ILM: Clinical Genetics Basics PDF (July 2024)
Document Details
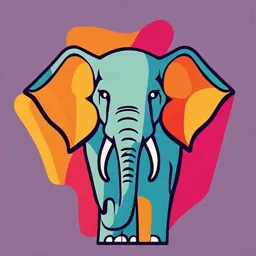
Uploaded by GratefulHyperbolic
Amerongen
2024
James Proffitt, PhD
Tags
Summary
This document is a handout with learning objectives for a clinical genetics course, covering basic concepts of inheritance. It includes information about session details, instructional methods, and readings for students. No questions are listed or identifiable in this sample.
Full Transcript
ILM: CLINICAL GENETICS BASICS Block: Foundations Block Director: James Proffitt, PhD Session Date: Monday, July 29, 2024 Time: 4:00 – 4:30 pm Instructor: Jordana Smith Department: Ophthalmology & Vision Science Email: Plea...
ILM: CLINICAL GENETICS BASICS Block: Foundations Block Director: James Proffitt, PhD Session Date: Monday, July 29, 2024 Time: 4:00 – 4:30 pm Instructor: Jordana Smith Department: Ophthalmology & Vision Science Email: Please contact Dr. Proffitt with questions – [email protected] INSTRUCTIONAL METHODS Primary Method: IM10: Independent Learning Flipped Correlation Resource Types: RE18: Written or Visual Media (or Digital Equivalent) INSTRUCTIONS Read the notes LO #1 is a ppt slide deck of definitions that you can use/transcribe onto Anki to test yourself on the various terms Watch the video linked online Optional worksheet you can use to practice some questions on inherited disorders, risk calculations – you can continue to fill out this worksheet as you encounter many of these diseases during the block READINGS Notes Optional additional reading – published paper linked to the session on MedLearn on “Spectrum of Recessiveness” – this paper introduces some of the complexities in clinical genetics beyond the “pea experiments” and shows that recessive carriers can certainly have symptoms and this can be quite variable depending on the involved gene, mutation, and final phenotype LEARNING OBJECTIVES 1. Define the following terms and identify examples of each: locus, genotype, phenotype, allele, homozygote, heterozygote, hemizygote, genetic heterogeneity (allelic and locus), variable expression, pleiotropy, reduced penetrance, consanguinity, Single Nucleotide Polymorphism (SNP), copy number variant (CNV), Variant of Uncertain Significance (VUS/VOUS). 2. Using information from a medical history and pedigree, assign a disorder to an autosomal dominant, autosomal recessive, X-linked dominant and X-linked recessive inheritance pattern. Learn how this determination can help to differentiate clinical presentations. 3. Understand the mechanisms of pedigree formation, including limitations in the current standard process. 4. Calculate the risk of having an affected child for selected individuals in pedigrees of autosomal dominant, autosomal recessive, and X-linked inheritance. Block: Foundations | SMITH [1 of 9] ILM: CLINICAL GENETICS BASICS CURRICULAR CONNECTIONS Below are the competencies, educational program objectives (EPOs), course objectives, session learning objectives, disciplines and threads that most accurately describe the connection of this session to the curriculum. Related Related COs Competency\EPO Disciplines Threads LOs CO-01 LO-01 MK-01: Core of basic sciences Genetics H & I: Medical Genetics CO-01 LO-02 MK-05: The altered structure and function Genetics H & I: Medical Genetics (pathology & pathophysiology) of the body/organs in disease CO-02 LO-03 PRO-03: Respect for patients’ race, sex, Genetics H & I: Medical Genetics ethnicity, culture, ability, disability, socioeconomic status, education level, Health Disparities language, religion, spiritual practices, sexual orientation, gender identity, geographic region, age, country of origin, education and ICS-03: Document and present patient data and clinical information in an organized, accurate, legible and/or verbally clear manner CO-01 LO-04 MK-10:L The scientific method in Genetics H & I: Medical Genetics establishing the cause of disease and efficacy of treatment, including principles of epidemiology and statistics NOTES BASIC CONCEPTS OF SINGLE GENE INHERITANCE Single-gene traits are often called Mendelian traits because they follow the patterns described by Gregor Mendel in the 1860's. Single-gene disorders are usually the result of mutations at a single genetic locus. A locus is the position of a gene on a chromosome. Alleles are alternative forms of a gene found at the same locus on homologous chromosomes. These segregate at meiosis so one is given to an individual from each parent. Normal alleles are usually referred to as wild type alleles, and abnormal alleles are usually referred to as mutant alleles. It is important to distinguish between the genotype of an individual, which is the genetic constitution; and the phenotype, which is the observable trait(s) or characteristic(s). The genotype of an individual is usually described according to the alleles present at a particular locus. Homozygotes have2 identical alleles at a locus. Heterozygotes