Guyton and Hall Physiology Chapter 50 - The Eye I. PDF
Document Details
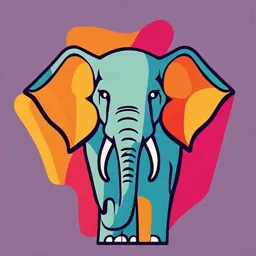
Uploaded by PrizeMeerkat
Tags
Related
Summary
This document details the physical principles of optics, focusing on light refraction and the optical system of the human eye. It explores the concepts of refractive indices, lenses (convex and concave), and how they work to form images.
Full Transcript
CHAPTER 50 UNIT X The Eye: I. Optics of Vision PHYSICAL PRINCIPLES OF O...
CHAPTER 50 UNIT X The Eye: I. Optics of Vision PHYSICAL PRINCIPLES OF OPTICS ratio of the two refractive indices of the two transparent media; and (2) the degree of angulation between the inter- Understanding the optical system of the eye requires face and the entering wave front. familiarity with the basic principles of optics, including such factors as the physics of light refraction, focusing, Application of Refractive Principles to Lenses and depth of focus. A brief review of these physical prin- Convex Lens Focuses Light Rays. Figure 50-2 shows ciples is presented in this chapter, followed by discussion parallel light rays entering a convex lens. The light rays of the optics of the eye. passing through the center of the lens strike the lens ex- actly perpendicular to the lens surface and, therefore, pass Refraction of Light through the lens without being refracted. Toward either edge of the lens, however, the light rays strike a progres- Refractive Index of a Transparent Substance. Light rays sively more angulated interface. The outer rays bend more travel through air at a velocity of about 300,000 km/sec, but and more toward the center, which is called convergence of they travel much slower through transparent solids and liq- uids. The refractive index of a transparent substance is the A ratio of the velocity of light in air to the velocity in the sub- stance. The refractive index of air is 1.00. Thus, if light trav- els through a particular type of glass at a velocity of 200,000 km/sec, the refractive index of this glass is 300,000 divided by 200,000, or 1.50. Refraction of Light Rays at an Interface Between Two Media With Different Refractive Indices. When light rays Wave fronts Glass traveling forward in a beam (as shown in Figure 50-1A) B strike an interface that is perpendicular to the beam, the rays enter the second medium without deviating from their course. The only effect that occurs is decreased velocity of transmission and shorter wavelength, as shown in the fig- ure by the shorter distances between wave fronts. If the light rays pass through an angulated interface, as shown in Figure 50-1B, the rays bend if the refractive in- Figure 50-1. Light rays entering a glass surface perpendicular to the dices of the two media are different from each other. In this light rays (A) and a glass surface angulated to the light rays (B). This figure, the light rays are leaving air, which has a refractive in- figure demonstrates that the distance between waves after they en- dex of 1.00, and are entering a block of glass having a refrac- ter the glass is shortened to about two-thirds that in air. It also shows tive index of 1.50. When the beam first strikes the angulated that light rays striking an angulated glass surface are bent. interface, the lower edge of the beam enters the glass ahead of the upper edge. The wave front in the upper portion of the beam continues to travel at a velocity of 300,000 km/sec, whereas that which entered the glass travels at a velocity of 200,000 km/sec. This difference in velocity causes the upper portion of the wave front to move ahead of the lower portion so that the wave front is no longer vertical but is angulated to the right. Because the direction in which light travels is al- ways perpendicular to the plane of the wave front, the direc- tion of travel of the light beam bends downward. Light from Focal length This bending of light rays at an angulated interface is distant source known as refraction. Note particularly that the degree of Figure 50-2. Bending of light rays at each surface of a convex spheri- refraction increases as a function of the following: (1) the cal lens showing that parallel light rays are focused to a focal point. 