HBF-ii_2024-L8_notes-chung PDF
Document Details
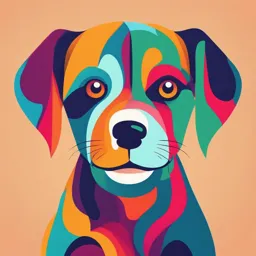
Uploaded by FruitfulIntegral
Wayne State University
2024
Charles S Chung
Tags
Related
- Lecture 12 Introduction to the Cardiovascular System PDF
- HBF-ii 2024-L6 pt1 notes-chung PDF
- Human Body Foundations II: Cardiac Electrophysiology and Cardiac Function (Physiology) 2024 PDF
- Human Body Foundations II: Cardiac Electrophysiology and Cardiac Function (Physiology) 2024 PDF
- Physiologie Cardiovasculaire - Généralités sur la Circulation - Assabar PDF
- DSA 11 Electrophysiology PDF
Summary
These notes cover the topic of cardiac electrophysiology and cardiac function, specifically focusing on excitation-contraction coupling, cytosolic calcium regulation, and related processes within cardiac myocytes. The notes are part of a larger curriculum, and they are designed to prepare students for further study in the subject.
Full Transcript
Human Body Foundations II: Cardiac Electrophysiology and Cardiac Function (Physiology) 2024 Faculty Charles S Chung PhD Associate Professor of Physiology [email protected] Note: In most cases, if a statement text is in a gray color, it is either an exercise or additional information. Throughout...
Human Body Foundations II: Cardiac Electrophysiology and Cardiac Function (Physiology) 2024 Faculty Charles S Chung PhD Associate Professor of Physiology [email protected] Note: In most cases, if a statement text is in a gray color, it is either an exercise or additional information. Throughout the notes, essential concepts are underlined, but studying all components is important to prepare for additional topics. Lecture 8-Cardiac Function (Cellular) Learning Objectives Describe Excitation-Contraction coupling of cardiac myocytes Describe the mechanisms of cytosolic calcium release and how it may be regulated Describe the mechanisms of thin filament activation and how it may be regulated Describe tension generation by the Myosin ATPase and how it may be regulated Define cardiac load Define the molecular mechanisms that provide passive force Describe how Length Dependent Activation changes the tension a myocyte develops and how it differs from filament overlap 1 Suggested Review Topics Cardiomyocyte Structure Nucleus/nuclei T-tubules Sarcoplasmic Reticulum Mitochondria (~30% cell) Sarcomere (~60% cell) Thin-filament Actin Troponin Complex (TnC, TnT, TnI) Tropomyosin Thick filament Myosin heads Light Chains, other regulatory proteins Striations I-band Isotropic band A-band Anisotropic band Thick filament Does not change length H-zone Thick filament without actin overlap M-line (M-disk) Z-line (Z-disk) Length-tension relationship Excitation-Contraction Coupling Tension cannot be generated without a change in cytosolic calcium, which does not occur without depolarization. Excitation (depolarization) couples with contraction (tension) because depolarization changes calcium. Excitation-contraction coupling describes how myocyte depolarization and calcium release leads to contraction, or the generation of tension (force). Thus, the steps maybe summarized as: Depolarization RyR calcium-induced calcium release Thin filament activation Myosin binding, ATPase cycle SR calcium uptake Thin filament deactivation Reduction in myosin binding 2 Bers Nature 415, 198-205 (2002) Figure: Excitation-Contraction coupling describes how the depolarization (excitation) leads to contraction (tension generation). Cytosolic Calcium Depolarization allows extracellular calcium to enter the cell Calcium-induced-calcium release occurs (RyR-2) Cytosolic calcium rises from ~10-7 M to nearly 10-5 M SERCA removes cytosolic calcium, regulated by PLB Depolarization occurs near sarcoplasmic reticulum (SR) T-tubules are essential to keep the action potential near the SR Cardiac muscle expresses the RyR-2 gene Skeletal muscle Ryanodine Receptor 2 (RyR2) allows for calcium-induced-calcium release Not coupled to T-Tubule like RyR1 T-tubules take action potentials and calcium deep inside the muscle RyR-2 releases calcium via calcium-induced-calcium release Calcium sequestered back to SR Calcium is sequestered back to the sarcoplasmic reticulum via the Sarco-Endoplasmic Reticulum Calcium ATPase (SERCA) SERCA is regulated by Phospholamban (PLB, sometimes PLN) More active SERCA (less interaction with PLB) means more calcium in SR 3 Differences between cardiac and Skeletal Muscle: Cardiac Muscle: RyR2 (ryanodine receptor) is not mechanically coupled to the T-tubule RyR2 requires calcium calcium influx through L-type calcium channels Calcium release from SR causes a large increase in cytosolic (intracellular) calcium “Calcium induced calcium release” Skeletal muscle: T-tubules couple to the Sarcoplasmic Reticulum via the DHC receptor (tetrad of L-type calcium channels) When the L-type calcium channel is activated (membrane depolarization), mechanical coupling to the RyR1 (ryanodine receptor) causes cytosolic calcium release Does not require inward calcium movement (calcium influx) to