Rock Strength and Deformability Chapter 3 PDF
Document Details
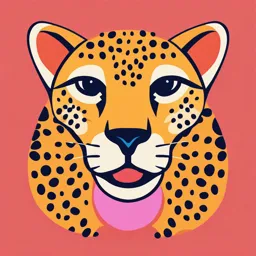
Uploaded by NoteworthyCesium
Palawan State University
2024
Engr. Keren Joy P. Orillosa, Magsipoc, Pinky Joy O., Sabillo, Krizelle T.
Tags
Summary
This is a chapter on rock strength and deformability from a university course. It discusses topics including rock strength, deformation, and features causing rock anisotropy. The chapter is from Palawan State University, Philippines.
Full Transcript
CE PC1 ROCK MECHANICS ROCK STRENGTH AND DEFORMABILITY CHAPTER 3 Instructor ENGR. KEREN JOY P. ORILLOSA Prepared By MAGSIPOC, PINKY JOY O. SABILLO, KRIZELLE T. JULY 2024 Republic of the Philippines Palawan State Universit...
CE PC1 ROCK MECHANICS ROCK STRENGTH AND DEFORMABILITY CHAPTER 3 Instructor ENGR. KEREN JOY P. ORILLOSA Prepared By MAGSIPOC, PINKY JOY O. SABILLO, KRIZELLE T. JULY 2024 Republic of the Philippines Palawan State University College of Engineering, Architecture and Technology Department of Civil Engineering CE PC 1: ROCK MECHANICS (GEOTECHNICAL ENGINEERING II) ROCK STRENGTH AND DEFORMABILITY CHAPTER THREE Prepared by: Magsipoc, Pinky Joy O. Sabillo, Krizelle T. Group 6 Submitted to: Engr. Keren Joy P. Orillosa Professional Course 1 Instructor July 3, 2024 CHAPTER 3: ROCK STRENGTH AND DEFORMABILITY TABLE OF CONTENTS Objectives ----------------------------------------------------------------------------------------------------- 2 Discussion Lesson 1: Concept and Definitions --------------------------------------------------------------------- 3 Rock Strength ------------------------------------------------------------------------------------------ 3 Rock Deformation ------------------------------------------------------------------------------- 3 Additional Terms ------------------------------------------------------------------------------- 5 Lesson 2: Isotropic Rock Behavior --------------------------------------------------------------------- 6 Isotropy ------------------------------------------------------------------------------------------ 6 Isotropic Rock Behavior on Uniaxial Compression ------------------------------------- 6 Isotropic Rock Behavior on Multiaxial Compression ------------------------------------- 7 Strength Criteria -------------------------------------------------------------------------------- 7 Lesson 3: Anisotropic Rock Behavior --------------------------------------------------------------------- 9 Anisotropy ------------------------------------------------------------------------------------------ 9 Features Causing Rock Anisotropy ----------------------------------------------------------- 9 Classification of Rock Anisotropy ----------------------------------------------------------- 10 Types of Anisotropic Rock Failure Criteria ------------------------------------------------- 11 Lesson 4: Behavior of Discontinuous Rock ----------------------------------------------------------- 12 Discontinuity ------------------------------------------------------------------------------------------ 12 Effect of Discontinuities -------------------------------------------------------------------------------- 13 References --------------------------------------------------------------------------------------- 14 1 CHAPTER 3: ROCK STRENGTH AND DEFORMABILITY OBJECTIVES At the end of this module, you should be able to: Define fundamental terms and concepts in rock mechanics and the importance of understanding these concepts in the context of engineering and geological applications. Differentiate between isotropic and anisotropic behaviors in rocks. Determine rock properties with different direction and the implications for engineering design and stability assessments. Identify common discontinuities in rock masses and their influence on rock behavior. 2 CHAPTER 3: ROCK STRENGTH AND DEFORMABILITY LESSON 1: CONCEPTS AND DEFINITIONS 1.1. Rock Strength Rock Strength is ability of rocks to withstand an applied stress without complete failure. The most common strengths of rock are: compressive strength- the capacity of a material or structure to withstand loads tending to reduce size tensile strength- the maximum stress that a material can bear before breaking when it is allowed to be stretched or pulled shear strength- the ability to resist forces that cause the material's internal structure to slide against itself. The principal factors controlling the strength of rocks are: 1. mineral composition, structure and texture; 2. bedding, jointing and anisotropy; 3. water content; 4. state of stress in the rock mass. 1.2. Rock Deformation Rock deformation is the process by which rocks change shape or size in response to stress. Stress is a force applied per unit area, and it can be caused by a variety of factors, including plate tectonics, gravity, and human activity. Types of Stress: Tensional stress Compressional stress Shear stress Rocks can deform in two main ways: brittlely or ductilety. 