have 2 different alleles at a locus. Hemizygotes have only 1 allele at a locus. The term hemizygote is used primarily for X-linked traits in males. Phenotypic traits may be dominant or recessive. Dominant traits are seen in both heterozygotes and homozygotes. Recessive traits are seen in homozygotes for autosomal traits and hemizygotes for X- linked recessive traits. Although strictly speaking dominant and recessive refer only to traits, in practice these terms are also applied to genes and disorders (as in "a dominant gene" or "a dominant disorder”). Block: Foundations | SMITH [2 of 9] ILM: CLINICAL GENETICS BASICS A pedigree is the shorthand language used to communicate family structure and therefore to understand patterns of single-gene inheritance. Please refer to the figure following this paragraph and commit to memory the pedigree symbols that will allow you to understand this "language." Common terminology is used to describe members of a pedigree. The proband is the first affected family member who seeks medical attention and is thus the person through whom genetic disease is ascertained in a family. Brothers and sisters are called siblings, or sibs, and their relationship constitutes a sibship. First, second, and third-degree relatives are classified according to their degree of allele sharing, but in pedigree terms can be determined from the number of intervening relatives between them in the pedigree. Couples who are related are known as consanguineous – this is reserved for couples who are second cousins or closer in relation although some societies only consider first cousins and closer in relation only as consanguineous. Pedigree Symbols Genetic heterogeneity is a term used to describe the phenomenon where the same phenotype is caused by different genotypic abnormalities. Allelic heterogeneity occurs when the abnormal phenotype is caused by different mutations at the same locus. A good example of this is cystic fibrosis (CF), where hundreds of mutations of the CFTR protein have been described, all leading to the CF phenotype. Locus, or nonallelic heterogeneity is used to describe the situation where a particular phenotype can be the result of mutations at 2 or more separate loci. A good example of this phenomenon is congenital sensorineural deafness, in which dominant, recessive, and X-linked forms have all been described. AUTOSOMAL DOMINANT INHERITANCE Autosomal dominant disorders or traits are those expressed in either the heterozygote or the homozygote. Although there are some exceptions, the features most often seen in pedigrees for autosomal dominant disorders are as follows: (1) the phenotype appears in every generation; (2) any child of an affected parent has a 50% chance of inheriting the trait; (3) phenotypically normal family Block: Foundations | SMITH [3 of 9] ILM: CLINICAL GENETICS BASICS members do not transmit the trait to their children; and (4) males and females are equally likely to transmit the phenotype to their offspring of either gender. Male to male transmission is the cardinal feature distinguishing autosomal dominant from X-linked traits. Affected Parent Normal Parent In assessing the risk to an offspring for inheriting an autosomal trait, I will use a diagram showing a mating between an affected (heterozygous for the mutant allele) and an unaffected (homozygous for the wild-type allele)individual. Those of you more familiar with Punnett squares will recognize the figure above. No matter the method of illustration, the basic concepts remain the same. First, assume that the affected individual has 2 different alleles at this autosomal locus (i.e., he or she is heterozygous): D denotes the [dominant] mutant allele, while d denotes the wild-type allele. The unaffected individual is homozygous for the d allele. From this diagram and from the example presented in class it can be seen that an affected individual has a 50% chance that his or her child will have the same trait, regardless of the gender of the child or the parent. Although almost all genetic disorders display the 3 following features, they are often most striking for autosomal dominant disorders: pleiotropy, variable expression, and reduced penetrance. Mutant genes usually produce their effects on multiple organ systems and functions and, are therefore, said to exhibit pleiotropy. For example, individuals with Marfan syndrome most notably have aortic root dilation and an increased risk of dissection or rupture; however, there are pleiotropic effects on other organs and organ systems such as the skeleton, the eye, and the dura of the spinal cord. Variable expression is said to occur when individuals with the same genotype have different phenotypes. An example of this phenomenon concerns the condition neurofibromatosis, type I (NF). Within a single family, there is marked variation in the number and location of pigmented skin lesions, benign peripheral nerve sheath tumors, learning disability and/or