627 UNIT X The Nervous System: B. The Special Senses A Light from distant source Figure 50-3. Bending of light rays at each surface of a concave spherical lens showing that parallel light rays are diverged. the rays. Half the bending occurs when the rays enter the B lens, and half occurs as the rays exit from the opposite side. If the lens has exactly the proper curvature, parallel light rays passing through each part of the lens will be bent ex- actly enough so that all the rays will pass through a single point, called the focal point. Concave Lens Diverges Light Rays. Figure 50-3 shows the effect of a concave lens on parallel light rays. The rays that enter the center of the lens strike an interface that is perpendicular to the beam and, therefore, do not refract. The rays at the edge of the lens enter the lens ahead of the rays in the center. This effect is opposite to the effect in Figure 50-4. A, Point focus of parallel light rays by a spherical con- the convex lens, and it causes the peripheral light rays to vex lens. B, Line focus of parallel light rays by a cylindrical convex lens. diverge from the light rays that pass through the center of the lens. Thus, the concave lens diverges light rays, but the cylindrical lenses at right angles to each other. The vertical convex lens converges light rays. cylindrical lens converges the light rays that pass through Cylindrical Lens Bends Light Rays in Only One Plane— the two sides of the lens, and the horizontal lens converges Comparison With Spherical Lenses. Figure 50-4 shows the top and bottom rays. Thus, all the light rays come to both a convex spherical lens and a convex cylindrical lens. a single point focus. In other words, two cylindrical lenses Note that the cylindrical lens bends light rays from the two crossed at right angles to each other perform the same func- sides of the lens but not from the top or the bottom—that tion as one spherical lens of the same refractive power. is, bending occurs in one plane but not the other. Thus, parallel light rays are bent to a focal line. Conversely, light Focal Length of a Lens rays that pass through the spherical lens are refracted at all The distance beyond a convex lens at which parallel rays edges of the lens (in both planes) toward the central ray, converge to a common focal point is called the focal length and all the rays come to a focal point. of the lens. The diagram at the top of Figure 50-6 demon- The cylindrical lens is well demonstrated by using a test strates this focusing of parallel light rays. tube full of water. If the test tube is placed in a beam of sun- In the middle diagram, the light rays that enter the con- light and a piece of paper is brought progressively closer to vex lens are not parallel but are diverging because the origin the opposite side of the tube, a certain distance will be found of the light is a point source not far away from the lens. at which the light rays come to a focal line. The spherical lens Because these rays are diverging outward from the point is demonstrated by an ordinary magnifying glass. If such a source, they do not focus at the same distance away from lens is placed in a beam of sunlight, and a piece of paper is the lens as do parallel rays. In other words, when rays of brought progressively closer to the lens, the light rays will light that are already diverging enter a convex lens, the dis- impinge on a common focal point at an appropriate distance. tance of focus on the other side of the lens is farther from Concave cylindrical lenses diverge light rays in only one the lens than the focal length of the lens for parallel rays. plane in the same manner that convex cylindrical lenses The bottom diagram of Figure 50-6 shows light rays converge light rays in one plane. Figure 50-5A shows how diverging toward a convex lens that has far greater curva- light is focused from a point source to a line focus by a cy- ture than that of the other two lenses in the figure. In this lindrical lens. diagram, the distance from the lens at which the light rays Combination of Two Cylindrical Lenses at Right Angles come to focus is exactly the same as that from the lens in the Equals a Spherical Lens. Figure 50-5B shows two convex first diagram, in which the lens is less convex but the rays 628 CHAPTER 50 The Eye: I. Optics of Vision A entering it are parallel. This demonstrates that both parallel Line focus rays and diverging rays can be focused at the same distance beyond a lens, provided that the lens changes its convexity. Formation of an Image by a Convex Lens Figure 50-7A shows a convex lens with two point sources UNIT X of light to the left. Because light rays pass through the center of a convex lens without being refracted in either direction, the light rays from each point source of light are shown to Point source of light come to a point focus on the opposite side of the lens di- rectly in line with the point source and the center of the lens. Any object in front of the lens is, in reality, a mosaic of point sources of light. Some of these points are very bright and some are very weak, and they vary in color. Each point source of light on the object comes to a separate point focus on the opposite side of the lens in line with the lens center. If a white sheet of paper is placed at the focus distance from the lens, one can see an image of the object, as demonstrat- ed in Figure 50-7B. However, this image is upside down with respect to the original object, and the two lateral sides B Point source of light of the image are reversed. The lens of a camera focuses im- ages