1. Brittle deformation - Means the rock cannot accommodate a force by flowing but will instead “snap,” or break. - Most common in the upper crust of the Earth, where the temperature and pressure conditions are relatively low. - Brittle deformation can produce a variety of features, including: ✓ Faults: Faults are fractures in rocks along which there has been displacement. Faults can be caused by a variety of stresses, including compression, tension, and shear. 3 CHAPTER 3: ROCK STRENGTH AND DEFORMABILITY ✓ Joints: Joints are fractures in rocks that do not show any displacement. Joints are often caused by cooling or drying of rocks. ✓ Breccia: Breccia is a type of rock that is made up of angular fragments of other rocks. Breccia is often formed by brittle deformation of rocks. 2. Ductile deformation - Means the rock accommodates a force by “flowing” instead of breaking. - Most common in the lower crust and mantle of the Earth, where the temperature and pressure conditions are relatively high. - Ductile deformation can produce a variety of features, including: ✓ Folds: Folds are bends in rocks. Folds can be caused by a variety of stresses, including compression and tension. Types of folds: Symmetrical fold Assymetrical fold Overfold Recumbent fold ✓ Foliation: Foliation is a layering that can be seen in some metamorphic rocks. Foliation is caused by the alignment of minerals in the rock during ductile deformation. ✓ Lineation: Lineation is a linear feature that can be seen in some metamorphic rocks. Lineation is caused by the alignment of minerals or elongated grains in the rock during ductile deformation. Factors affecting the type of deformation: 1. Rock Type - the type of rock will influence the way it deforms in response to forces acting on it. Generally speaking, if the rock is made up primarily of minerals that are hard (6 or above on Moh’s scale) and non-platy, then it will tend to deform in a brittle manner. If the minerals are primarily soft or platy, then deformation will tend to be ductile. 2. Temperature- At higher temperatures, rock is able to stretch more when stress is applied. Since rock is more malleable at high temperatures, it forms more ductile structures. At lower temperatures closer to the surface of the Earth, rock is more likely to fracture or break when stressed. 3. Deformation Rate- the rate at which force is applied will also influence whether the rock will undergo brittle or ductile deformation. The faster force is applied, the greater the likelihood of the rock breaking in a brittle fashion, while a slow application of force can allow the rock to deform in a ductile manner. For example, wood is brittle at room temperature and tends to break if a large force is applied quickly. However, wood can be bent into curved shapes for construction purposes through a process that requires slow application of force to encourage bending and prevent breaking. Stages of deformation: Elastic Deformation - wherein the strain is reversible. Ductile Deformation -wherein the strain is irreversible. 4 CHAPTER 3: ROCK STRENGTH AND DEFORMABILITY Fracture - irreversible strain wherein the material breaks. 1.3. Additional Terms Peak strength is the maximum stress, usually averaged over a plane, that the rock can sustain under a given set of conditions. After its peak strength has been exceeded, the specimen may still have some load-carrying capacity or strength. residual strength is the minimum stress, reached generally only after considerable post-peak deformation. stress that a damaged rock can still carry Fracture is the formation of planes of separation in the rock material. It involves the breaking of bonds to form new surfaces. Brittle fracture is the process by which sudden loss of strength occurs across a plane following little or no permanent (plastic) deformation. It is usually associated with strain-softening or strain- weakening behaviour of the specimen. Yield occurs when there is a departure from elastic behaviour, i.e. when some of the deformation becomes irrecoverable. The point where a permanent deformation begins. The yield stress is the stress at which permanent deformation first appears. Failure is a state/point where a rock no longer adequately supports the load Effective stress is defined, in general terms, as the stress which governs the gross mechanical response of a porous material. The effective stress is a function of the total or applied stress and the pressure of the fluid in the pores of the material, known as the pore pressure or pore-water pressure. The concept of effective stress was first developed by Karl Terzaghi who used it to provide a rational basis for the understanding of the engineering behaviour of soils. 