intracranial (brain) tumors that can be life-threatening. Some of the variation in NF is age-related—people develop more visible manifestations as they get older (Age-related penetrance; see below). Reduced penetrance may also be a feature of autosomal dominant conditions. Penetrance is an all-or-none phenomenon. That is, one either shows some features of the genotype or none at all. In this way, it can be thought of as the extreme end of the spectrum of variable expressivity. Expressed mathematically, penetrance equals the number of individuals of a particular genotype who have the disorder divided by the total number of individuals with the genotype. For example, having a deleterious BRCA1 mutation confers a lifetime risk of cancer of approximately 80%. Thus 20% of people with the same mutation will never develop cancer and the penetrance of the mutation is about 80%. We will be reviewing all of these conditions in week #4 of the Foundations block. New mutations may be common in some autosomal dominant conditions. The survival of mutations in the gene pool is directly related to the fitness of persons carrying the mutation. The fitness of a condition is measured by the number of affected persons who are able to survive to reproductive age and to have offspring. If a disorder has a fitness of 0 (f=0), then all cases represent new mutations; and, if a disorder has a fitness of 1, then new mutation is presumed to be very uncommon. In fact, in Huntington's disease, which is believed to have a fitness of 1, molecular genetic analysis has shown very, very few new mutation cases. By contrast, the disorder known as thanatophoric dysplasia, a skeletal dysplasia that is invariably lethal in the newborn period has a fitness of 0, and all cases are therefore new mutations. Advanced paternal age is associated with new mutations for autosomal dominant disorders. This is not surprising when one considers the number of mitoses through which a germ cell has gone in older males and therefore the increased likelihood of error. Block: Foundations | SMITH [4 of 9] ILM: CLINICAL GENETICS BASICS AUTOSOMAL RECESSIVE INHERITANCE Autosomal recessive phenotypes are less common than autosomal dominant conditions. They are expressed only in homozygotes, who have inherited one mutant allele from each parent. The criteria for autosomal recessive (AR) traits are as follows: (1) an AR trait generally appears only in the sibship of the proband; (2) the recurrence for siblings of affected individuals is 1 in 4. If a sibling is unaffected, then the sibling has a 2 out of 3 chance of being a carrier (i.e., heterozygous); (3) parents of affected individuals may sometimes be consanguineous. This is especially true for rare recessive disorders; (4) parents of an affected person are asymptomatic carriers of mutant alleles; and (5) males and females are equally likely to be affected for most AR conditions. Carrier Parent Carrier Parent To explain the risk of an affected offspring to 2 carrier (heterozygous for the mutant allele) parents, please refer to the figure above. R represents the wild-type allele, and r represents the mutant allele. As you can see, the four possible combinations of alleles from the parents show that the chance is 1/4 for a homozygous normal offspring, 2/4 for a carrier, and 1/4 for an affected child. For rare autosomal recessive disorders, the chance that the parents are consanguineous is increased. It has long been known that the tendency for children with rare disorders to be the product of first-cousin matings is increased. This information has led to the prohibition of consanguineous marriages in many states. The increased risk to offspring of consanguineous matings is the result of homozygosity by descent. When one is homozygous by descent, the mutant alleles present in the affected [homozygous] individual are both derived from a single common ancestor. This phenomenon is also referred to as a founder effect. The tendency of rare autosomal recessive disorders to be more common in consanguineous mating means that inquiry about consanguinity is important in cases of AR disorders. However, collectively in consanguineous matings, the increased risk for an abnormal offspring is only about 1% above the general population. Some AR disorders are concentrated within genetic isolates. Tay-Sachs disease is a characteristic example of this phenomenon. The carrier rate in Ashkenazi Jews (those of Eastern European heritage) is approximately1 in 30, compared to about 1 in 300 in the non-Jewish population. This concentration of mutant genes in isolated populations represents another example of a founder effect since the mutation in most Jews is of one type and probably derived from a single ancestral individual. In another genetic isolate, French Canadians, the carrier rate is also increased, but the mutation is of a different type. Heterozygote detection is now sought by most Ashkenazi Jews, and this has resulted