cause RyR1 activation Regulation of Calcium Release: RyR2 ATP and caffeine also make opening more likely Can be phosphorylated to phosphorylated (PKA) to make opening more likely Reuptake: SERCA Regulated by Phospholamban (PLB, sometimes PLN) PLB itself is regulated by PKA, PKA phosphorylation prevents PLB from inhibiting SERCA Increased SERCA activity means more calcium can be sequestered in the SR, causing a greater calcium release Contraction Two steps to achieve contraction: Thin Filament Activation Calcium binds Opens the binding sites Myosin ATPase Myosin binds to actin Generates force 4 Thin Filament Activation See HBF-I Muscle Lectures for details, including the 3 subunits of the troponin complex As calcium enters the cytosol, calcium diffuses and binds to calcium binding sites on troponin. This causes a conformational change in troponin, which removes an inhibitory lock that was blocking binding sites on actin, and promotes this by pushing tropomyosin aside to make myosin binding easier. Thus, calcium has opened the binding sites. Regulation of this process can be done via: More cytosolic calcium=more available binding sites to generate tension As calcium is removed, binding sites will close Troponin complex PKA phosphorylation increases calcium dissociation rate of TnC subunit Other phosphorylation can change tropomyosin inhibition Tropomyosin Difficult to pharmacologically regulate, but mutations can change its movement Impacts cooperative-activation Cooperative activation is the opening of a nearby binding site when myosin binds Can regulate deactivation of the thin fiament Myosin ATPase (contraction) If the thin filament is activated, the myosin ATPase will cycle, generating tension. i.e. actin filaments and therefore z-disks will be pulsed towards the m-line. The myosin ATPase (crossbridge) cycle includes steps such as: myosin hydrolyzing ATP to ADP-Pi, myosin binding actin, the power stroke where Pi and ADP are released, the rigor bride, ATP binding and myosin release from actin. A prior example of regulation of the myosin ATPase was shown in Dr. Ram’s Muscle Physiology lecture. There are multiple muscle fiber types and the myosin ATPase isozyme activity is different for each one. Therefore certain skeletal muscles can contract faster or slower. Cardiac muscle can be similarly regulated. Cardiac muscle has to myosin isotypes α-MHC (fast) MYH6 gene ATPase and power stroke cycle quickly β-MHC (slow) MYH7 gene Type I skeletal myosin ATPase and power stroke cycle more slowly and generate more force Humans typically have about a 50/50 split of α- vs β-MHC isoforms. Disease can lead to an even higher expression of this isoform. Thus, disease itself can lead to regulation of contraction. Note: small animals like rodents typically express the α-MHC isoform, almost exclusively. Their heart rates are faster. Why might isotypes be important? 5 The ATPase can also be regulated without modifying the isoform. The myosin heads may self-regulate. There is research into a “super relaxed” (SRX) state where the ATPase activity is blocked. This might be related to a structural change known as the “Interacting Heads Motif”. Regardless, this is related to a recently approved FDA drug. The research name was Mavacamten but the current trade name is Camzyos. This was FDA Approved in April 2022 and is the first ever pharmacological treatment for obstructive hypertrophic cardiomyopathy. Its mechanism of action is to reduce the amount of force (contraction) generated. There are two other regulatory proteins that interact with myosin you may hear about. Boron 9-5; Pful and Gautel J Muscle Res Cel Motility 33, 83-94-2012 Figure: Left: localization of myosin light chains. Right: schematic of interactions of myosin binding protein C with myosin and the thin filament. Myosin light chains bind to the myosin molecule. There are two forms, Essential and Regulatory light chains. Because they bind near where myosin head bend, they can impact myosin binding to actin and the myosin ATPase. They can be phosphorylated. Myosin Binding Protein-C is associated with several cardiomyopathies. It is unusual in that it might impact thin filament activation (opening of binding sites) and could inhibit the myosin ATPase. Interestingly, these two effects alternate when this protein is phosphorylated or not. 6 Cardiac Load Load is alternately referred to for muscle length or tension. Boron 9-9 FIGURE: Left: an example of a muscle starting at a fixed length (preload) but facing an isometric afterload remains isometric. Right: an example of a muscle starting at a given preload (length) but facing a constant tension (isotonic) afterload (weight). Preload is the load on the muscle prior to excitation, i.e. the pre-contraction load. Preload is usually the length of the muscle before contraction (instead of tension) Afterload is the load on the muscle after contraction has started. Afterload is usually the tension on the muscle after contraction (instead of length, especially since the length may change). Afterload will be used interchangeably with pressure, i.e. arterial pressure when referring to the heart. Preload Mechanisms and Stiffness Two microstructures/proteins define the major properties of the muscle at a given preload, titin and the extracellular matrix (collagen). Titin Titin is a giant molecular spring that lies across the sarcomere. The important part is in the I- band, where titin will resist stretch and try to restore length if it is compressed. Titin is associated clinically with dilated cardiomyopathies. Titin’s properties can be regulated by changing its stiffness. The most substantial way to change stiffness is to change the isoform. Titin can also be phosphorylated. 