5 CHAPTER 3: ROCK STRENGTH AND DEFORMABILITY LESSON 2: Isotropic Rock Behavior 2.1 Isotropic Isotropy means uniform in all directions and comes from the Greek words isos (equal) and tropos (way). Isotropic materials are materials whose properties remain the same when tested in different directions. A good example of an isotropic material is something with a crystalline structure. Isotropic can mean other things in different fields of science or contexts. However, the root meaning of “the same in every direction” is consistent. 2.2 Isotropic Rock Behavior on Uniaxial Compression Uniaxial Compression of cylindrical specimens prepared from drill core, is probably the most widely performed test on rock. It is used to determine the uniaxial or unconfined compressive strength, the elastic constants, Young’s modulus and Poisson’s ratio of the rock material. Axial Young’s Modulus of the specimen varies throughout the loading history and so is not a uniquely determined constant for the material. It may be calculated in a number of ways, the most common being: (a) Tangent Young’s modulus, Et, is the slope of the axial stress–axial strain curve at some fixed percentage, generally 50%, of the peak strength. (b) Average Young’s modulus, Eav, is the average slope of the more-or-less straight-line portion of the axial stress–strain curve. (c) Secant Young’s modulus, Es, is the slope of a straight line joining the origin of the axial stress–strain curve to a point on the curve at some fixed percentage of the peak strength. Suggested techniques for determining the uniaxial compressive strength and deformability of rock material are given by the International Society for Rock Mechanics Commission on Standardization of Laboratory and Field Tests (ISRM Commission, 1979). The essential features of the recommended procedure are: (a) The test specimens should be right circular cylinders having a height to diameter ratio of 2.5–3.0 and a diameter preferably of not less than NX core size, approximately 54 mm. The specimen diameter should be at least 10 times the size of the largest grain in the rock. (b) The ends of the specimen should be flat to within 0.02 mm and should not depart from perpendicularity to the axis of the specimen by more than 0.001 rad or 0.05 mm in 50 mm. (c) The use of capping materials or end surface treatments other than machining is not permitted. 6 CHAPTER 3: ROCK STRENGTH AND DEFORMABILITY (d) Specimens should be stored, for no longer than 30 days, in such a way as to preserve the natural water content, as far as possible, and tested in that condition. (e) Load should be applied to the specimen at a constant stress rate of 0.5–1.0 MPa s^−1. (f) Axial load and axial and radial or circumferential strains or deformations should be recorded throughout each test. (g) There should be at least five replications of each test. 2.3 Isotropic Rock Behavior on Multiaxial Compression A basic principle of the laboratory testing of rock to obtain data for use in design analyses, is that the boundary conditions applied to the test specimen should simulate those imposed on the rock element in situ. This can rarely be achieved. General practice is to study the behavior of the rock under known uniform applied stress systems. Types of Multiaxial Compression Test (a) Biaxial compression tests are carried out by applying different normal stresses to two pairs of faces of a cube, plate or rectangular prism of rock. (b) Triaxial compression tests is carried out on cylindrical specimens prepared in the same manner as those used for uniaxial compression tests. (c) Polyaxial compression tests may be carried out on cubes or rectangular prisms of rock with different normal stresses being applied to each pair of opposite faces. Figure 2.2 Types of Multiaxial Compression Test 2.4 Strength Criteria The strength criterion is a basic mechanical theory. The failure modes of materials are relevant not only to stress conditions but also to material properties, and the mechanical basis and verification standards of strength criteria are discussed. Types of Strength Criterion (a) Peak Strength Criterion is a relation between stress components which will permit the peak strengths developed under various stress combinations to be predicted. 