in a marked decrease in Tay- Sachs within this population. The basis of arranged marriages in some orthodox Jewish sects considers the heterozygote “carrier” status of the couple. X-LINKED INHERITANCE: GENERAL PRINCIPLES X-linked disorders are conditions for which the locus is on the X chromosome. Because of this, both the inheritance and the clinical expression of these disorders are different between males and females. This is primarily the result of the fact that males have only one allele (hemizygous) for X-linked Block: Foundations | SMITH [5 of 9] ILM: CLINICAL GENETICS BASICS genes, whereas females have 2 alleles. While they are often discussed as recessive or dominant traits, severity of these conditions in females falls along a continuum because of the presence of variable inactivation of the affected and unaffected X chromosomes. To understand X-linked inheritance, it is first important to understand methods of X-chromosome gene expression and X- inactivation. The principle of X-inactivation and of gene expression is often referred to as the Lyon hypothesis, as described by Mary Lyon in1961. These principles can be summarized as follows: In female somatic cells only one X is active. The second X is condensed and is represented as the Barr body in interphase cells. Inactivation is an early embryonic event. The inactive X may be either the paternal or maternal X. Once a particular X is inactivated, all the clonal descendants of that cell will have the same inactive X. The Lyon hypothesis explains dosage compensation and variable expression in female heterozygotes. Dosage compensation refers to the finding that females express only one allele (i.e., one "dose") of their X-linked genes, although they actually have two alleles (i.e., two "doses"). This ensures that females do not produce twice the gene product of their male counterparts, who have only one allele for X-linked genes. Variable expression in female heterozygotes may also be the result of Lyonization. Because X- inactivation is a random event most females will have the paternally- derived X as the active X in about half their cells and the maternally- derived X as the active X in about half. However, some individuals will have proportions that will deviate from this average; and, depending on the relative proportions of active and inactive X-linked genes in a female heterozygous for a mutation, she will have a milder or more severe phenotype. In humans, for some tissues such as muscle cells in heterozygous females with mutations for Duchenne muscular dystrophy, mosaicism can be demonstrated clearly. There will be 2 populations of cells within her muscle: a population that has no dystrophin present because the active X in that cell line carries the dystrophin mutation; and a population with normal dystrophin because the active X carries the wild-type allele. Some animals clearly express mosaicism for coat color, which is often coded for by an X- chromosome. X-LINKED RECESSIVE INHERITANCE X-linked recessive disorders, like autosomal conditions, follow a characteristic inheritance pattern which should be readily recognizable by pedigree analysis. Criteria for X-linked recessive inheritance include the following: (1) the incidence of the trait is much higher in males than females; (2) the male offspring of carrier females have a 50% chance of being affected and the female offspring have a 50% chance of being carriers; (3) the mutant gene is transmitted from an affected man to all his daughters, who are therefore all carriers; (4) the mutant gene is never transmitted from father to son; (5) affected males in a kindred are related through females; and (6) heterozygous females are usually unaffected but may show variable expression of the condition. As with the other types of inheritance, depicted on the left (below) is a Punnett square illustration of X-linked recessive inheritance in a carrier mother and normal father. X represents the normal allele, and x represents the mutant allele. As you can see, in X-linked types of inheritance, the sex of the offspring influences the expression of the condition, owing largely to the Lyon hypothesis discussed above. With X- linked recessive inheritance and a carrier [heterozygous] female, the chance is 1/2 that any boy she has will be affected; and the chance is 1/2that any daughter she has will be a carrier. Block: Foundations | SMITH [6 of 9] ILM: CLINICAL GENETICS BASICS Normal Mother Carrier Mother Affected Father Regarding the offspring of an affected male, all his daughters will be carriers, while all his sons will be normal. This is illustrated by the figure depicted on the right. Hemophilia A, or factor VIII deficiency, is a characteristic X-linked disorder which has been described since ancient times. For example, Talmudic writings allowed a boy to forego circumcision if a cousin or olderbrother had died from this procedure. Additional historical importance derives from the presence