corrections time. SAS reduces pilot workload by improving basic aircraft necessary to hold these positions within certain tolerances. control harmony and decreasing disturbances. These systems The blades should all track one another as closely as possible. are very useful when the pilot is required to perform other The purpose of blade tracking is to bring the tips of all blades duties, such as sling loading and search and rescue operations. into the same tip path throughout their entire cycle of rotation. Various methods of blade tracking are explained below. Helicopter Vibration The following paragraphs describe the various types of Flag & Pole vibrations. Figure 2-50 shows the general levels into which The flag and pole method, as shown in Figure 2-51, shows frequencies are divided. the relative positions of the rotor blades. The blade tips are marked with chalk or a grease pencil. Each blade tip should Extreme Low Frequency Vibration be marked with a different color so that it is easy to determine Extreme low frequency vibration is pretty well limited to pylon the relationship of the other tips of the rotor blades to each rock. Pylon rocking (two to three cycles per second) is inherent other. This method can be used on all types of helicopters with the rotor, mast, and transmission system. To keep the that do not have jet propulsion at the blade tips. Refer to vibration from reaching noticeable levels, transmission mount the applicable maintenance manual for specific procedures. dampening is incorporated to absorb the rocking. Electronic Blade Tracker Low Frequency Vibration The most common electronic blade tracker consists of Low frequency vibrations (1/rev and 2/rev) are caused by the a Balancer/Phazor, Strobex tracker, and Vibrex tester. rotor itself. 1/rev vibrations are of two basic types: vertical [Figures 2-52 through 2-54] The Strobex blade tracker permits blade tracking from inside or outside the helicopter while on the ground or inside the helicopter in flight. The Helicopter Vibration Types system uses a highly concentrated light beam flashing in Frequency Level Vibration sequence with the rotation of the main rotor blades so that a Extreme low frequency Less than 1/rev PYLON ROCK fixed target at the blade tips appears to be stopped. Each blade Low frequency 1/rev or 2/rev type vibration is identified by an elongated retroreflective number taped or Medium frequency Generally 4, 5, or 6/rev attached to the underside of the blade in a uniform location. High frequency Tail rotor speed or faster When viewed at an angle from inside the helicopter, the taped numbers will appear normal. Tracking can be accomplished Figure 2-50. Various helicopter vibration types. with tracking tip cap reflectors and a strobe light. The tip 2-30 Curtain Curtain Leading edge Blade BLADE Pole Position of chalk mark (approximately 2 inches long) Pole Handle Line parallel to longitudinal axis of helicopter Curtain 1/2" max spread (typical) Approximate position of chalk marks Pole Figure 2-51. Flag and pole blade tracking. Strobe flash tube RPM dial Meter A Channel B A B TRACK COMMON MAGNETIC FUNCTION Band-pass filter PICKUP BALANCER MODEL 177M-6A RPM TONE X10 X1 X100 PUSH FOR SCALE 2 RPM RANGE Phase meter 12 11 1 DOUBLE TEST 10 2 PHAZOR 9 3 8 4 7 5 6 Figure 2-52. Balancer/Phazor. Figure 2-53. Strobex tracker. 2-31 Interrupter plate Motor Figure 2-54. Vibrex tracker. caps are temporarily attached to the tip of each blade. The high-intensity strobe light flashes in time with the rotating blades. The strobe light operates from the aircraft electrical power supply. By observing the reflected tip cap image, it is possible to view the track of the rotating blades. Tracking is Figure 2-55. Tail rotor tracking. accomplished in a sequence of four separate steps: ground tracking, hover verification, forward flight tracking, and maintenance manual. Run engine with pedals in neutral autorotation rpm adjustment. position. Reset marking device on underside of tail boom assembly. Slowly move marking device into disc of tail rotor Tail Rotor Tracking approximately one inch from tip. When near blade is marked, The marking and electronic methods of tail rotor tracking stop engine and allow rotor to stop. Repeat this procedure are explained in the following paragraphs. until tracking mark crosses over to the other blade, then extend pitch control link of unmarked blade one half turn. Marking Method Procedures for tail rotor tracking using the marking method, Electronic Method as shown in Figure 2-55, are as follows: The electronic Vibrex balancing and tracking kit is housed in a carrying case and consists of a Model 177M-6A Balancer, After replacement or installation of tail rotor hub, a Model 135M-11 Strobex, track and balance charts, an blades, or pitch change system, check tail rotor rigging accelerometer, cables, and attaching brackets. and track tail rotor blades. Tail rotor tip clearance shall