7 CHAPTER 3: ROCK STRENGTH AND DEFORMABILITY (b) Residual Strength Criterion may be used to predict residual strengths under varying stress conditions. (c) Yield Criterion is a relation between stress components which is satisfied at the onset of permanent deformation. Strength Criteria for Isotropic Rock Material (a) Coulomb’s Shear Strength Criterion Coulomb (1776) postulated that the shear strengths of rock and of soil are made up of two parts – a constant cohesion and a normal stress-dependent frictional component. Although it is widely used, Coulomb’s criterion is not a particularly satisfactory peak strength criterion for rock material. The reasons for this are: It implies that a major shear fracture exists at peak strength. Observations such as those made by Wawersik and Fairhurst (1970) show that this is not always the case. It implies a direction of shear failure which does not always agree with experimental observations. Experimental peak strength envelopes are generally non-linear. They can be considered linear only over limited ranges of 𝜎𝑛 or 𝜎3. For these reasons, other peak strength criteria are preferred for intact rock. However, the Coulomb criterion can provide a good representation of residual strength conditions, and more particularly, of the shear strengths of discontinuities in rock. (b) Griffith Crack Theory Griffith (1921) postulated that fracture of brittle materials, such as steel and glass, is initiated at tensile stress concentrations at the tips of minute, thin cracks (now referred to as Griffith cracks) distributed throughout an otherwise isotropic, elastic material. Griffith based his determination of the conditions under which a crack would extend on his energy instability concept where a crack will extend only when the total potential energy of the system of applied forces and material decreases or remains constant with an increase in crack length. Figure 2.2 Three Basic Modes of Distortion at a Crack Tip 8 CHAPTER 3: ROCK STRENGTH AND DEFORMABILITY LESSON 3: ANISOTROPIC ROCK BEHAVIOR 3.1. Anisotropy Anisotropy is a property of rock that results in the rock's strength (or other material properties) being directionally dependent. Unlike isotropic rocks, which have the same strength properties in all loading directions, anisotropic rocks can have significantly lower strength when loaded along their weakest orientation. 3.2. Features Causing Rock Anisotropy Rocks are defined as a mechanical bonding of the grains of one mineral (mono mineral rock) or more than one mineral (Poly-mineral rock), this mechanical bonding depends on the origin of the rock, whether it is igneous, metamorphic or sedimentary rock. During the formation of different types of rocks there are different processes taking place which are categorized into both primary and secondary structures. A. Primary structures Micro geological features are named also the primary structures which are found during the formation stage of different rocks. These features influence the rock anisotropy by: rock fabric anisotropy, texture, schistosity and feasibility. They are mainly found in the microscopic scale and also are related to the grain size. In general, the anisotropic behavior of the rocks mainly depending on the textures and fabric of the principal rock-forming minerals (the microscopic fabric) (Ullemeyer et al. 2006). According to (Bagheripour et al. 2011), the anisotropic nature of rocks is found at: Most foliated metamorphic rocks, such as schist, slates, gneisses and phyllites, contain a natural orientation in their flat/long minerals or a banding phenomenon which results in anisotropy in their mechanical properties. Stratified sedimentary rocks like sandstone, shale or sandstone - shale alteration often display anisotropic behaviors due to presence of bedding planes. The major reason for the anisotropy in sedimentary rocks is related to the sedimentation processes of the different layers (strata) or different minerals with various grain sizes. Igneous rocks having flow structures as may be observed in porous rhyolites due to weathering (Matsukura, Hashizume, and Oguchi 2002). Generally, igneous rocks have few possibilities of fabric anisotropy. But in some cases, the anisotropy may be found due to layering when the lava flows and moves as highly viscous masses immediately before the consolidation (such as granite) (Walhlstrom 1973). B. Secondary structures Secondary structures are known also as the macro-scale features of rocks which are defined by one word as “discontinuities”. They are defined as: (i) cracks and fractures, (ii) bedding planes and (iii) shear planes and faults (Salager et al. 2013). Such features’ influence is significant and associated with three distinct issues (Bobet et al. 2009): The scale: which effects the modelling of these planes implicitly or explicitly, stress and/or displacement conducted: these planes significantly reduce the rock strength, and 9 CHAPTER 3: ROCK STRENGTH AND DEFORMABILITY relative motions of rock blocks: the discontinuity limits the elastic behavior of the rock material. 