on film via this method. Point focus Measurement of the Refractive Power of a Lens— Diopter Figure 50-5. A, Focusing of light from a point source to a line focus The more a lens bends light rays, the greater is its “refrac- by a cylindrical lens. B, Two cylindrical convex lenses at right angles to tive power.” This refractive power is measured in terms each other, demonstrating that one lens converges light rays in one of diopters. The refractive power in diopters of a convex plane, and the other lens converges light rays in the plane at a right lens is equal to 1 meter divided by its focal length. Thus, a angle. The two lenses combined give the same point focus as that spherical lens that converges parallel light rays to a focal obtained with a single spherical convex lens. point 1 meter beyond the lens has a refractive power of +1 diopter, as shown in Figure 50-8. If the lens is capable of bending parallel light rays twice as much as a lens with a power of +1 diopter, it is said to have a strength of +2 diopters, and the light rays come to a focal point 0.5 me- ter beyond the lens. A lens capable of converging parallel light rays to a focal point only 10 centimeters (0.10 meter) beyond the lens has a refractive power of +10 diopters. The refractive power of concave lenses cannot be stated in terms of the focal distance beyond the lens because the light rays diverge rather than focus to a point. However, if a concave lens diverges light rays at the same rate that a 1-diopter convex lens converges them, the concave lens is said to have a dioptric Light from distant source strength of −1. Likewise, if the concave lens diverges light rays as much as a +10-diopter lens converges them, this lens is said to have a strength of −10 diopters. Focal Concave lenses “neutralize” the refractive power of con- points vex lenses. Thus, placing a 1-diopter concave lens imme- diately in front of a 1-diopter convex lens results in a lens Point source system with zero refractive power. The strengths of cylindrical lenses are computed in the same manner as the strengths of spherical lenses, ex- cept that the axis of the cylindrical lens must be stated in addition to its strength. If a cylindrical lens focuses parallel light rays to a line focus 1 meter beyond the lens, it has a strength of +1 diopter. Conversely, if a cylindri- Figure 50-6. The two upper lenses of this figure have the same focal length, but the light rays entering the top lens are parallel, whereas cal lens of a concave type diverges light rays as much as those entering the middle lens are diverging. The effect of parallel a +1-diopter cylindrical lens converges them, it has a versus diverging rays on the focal distance is shown. The bottom lens strength of −1 diopter. If the focused line is horizontal, has far more refractive power than either of the other two lenses (i.e., its axis is said to be 0 degrees. If it is vertical, its axis is it has a much shorter focal length), demonstrating that the stronger 90 degrees. the lens, the nearer to the lens is the focal point. 629 UNIT X The Nervous System: B. The Special Senses A Point sources Focal points B Figure 50-7. A, Two point sources of light focused at two separate points on opposite sides of the lens. B, Formation of an image by a convex spherical lens. Total refractive power = 59 diopters 1 diopter Image Object 2 diopters Vitreous Lens Aqueous Cornea Air 10 humor 1.40 humor 1.38 1.00 diopters 1.34 1.33 Figure 50-9. The eye as a camera. The numbers are the refractive indices. 1 meter Figure 50-8. Effect of lens strength on the focal distance. is considered to exist, with its central point 17 millimeters in front of the retina and a total refractive power of 59 di- OPTICS OF THE EYE opters when the lens is accommodated for distant vision. The lens system of the eye (Figure 50-9) is composed of About two-thirds of the 59 diopters of refractive power four refractive interfaces: (1) the interface between air of the eye is provided by the anterior surface of the cor- and the anterior surface of the cornea; (2) the interface nea (not by the eye lens). The principal reason for this between the posterior surface of the cornea and the aque- phenomenon is that the refractive index of the cornea is ous humor; (3) the interface between the aqueous humor markedly different from that of air, whereas the refractive and the anterior surface of the lens of the eye; and (4) the index of the eye lens is not greatly different from the indi- interface between the posterior surface of the lens and the ces of the aqueous humor and vitreous humor. vitreous humor. The internal index of air is 1, the cornea, The total refractive power of the internal lens of the eye, 1.38, the aqueous humor, 1.33, the crystalline lens (on as it normally lies in the eye surrounded by fluid on each average), 1.40, and the vitreous humor, 1.34. side, is only 20 diopters, about