be set before tracking and checked again after tracking. The Vibrex balancing kit is used to measure and indicate the level of vibration induced by the main rotor and tail rotor of The strobe-type tracking device may be used if a helicopter. The Vibrex analyzes the vibration induced by available. Instructions for use are provided with the out-of-track or out-of-balance rotors, and then by plotting device. Attach a piece of soft rubber hose six inches vibration amplitude and clock angle on a chart the amount long on the end of a ½ × ½ inch pine stick or other and location of rotor track or weight change is determined. In flexible device. Cover the rubber hose with Prussian addition, the Vibrex is used in troubleshooting by measuring blue or similar type of coloring thinned with oil. the vibration levels and frequencies or rpm of unknown disturbances. Note: Ground run-up shall be performed by authorized personnel only. Start engine in accordance with applicable 2-32 Rotor Blade Preservation & Storage Accomplish the following requirements for rotor blade Intake valve Exhaust valve preservation and storage: Condemn, demilitarize, and dispose of locally any blade which has incurred nonrepairable damage. Tape all holes in the blade, such as tree damage, or foreign object damage (FOD) to protect the interior Spark plug Piston of the blade from moisture and corrosion. Thoroughly remove foreign matter from the entire exterior surface of blade with mild soap and water. Protect blade outboard eroded surfaces with a light coating of corrosion preventive or primer coating. Protect blade main bolt hole bushing, drag brace Crankshaft Connecting rod retention bolt hole bushing, and any exposed bare metal (i.e., grip and drag pads) with a light coating of 1. Intake 2. Compression corrosion preventive. Secure blade to shock-mounted support and secure container lid. Place copy of manufacturer’s blade records, containing information required by Title 14 of the Code of Federal Regulations (14 CFR) section 91.417(a)(2)(ii), and any other blade records in a waterproof bag and insert into container record tube. Obliterate old markings from the container that pertained to the original shipment or to the original item it contained. Annotate the blade model, part number (P/N) and serial number, as applicable, on 3. Power 4. Exhaust the outside of the container. Figure 2-56. The arrows indicate the direction of motion of the Helicopter Power Systems crankshaft and piston during the four-stroke cycle. Powerplant The two most common types of engines used in helicopters When the piston moves away from the cylinder head on the are the reciprocating engine and the turbine engine. intake stroke, the intake valve opens and a mixture of fuel Reciprocating engines, also called piston engines, are and air is drawn into the combustion chamber. As the cylinder generally used in smaller helicopters. Most training moves back toward the cylinder head, the intake valve closes, helicopters use reciprocating engines because they are and the air-fuel mixture is compressed. When compression relatively simple and inexpensive to operate. Turbine is nearly complete, the spark plugs fire and the compressed engines are more powerful and are used in a wide variety of mixture is ignited to begin the power stroke. The rapidly helicopters. They produce a tremendous amount of power expanding gases from the controlled burning of the air-fuel for their size but are generally more expensive to operate. mixture drive the piston away from the cylinder head, thus providing power to rotate the crankshaft. The piston then Reciprocating Engine moves back toward the cylinder head on the exhaust stroke The reciprocating engine consists of a series of pistons where the burned gases are expelled through the opened connected to a rotating crankshaft. As the pistons move up exhaust valve. Even when the engine is operated at a fairly and down, the crankshaft rotates. The reciprocating engine low speed, the four-stroke cycle takes place several hundred gets its name from the back-and-forth movement of its times each minute. In a four-cylinder engine, each cylinder internal parts. The four-stroke engine is the most common operates on a different stroke. Continuous rotation of a type, and refers to the four different cycles the engine crankshaft is maintained by the precise timing of the power undergoes to produce power. [Figure 2-56] strokes in each cylinder. 