3.3. Classification of Rock Anisotropy Anisotropy of rocks can be quantified and classified (degree of anisotropy). There are several ways to classify rocks. Two often used systems are: (i) The Point Load Index (ii) The Strength Anisotropy Classification 1. The Point Load Index Tsidzi (1990) has proposed a classification for foliated rocks to classify the degree of foliation as well as the degree of anisotropy. A fabric index (Tsidzi, 1986) was introduced first to classify metamorphic rocks. Then, it has been noted that there is a strong relation between the degree of the foliation and the point load strength anisotropy index according to Eq. (2) which is proposed by the ISRM (1985) 2. Strength Anisotropy Classification (Rc). Ramamurthy (1993) defined the anisotropy strength (Rc), eq. (3) quantifies the Rc value as the ratio between strength of the intact rock of orientation angle (90°) and the minimum strength of the same intact rock with orientation angle (0°) 10 CHAPTER 3: ROCK STRENGTH AND DEFORMABILITY 3.4. Types of Anisotropic Rock Failure Criteria Various studies and analyses have been donated to investigate the rock anisotropy and specially the strength anisotropy. Out of these researches, many failure criteria have been introduced which tried to predict the behavior of the anisotropic rocks under loading and therefore the rock strength. Duveau et al. (1998) have performed a classification of most of the introduced anisotropic rock failure criteria till 1998 and Ambrose (2014) has added the criteria which are developed till 2014. The classification of the failure criteria was mainly to both continuous and discontinuous criteria. For the continuous criteria, the rock material is considered as a continuous body with the assumptions of a continuous variation of strength. The continuous criteria are divided into both continuous criteria using mathematical approach and continuous criteria using empirical approach Mathematical Continuous Criteria A criterion at which the strength function is generally described by a mathematical technique, which considers the type of symmetries existing in the material. Out of these criteria, number of constants are generated. Empirical Continuous Criteria They are an extension from isotropic failure criterion to describe the anisotropic strength by empirical laws that define the variation of material parameters with respect to loading orientation. The various parameters are determined from fitting experimental data. 11 CHAPTER 3: ROCK STRENGTH AND DEFORMABILITY LESSON 4: Behavior of Discontinuous Rock 4.1 Discontinuity Discontinuity is a collective term used for all structural breaks in geologic materials which is usually are unhealed and have zero or low tensile strength. Classification of Discontinuities (a) Fracture is a term used to describe any natural break in geologic material excluding shears and shear zones. (b) Shear is a structural break where differential movement has taken place along a surface or zone of failure by shear. (c) Fault is a shear with significant continuity which can be correlated between observations. (d) Shear/Fault Zone is a shear that is expressed in relative terms of width. (e) Shear/Fault Disturbed Zone an associated zone of fractures and/or folds adjacent to a shear or shear zone where the country rock has been subjected to only minor catacaustic action and maybe mineralized. Properties of Discontinuities (a) Fracture frequency is defined as the number of natural fractures occurring within a base length or core run. The number of fractures is divided by the length and is reported as number of fractures per foot or fractures per meter. Fracture frequency is expressed as three fractures per meter or six fractures per foot. (b) Fracture density is based on the spacing of all natural fractures in an exposure or core recovery lengths in boreholes. (c) Fracture spacing corresponds to the shortest distance between two consecutive fractures. Care should be taken to measure the true spacing instead of the apparent spacing. (d) Fracture continuity where discontinuities rarely cross entire rock masses and often terminates