one third the total refractive power of the eye. However, the importance of the internal Consideration of All Refractive Surfaces of the Eye as lens is that in response to nervous signals from the brain, a Single Lens—The “Reduced” Eye. If all the refractive its curvature can be increased markedly to provide “accom- surfaces of the eye are added together algebraically and modation,” which is discussed later in the chapter. then considered to be one single lens, the optics of the nor- mal eye may be simplified and represented schematically Formation of an Image on the Retina. In the same man- as a “reduced eye.” This representation is useful in simple ner that a glass lens can focus an image on a sheet of paper, calculations. In the reduced eye, a single refractive surface the lens system of the eye can focus an image on the retina. 630 CHAPTER 50 The Eye: I. Optics of Vision The image is inverted and reversed with respect to the object. Sclerocorneal Cornea However, the mind perceives objects in the upright position junction despite the upside-down orientation on the retina because Meridional the brain is trained to consider an inverted image as normal. fibers Sclera MECHANISM OF “ACCOMMODATION” UNIT X In children, the refractive power of the lens of the eye Lens can be increased voluntarily from 20 diopters to about 34 diopters, which is an “accommodation” of 14 diopters. Suspensory Circular To make this accommodation, the shape of the lens is ligaments fibers changed from that of a moderately convex lens to that of Choroid Retina a very convex lens. In a young person, the lens is composed of a strong elastic capsule filled with viscous, proteinaceous, but transparent fluid. When the lens is in a relaxed state, with Ciliary muscle no tension on its capsule, it assumes an almost spherical shape, owing mainly to the elastic retraction of the lens Suspensory capsule. However, as shown in Figure 50-10, about 70 Lens ligaments suspensory ligaments attach radially around the lens, pull- ing the lens edges toward the outer circle of the eyeball. These ligaments are constantly tensed by their attach- ments at the anterior border of the choroid and retina. The tension on the ligaments causes the lens to remain Figure 50-10. Mechanism of accommodation (focusing). relatively flat under normal eye conditions. Also located at the lateral attachments of the lens liga- ments to the eyeball is the ciliary muscle, which has two to keep the object constantly in focus. Sympathetic stimu- separate sets of smooth muscle fibers—meridional fibers lation has an additional effect in relaxing the ciliary muscle, and circular fibers. The meridional fibers extend from the but this effect is so weak that it plays almost no role in the peripheral ends of the suspensory ligaments to the cor- normal accommodation mechanism; the neurophysiology neoscleral junction. When these muscle fibers contract, of this mechanism is discussed in Chapter 52. the peripheral insertions of the lens ligaments are pulled Presbyopia—Loss of Accommodation by the Lens. As medially toward the edges of the cornea, thereby releas- a person grows older, the lens grows larger and thicker ing the ligaments’ tension on the lens. The circular fibers and becomes far less elastic, partly because of progressive are arranged circularly all the way around the ligament denaturation of the lens proteins. The ability of the lens attachments so that when they contract, a sphincter-like to change shape decreases with age. The power of accom- action occurs, decreasing the diameter of the circle of lig- modation decreases from about 14 diopters in a child to ament attachments; this action also allows the ligaments less than 2 diopters by the time a person reaches 45 to 50 to pull less on the lens capsule. years and to essentially 0 diopters at age 70 years. There- Thus, contraction of either set of smooth muscle fibers after, the lens remains almost totally nonaccommodating, in the ciliary muscle relaxes the ligaments to the lens cap- a condition known as presbyopia. sule, and the lens assumes a more spherical shape, like Once a person has reached the state of presbyopia, that of a balloon, because of the natural elasticity of the each eye remains focused permanently at an almost con- lens capsule. stant distance; this distance depends on the physical char- Accommodation Is Controlled by Parasympathetic acteristics of each person’s eyes. The eyes can no longer Nerves. Ciliary muscle is controlled almost entirely by par- accommodate for both near and far vision. To see clearly asympathetic nerve signals transmitted to the eye through both in the distance and nearby, an older person must the third cranial nerve from the third nerve nucleus in the wear bifocal glasses, with the upper segment focused for brain stem, as explained in Chapter 52. Stimulation of par- far-seeing and the lower segment focused for