7 Long isoform Picrosirius red stained myocardium Short isoform Bright-field (collagen=red) Polarized light (collagen=green) Granzier and Labeit Circ Res 2004; Shirani J am Coll Cardiol 35 2000 36-44 FIGURE: Left: Titin (blue/orange in schematic at top) acts as a spring. Changing the isoform (or post-translational modifications like phosphorylation) can change the stiffness. i.e. it will regulate how much tension is generated at a specific preload (length), or it will make it harder or easier to stretch. Right: Picrosirus red stains collagen showing that it surrounds cells. Collagen (Extracellular Matrix) Collagen (along with other proteins like elastin) make up the extracellular matrix. It surrounds myocytes (as the name might suggest), mostly aligning along them, but also across them. Collagen can be deposited more heavily (or less) or crosslinked, which will make it hard to stretch (increase its stiffness. Since both Collagen and Titin are elastic, they both contribute to passive stiffness (i.e. the tension at a specific preload/length). Titin is thought to contribute more at physiological preloads. 8 Relationship between Length and Tension – Length Dependent Activation Since the overlap of the thick filament that contains myosin and the thin filament that contains actin alters the number of binding sites, we know that if you open all binding sites, the tension is related to the length (preload). This is true in skeletal muscle, especially at maximal activation. 100 80 Tension 60 Reduced calcium? 40 20 0 % Length 80 100 120 1.6 2.0 2.4 Approx cardiac Sarcomere Length [um] FIGURE: Left: Tension is skeletal muscle scales with the overlap of the thin and thick filaments. Right: The predicted length-tension curve for a cardiac muscle. Cardiac muscles do not go through the full length tension curve, instead it really only goes to lo, the length at peak tension. But if calcium is reduced, for example during a twitch, does the tension scale in cardiac muscle as it would in skeletal muscle? If an experiment is performed on a muscle at different preloads (length) with maximal calcium, a muscle will generate more force when the length is longer. A twitch contraction occurs at a lower calcium. If filament overlap (preload) were the only mechanism for how much tension is generated, then we would assume that the tension would be reduced by the same proportion, i.e. when normalized, the tensions would be equal. However, in experiments, longer lengths generate more tension than would be predicted if we simply scaled the length-tension curve. This is unexpected and called Length dependent activation. The slope of the relationship between the length and tension is an index of contractility, so a higher slope will correspond to a higher contractility. 9 Higher slope (solid line) than predicted (dashed line) because of Length Dependent Activation Tension Actual tension Low Calcium High Calcium Slope ~contractility Tension Expected from LDA % Length 80 100 120 1.6 2.0 2.4 Low Calcium High Calcium Approx cardiac Sarcomere Length [um] Sequeira et al Circulation Research. 2013;112:1491–1505 FIGURE: Left: example experiment showing the tension at a given calcium for a muscle at two sarcomere lengths (different preload). If filament overlap were the sole determinant of force, then the normalized (relative) forces should be equal at the same sarcomere length. However, it is clear here that it is different. Right: The tension-length relationship. Then the expected contractility would be along the scaled length-tension relationship (dashed purple line). However, at a longer length, the actual tension is above the expected, so the slope of the relationship becomes higher (solid purple line). This reflects length dependent activation. The relationship between length and tension is thus relatively simple. Passive forces, i.e. the forces measured in resting (hyperpolarized cells), are mostly defined by titin and collagen. Developed tension are defined by the myosin ATPase, therefore the length tension relationship can be regulated. Myosin ATPase 100 80 Tension 60 Titin 40 Collagen Basal contractions 20 0 % Length 80 100 120 1.6 2.0 2.4 Approx cardiac Sarcomere Length [um] FIGURE: How the length tension relationship of myocytes would look, and its molecular contributions. 10