or branch and form bridges of intact rock. (e) Fracture openness, infilling and healing where openness of a fracture and the type and amount of infilling material have an effect on its hydraulic behavior and shear strength properties. Clean and tight fractures are usually less pervious and have higher shear strengths than open and filled discontinuities. It is also important to distinguish between the major types of fracture infilling. (f) Fracture moisture conditions are flow in a rock mass which is essentially along discontinuities. Different descriptors can be used to describe the amount of water along discontinuities varying from dry conditions to continuous flow (in which case the amount of water must be estimated). (g) Fracture roughness as for fracture openness and infilling, fracture roughness controls the shear strength of rock discontinuities. Rough discontinuities have higher shear strength than smooth ones. (h) Fracture surface hardness can be determined by comparison between hardness measurements on different parts of a fracture surface can give an indication of the degree of surface weathering. 12 CHAPTER 3: ROCK STRENGTH AND DEFORMABILITY (i) Discontinuities orientation is usually planar and their orientation can be defined by two angles (i) strike and dip angles, or (ii) dip direction and dip angles. Strike - the compass direction of a line formed by the intersection of a horizontal plane and an inclined geologic plane such as a fault, fracture, joint, etc. because it is a compass direction, the strike is usually expressed relative to North or South. Hence, strike is expressed as "North (or South) so many degrees East" or "North (or South) so many degrees West" Dip - the angle between a horizontal plane and the plane of interest. A thin stream of water poured on an inclined surface always runs down parallel to dip. The inclination of the water line down from the horizontal plane is called the (true) dip angle. The true dip angle is always measured perpendicular to the strike line. Dip Direction - The angle between North and the direction that the water runs down an inclined geologic plane. It is measured clockwise and varies between 0 and 360 degrees. Apparent Dip - The inclination angle of a line on an inclined geologic plane measured in a direction oblique to the strike direction. It varies between the true dip and 0 degree. The orientation of a plane is shown on maps using a T-shaped symbol; the long line of the symbol Indicates the strike direction, and the short line shows the dip direction. (j) Strength and Deformability of particular interest when modeling the mechanical response of rock masses is the shear strength of rock discontinuities, and their deformability in the shear and normal directions. 4.2 Effect of Discontinuities Rock masses are far from being continua and consist essentially of two constituents. Intact rock and discontinuities (planes of weakness). The existence of one or several sets of discontinuities in a rock mass creates anisotropy in its response to loading and unloading, Also, compared to intact rock, jointed rock shows a higher permeability, reduced shear strength along the planes of discontinuity and increased deformability and negligible tensile strength in directions normal to those planes. Furthermore, discontinuities create scale effects. Finally, discontinuities form blocks by intersection that can result in stability problems during surface or underground excavations. Three approaches can be followed to account for the effect of discontinuities on rock mass strength and deformability : The first approach consists of empirically reducing the deformability and strength properties of rock masses from those measured on intact rock samples in the laboratory. A second approach consists of treating joints as discrete features. The third approach is to treat jointed rock as an equivalent anisotropic continuum with deformability and strength properties that are directional and also reflect the properties of intact rock and those of the joint sets, i.e. orientation, spacing and normal and shear stiffnesses. 13 CHAPTER 3: ROCK STRENGTH AND DEFORMABILITY References: ✓ Brady BHG, Brown ET. 2004. Rock mechanics for underground mining. 3rd ed. AZ. Dordrecht, The Netherlands: Kluwer Academic Publishers. ✓ M. Sc. Mohamed Ismael. Behaviour of anisotropic rocks. M. Sc. Lifu Chang & Prof. Dr.-Ing. habil. Heinz Konietzky (Geotechnical Institute, TU Bergakademie Freiberg) ✓ Discontinuities. (n.d.). B. Amadei. Retrieved July 3, 2024, from https://www.colorado.edu/faculty/amadei/sites/default/files/attachedfiles/discontinuities.p df 14