near-seeing asympathetic nerves contracts both sets of ciliary muscle (e.g., for reading). fibers, which relaxes the lens ligaments, thus allowing the PUPILLARY DIAMETER lens to become thicker and increase its refractive power. With this increased refractive power, the eye focuses on The major function of the iris is to increase the amount of objects nearer than when the eye has less refractive power. light that enters the eye during darkness and to decrease Consequently, as a distant object moves toward the eye, the amount of light that enters the eye in daylight. The the number of parasympathetic impulses impinging on the reflexes for controlling this mechanism are considered in ciliary muscle must be progressively increased for the eye Chapter 52. 631 UNIT X The Nervous System: B. The Special Senses The amount of light that enters the eye through the pupil is proportional to the area of the pupil or to the square of the diameter of the pupil. The pupil of the human eye can become as small as about 1.5 millimeters Point sources of light and as large as 8 millimeters in diameter. The quantity of light entering the eye can change about 30-fold as a result of changes in pupillary aperture. Lens Focal point “Depth of Focus” of the Lens System Increases With Lens Decreasing Pupillary Diameter. Figure 50-11 shows two eyes that are exactly alike except for the diameters of the pupillary apertures. In the upper eye, the pupillary aper- Point sources of light ture is small and, in the lower eye, the aperture is large. In front of each of these two eyes are two small point sources of light; light from each passes through the pupillary aper- Figure 50-11. Effect of small (top) and large (bottom) pupillary aper- ture and focuses on the retina. Consequently, in both eyes, tures on depth of focus. the retina sees two spots of light in perfect focus. If the retina is moved forward or backward to an out of focus po- sition (dashed lines), the size of each spot will not change much in the upper eye, but in the lower eye the size of each spot will increase greatly, becoming a “blur circle.” In other words, the upper lens system has far greater depth of focus than the bottom lens system. When a lens system has Emmetropia great depth of focus, the retina can be displaced consider- ably from the focal plane, or the lens strength can change considerably from normal, and the image will still remain nearly in sharp focus, whereas when a lens system has a “shallow” depth of focus, moving the retina only slightly away from the focal plane causes extreme blurring. The greatest possible depth of focus occurs when the pupil is extremely small. The reason for this is that with a Hyperopia very small aperture, almost all the rays pass through the center of the lens, and the central most rays are always in focus, as explained earlier. Errors of Refraction See Video 50-1. Myopia Emmetropia (Normal Vision). As shown in Figure 50-12, the eye is considered to be normal, or emmetropic, if parallel Figure 50-12. Parallel light rays focus on the retina in emmetropia, light rays from distant objects are in sharp focus on the retina behind the retina in hyperopia, and in front of the retina in myopia. when the ciliary muscle is completely relaxed. This means that the emmetropic eye can see all distant objects clearly closer and closer to the eye can also be focused sharply un- with its ciliary muscle relaxed. However, to focus objects til the ciliary muscle has contracted to its limit. In old age, at close range, the eye must contract its ciliary muscle and when the lens becomes “presbyopic,” a farsighted person is thereby provide an appropriate degree of accommodation. often unable to accommodate the lens sufficiently to focus Hyperopia (Farsightedness). Hyperopia, also known as even on distant objects, much less on near objects. “farsightedness,” is usually due to either an eyeball that is too Myopia (Nearsightedness). In myopia, or “nearsight- short or, occasionally, to a lens system that is too weak. In edness,” when the ciliary muscle is completely relaxed, the this condition, as seen in the middle panel of Figure 50-12, light rays coming from distant objects are focused in front parallel light rays are not bent sufficiently by the relaxed of the retina, as shown in the bottom panel of Figure 50-12. lens system to come to focus by the time they reach the This condition is usually due to too long an eyeball but also retina. To overcome this abnormality, the ciliary muscle can result from too much refractive power in the lens sys- must contract to increase the strength of the lens. By us- tem of the eye. ing the mechanism of accommodation, a farsighted person No mechanism exists whereby the eye can decrease the is capable of focusing distant objects on the retina. If the strength of its lens to less than that which exists when the person has used only a small amount