of the mutant gene in many of the royal families of Europe. A carrier female has a 50% chance that her son will have hemophilia and a 50% chance that a daughter will be a carrier. Previously, carrier detection in females who had not reproduced was most often performed by assaying factor VIII activity. In keeping with principles of Lyonization, carrier females have approximately 50% the factor VIII activity of non-carrier females and normal males. However, it is now possible in most cases to apply molecular genetic analysis to track the mutant allele in at-risk families. Because of increased survival (and therefore increased genetic fitness) of affected males, it is not uncommon that hemophiliacs have children. In keeping with principles of X-linked inheritance, all an affected male's sons will be normal, and all his daughters will be carriers. You will learn about hemophilia in week #2 of Foundations. Duchenne muscular dystrophy (DMD) is another well-known X-linked recessive genetic disorder. It is caused by mutations of the muscle protein, dystrophin. The gene that codes for dystrophin is massive, longer than any other known gene, making it a more prominent target for mutation than other genes. Because affected males do not reproduce, DMD can be termed a genetic lethal, with a genetic fitness of 0. It stands to reason that, since one-third of all DMD genes are carried in males and since these males do not reproduce (i.e., they have a genetic fitness of 0), then one-third of all cases of DMD must be the result of a new mutation. The same can be said of any [genetically] lethal X-linked disorder. You will learn more about Duchenne Muscular Dystrophy in the MSK block. X-LINKED DOMINANT INHERITANCE X-linked dominant traits are those which are regularly expressed in heterozygotes. Criteria for X- linked dominant inheritance include the following: (1) affected males have no affected sons and only affected daughters (no normal daughters); (2) both male and female offspring of heterozygous females have a 50% chance of being affected; (3) for rare phenotypes, affected females are twice as common as affected males and usually have milder expression of the phenotype; and (4) for conditions which are prenatal lethal in hemizygous males, the sex ratio of offspring of heterozygous mothers is 2:1 female to male, since all affected male conceptuses are spontaneously aborted. Block: Foundations | SMITH [7 of 9] ILM: CLINICAL GENETICS BASICS Normal Mother Affected Mother Affected Father The figure on the right illustrates the possible genotypes of offspring of an affected mother. X represents the mutant allele, and x represents the wild-type. As you can see, the risk is 50% for having an affected child. Ifthe affected child is male, the phenotype is more severe, while it is milder in females because of Lyonization, which results, on average, in half her X's expressing the mutation and in half expressing the normal allele. As can be seen from the illustration on the left, an affected father has only affected daughters. All his sons are normal. Some examples of X-linked dominant conditions include Alport Syndrome (inherited kidney disease stemming from defects in collagen genes leading to hematuria, end-stage kidney failure, hearing loss, and cataracts) and Incontinentia Pigmenti (disease affecting skin, hair, teeth, nails, and central nervous system including retinal development). GENETIC TESTING IN CLINICAL PRACTICE Clinical Presentation of Person to Genetic Testing be Tested Result Interpretation Recommendations for Family Members True positive. Disease-causing variant identified. Test confirms clinical diagnosis or clinical Targeted testing of first-degree family Affected Positive suspicion. members is strongly recommended. The result does not rule out the Affected Negative diagnosis or a genetic cause. Testing of family members not indicated. Affected Variant of The result, at this time, is Syndrome – parental testing to determine uncertain potentially uninformative. significance inheritance. De Novo variant may be (VUS) considered likely pathogenic. Finding the variant in an unaffected parent supports benign familial variant. Cancer - Testing of family members not typically indicated. Block: Foundations | SMITH [8 of 9] ILM: CLINICAL GENETICS BASICS Clinical Presentation of Person to Genetic Testing Result Interpretation Recommendations for Family Members be Tested Patient is at risk for developing Targeted testing of first-degree family Unaffected Positive cancer/phenotype. members is strongly recommended. Patient is likely not at increased risk of cancer, but a genetic cause cannot be ruled out Testing of family members may be Unaffected Negative unless familial variant is known. indicated, depending on the family history. Variant of uncertain significance The result, at this time, is Testing of family members not typically Unaffected (VUS) uninformative. indicated. Block: Foundations | SMITH [9 of 9]