2-33 Turbine Engine helicopters have a “burn off” capability and attempt to correct The gas turbine engine mounted on most helicopters is the situation without pilot action. If the problem cannot be made up of a compressor, combustion chamber, turbine, corrected on its own, the pilot must refer to the emergency and accessory gearbox assembly. The compressor draws procedures for that particular helicopter. filtered air into the plenum chamber and compresses it. The compressed air is directed to the combustion section through Main Rotor Transmission discharge tubes where atomized fuel is injected into it. The The primary purpose of the main rotor transmission is air-fuel mixture is ignited and allowed to expand. This to reduce engine output rpm to optimum rotor rpm. This combustion gas is then forced through a series of turbine reduction is different for the various helicopters. As an wheels causing them to turn. These turbine wheels provide example, suppose the engine rpm of a specific helicopter power to both the engine compressor and the accessory is 2,700. A rotor speed of 450 rpm would require a 6:1 gearbox. Power is provided to the main rotor and tail rotor reduction. A 9:1 reduction would mean the rotor would turn systems through the freewheeling unit which is attached at 300 rpm. Most helicopters use a dual-needle tachometer to the accessory gearbox power output gear shaft. The or a vertical scale instrument to show both engine and rotor combustion gas is finally expelled through an exhaust outlet. rpm or a percentage of engine and rotor rpm. The rotor rpm [Figure 2-57] indicator normally is used only during clutch engagement to monitor rotor acceleration, and in autorotation to maintain Transmission System rpm within prescribed limits. [Figure 2-58] The transmission system transfers power from the engine to the main rotor, tail rotor, and other accessories during normal In helicopters with horizontally mounted engines, another flight conditions. The main components of the transmission purpose of the main rotor transmission is to change the axis of system are the main rotor transmission, tail rotor drive rotation from the horizontal axis of the engine to the vertical system, clutch, and freewheeling unit. The freewheeling unit, axis of the rotor shaft. [Figure 2-59] or autorotative clutch, allows the main rotor transmission to drive the tail rotor drive shaft during autorotation. Helicopter Clutch transmissions are normally lubricated and cooled with their In a conventional airplane, the engine and propeller are own oil supply. A sight gauge is provided to check the directly connected. However, in a helicopter there is a oil level. Some transmissions have chip detectors located different relationship between the engine and the rotor. in the sump. These detectors are wired to warning lights Because of the greater weight of a rotor in relation to the located on the pilot’s instrument panel that illuminate in the power of the engine, as compared to the weight of a propeller event of an internal problem. The chip detectors on modern and the power in an airplane, the rotor must be disconnected from the engine when the starter is engaged. A clutch allows Gearbox Centrifugal Compression Section Turbine Section Combustion Section Section Exhaust air outlet N2 Rotor Stator N1 Rotor Compressor rotor Igniter plug Air inlet Fuel nozzle Gear Inlet air Compressor discharge air Output Shaft Combustion liner Combustion gases Exhaust gases Figure 2-57. Many helicopters use a turboshaft engine as shown above to drive the main transmission and rotor systems. The main difference between a turboshaft and a turbojet engine is that most of the energy produced by the expanding gases is used to drive a turbine rather than producing thrust through the expulsion of exhaust gases. 2-34 transmission and rotor system dragging it down. As the gas pressure increases through the power turbine, the rotor blades 20 E R begin to turn, slowly at first and then gradually accelerate to 15 25 3 10 2 4 30 110 100 110 100 normal operating rpm. 90 90 1 5 80 80 RPM 70 70 5 X100 35 On reciprocating helicopters, the two main types of clutches 60 60 50 50 ROTOR 0 40 ENGINE % RPM are the centrifugal clutch and the belt drive clutch. % RPM Centrifugal Clutch 120 110 The centrifugal clutch is made up of an inner assembly and 105 an outer drum. The inner assembly, which is connected to 60 50 ROTOR 80 70 100 the engine driveshaft, consists of shoes lined with material 40 PEEVER TURBINEA 90 similar to automotive brake linings. At low engine speeds, 95 30 100 springs hold the shoes in, so there is no contact with the outer 90 20 10 PERCENT RPM 110 80 drum, which is attached to the transmission input shaft. As 120 70 0 60 engine speed increases, centrifugal force causes the clutch 40 0 shoes to move outward and begin sliding against the outer NR NP drum. The transmission input shaft begins to rotate, causing the rotor to turn, slowly at first, but increasing as the friction increases between the clutch shoes and transmission drum. Figure 2-58. There are various types of dual-needle tachometers; As rotor speed increases, the rotor tachometer needle shows however, when the needles are superimposed, or married, the ratio of the engine rpm is the same as the gear reduction ratio. an increase by moving toward the engine tachometer needle. When the two needles are superimposed, the engine and the