of strength in the cili- ciliary muscle is completely relaxed. A myopic person has ary muscle to accommodate for the distant objects, he or no mechanism to focus distant objects sharply on the reti- she still has much accommodative power left, and objects na. However, as an object moves nearer to the person’s eye, 632 CHAPTER 50 The Eye: I. Optics of Vision it finally gets close enough that its image can be focused. Then, when the object comes still closer to the eye, the per- son can use the mechanism of accommodation to keep the image focused clearly. A myopic person has a definite limit- ing “far point” for clear vision. Correction of Myopia and Hyperopia Through Use of UNIT X Lenses. If the refractive surfaces of the eye have too much refractive power, as in myopia, this excessive refractive power can be neutralized by placing a concave spherical lens in front of the eye, which will diverge rays. Such cor- rection is shown in the upper diagram of Figure 50-13. Conversely, in a person who has hyperopia—that is, someone who has too weak a lens system—the abnormal vision can be corrected by adding refractive power using a convex lens in front of the eye. This correction is demon- strated in the lower diagram of Figure 50-13. One usually determines the strength of the concave or Figure 50-13. Correction of myopia with a concave lens (top) and convex lens needed for clear vision by “trial and error”— correction of hyperopia with a convex lens (bottom). that is, by first trying a strong lens and then a stronger or weaker lens until the one that gives the best visual acuity Point source is found. of light Astigmatism. Astigmatism is a refractive error of the eye that causes the visual image in one plane to focus at A B a different distance from that of the plane at right angles. Astigmatism usually results from too great a curvature of the cornea in one plane of the eye. An example of an astig- C matic lens would be a lens surface like that of an egg lying D sidewise to the incoming light. The degree of curvature in Focal line Plane AC the plane through the long axis of the egg is not nearly as for plane BD Plane BD (less refractive great as the degree of curvature in the plane through the (more refractive power) short axis. power) Focal line Because the curvature of the astigmatic lens along one for plane AC plane is less than the curvature along the other plane, light rays striking the peripheral portions of the lens in one plane are not bent nearly as much as the rays striking the periph- Figure 50-14. Astigmatism, demonstrating that light rays focus at eral portions of the other plane. This effect is demonstrated one focal distance in one focal plane (plane AC) and at another focal in Figure 50-14, which shows rays of light originating from distance in the plane at a right angle (plane BD). a point source and passing through an oblong, astigmatic lens. The light rays in the vertical plane, indicated by plane the astigmatic lens. Then, an additional cylindrical lens is BD, are refracted greatly by the astigmatic lens because of used to correct the remaining error in the remaining plane. the greater curvature in the vertical direction than in the To do this, both the axis and the strength of the required horizontal direction. By contrast, the light rays in the hori- cylindrical lens must be determined. zontal plane, indicated by plane AC, are not bent nearly as Several methods exist for determining the axis of the much as the light rays in vertical plane BD. Therefore, light abnormal cylindrical component of the lens system of an rays passing through an astigmatic lens do not all come to a eye. One of these methods is based on the use of paral- common focal point because the light rays passing through lel black bars of the type shown in Figure 50-15. Some of one plane focus far in front of those passing through the these parallel bars are vertical, some are horizontal, and other plane. some are at various angles to the vertical and horizontal The accommodative power of the eye can never com- axes. After placing various spherical lenses in front of the pensate for astigmatism because, during accommodation, astigmatic eye, a strength of lens that causes sharp focus of the curvature of the eye lens changes approximately equally one set of parallel bars but does not correct the fuzziness in both planes; therefore, in astigmatism, each of the two of the set of bars at right angles to the sharp bars is usually planes requires a different degree of accommodation. Thus, found. It can be shown from the physical principles of op- without the aid of glasses, a person with astigmatism never tics discussed earlier in this chapter that the axis of the out sees in sharp focus. of focus cylindrical component of the optical system is par- Correction of Astigmatism With a Cylindrical Lens. One allel to the bars that are fuzzy. Once this axis is found, the may consider an astigmatic eye as having a lens system examiner tries progressively stronger and weaker positive made up of two cylindrical lenses of different strengths and or negative cylindrical lenses, the axes of which are placed placed at right angles to each other. To correct for astigma- in line with the out of focus bars, until the patient sees all tism, the usual procedure is to find a spherical lens by trial the crossed bars with equal clarity. When this goal has been and error that corrects the focus in one of the two planes of accomplished, the examiner directs the optician to grind a 633 UNIT X The Nervous System: B. The Special Senses special lens combining both the spherical correction and When a cataract has obscured light transmission so the cylindrical correction at the appropriate axis. greatly that it seriously impairs vision, the condition can be Correction of Optical Abnormalities With Contact corrected by surgical removal of the lens. When the lens is Lenses. Glass or plastic contact lenses that fit snugly against removed, the eye loses a large portion of its refractive pow- the anterior surface of the cornea can be inserted. These lenses er, which must be replaced by placing a powerful convex are held in place by a thin layer of tear fluid that fills the space lens in front of the eye; usually, however, an artificial plastic between the contact lens and the anterior eye surface. lens is implanted in the eye in place of the removed lens. A special feature of the contact lens is that it nullifies the re- fraction that normally occurs at the anterior surface of the cor- VISUAL ACUITY nea almost entirely. The reason for this nullification is that the Theoretically, light from a distant point source, when tears between the contact lens and the cornea have a refractive index almost equal to that of the cornea, so the anterior surface focused on the retina, should be infinitely small. However, of the cornea no longer plays a significant role in the eye’s opti- because the lens system of the eye is never perfect, such cal system. Instead, the outer surface of the contact lens plays a retinal spot ordinarily has a total diameter of about 11 the major role. Thus, the refraction of this surface of the con- micrometers, even with maximal resolution of the normal tact lens substitutes for the cornea’s usual refraction. This factor eye optical system. The spot is brightest in its center and is especially important in people whose eye refractive errors shades off gradually toward the edges, as shown by the are caused by an abnormally shaped cornea, such as those who two-point images in Figure 50-16. have an odd-shaped, bulging cornea, a condition called kera- The average diameter of the cones in the fovea of the ret- toconus. Without the contact lens, the bulging cornea causes ina—the central part of the retina, where vision is most highly such severe abnormality of vision that almost no glasses can developed—is about 1.5 micrometers, which is one-seventh correct the vision satisfactorily; when a contact lens is used, the diameter of the spot of light. Nevertheless, because the however, the corneal refraction is neutralized, and normal re- fraction by the outer surface of the contact lens is substituted. spot of light has a bright center point and shaded edges, a The contact lens has several other advantages as well, person can normally distinguish two separate points if their including the following: (1) the lens turns with the eye and centers lie up to 2 micrometers apart on the retina, which is gives a broader field of clear vision than glasses; and (2) the slightly greater than the width of a foveal cone. This discrimi- contact lens has little effect on the size of the object the nation between points is also shown in Figure 50-16. person sees through the lens, whereas lenses placed about 1 The normal visual acuity of the human eye for discrim- centimeter in front of the eye do affect the size of the image inating between point sources of light is about 25 seconds in addition to correcting the focus. of arc. That is, when light rays from two separate points Cataracts—Opaque Areas in the Lens. “Cataracts” are strike the eye with an angle of at least 25 seconds between an especially common eye abnormality that occurs mainly them, they can usually be recognized as two points instead in older people. A cataract is a cloudy or opaque area (or of one. This means that a person with normal visual acu- areas) in the lens. In the early stage of cataract formation, ity looking at two bright pinpoint spots of light 10 meters the proteins in some of the lens fibers become denatured. Later, these same proteins coagulate to form opaque areas away can barely distinguish the spots as separate entities in place of the normal transparent protein fibers. when they are 1.5 to 2 millimeters apart. The fovea is less than 0.5 millimeter (