Genetics Exam 3
Document Details
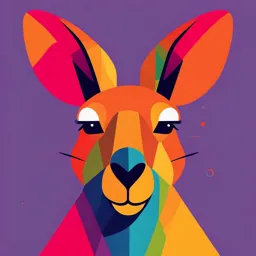
Uploaded by SupportiveAlbuquerque
BCMB 401
Tags
Summary
This document provides an overview of the DNA repair system and different types of mutations. It details proofreading, photoreactive, excision, and double-stranded break repair mechanisms. The document also covers various types of mutations (e.g., missense, nonsense, silent, transition, and transversion) and their causes, both spontaneous and induced.
Full Transcript
Highlight code: This color: definitions to know This color: molecules to know (usually proteins/enzymes) This color: people/ experiments to know DNA repair system 1. Proofreading and mismatch repair - Incorrect nucleotides are cut and removed by endonucleases and exonucleases, and the correct nucleo...
Highlight code: This color: definitions to know This color: molecules to know (usually proteins/enzymes) This color: people/ experiments to know DNA repair system 1. Proofreading and mismatch repair - Incorrect nucleotides are cut and removed by endonucleases and exonucleases, and the correct nucleotide is then replaced by DNA polymerase 2. Photoreactive repair - Photolyase cleaves a thymine dimer, making two thymine monomers. Photolyase uses light energy to break bonds between the dimers - A thymine dimer is a type of pyrimidine dimer that is formed when DNA is exposed to UV light. A pyrimidine dimer is made of two identical pyrimidines and it can distort the DNA and introduce errors - Humans don’t have photoreactive repair 3. Excision repair - A type of repair mechanism that does not need light (unlike photoreactive repair). Mechanism for excision repair is the same as mismatch repair - Base excision repair- DNA glycosylase recognizes an incorrect base, which is then replaced - Nucleotide excision repair- fixes DNA lesions. Lesions are damages to the DNA chemical structure, such as DNA breakage breaks. NER does not replace bases. An example of NER would be the uvr gene, which repair UV light damage - Xeroderma Pigmentosum is a genetic disorder affecting NER pathways. People with it are more likely to develop cancer 4. Double stranded break repair - The reattaching of DNA strands after they break. There are two different ways this repair can happen: homologous recombination repair and nonhomologous end joining - Homologous recombination: genetic material is swapped between homologous DNA molecules. In bacteria and archaea, this happens during conjugation if DNA damage occurs. In eukaryotes, this happens during prophase I of meiosis. - Nonhomologous end joining: occurs in G1 before replication of the DNA and repairs double strand breaks. It may involve kinase and BRCA1. Mutations Background - Luria and Delbrook proved the random mutation hypothesis - Adaptation hypothesis- mutations arise from adaptations to the environment - Random mutation hypothesis- mutations occur randomly and not in response to the environment Types: missense, nonsense, silent, transition, and transversion - Missense: a type of point mutation in which the changing of a base results in a triplet code that codes for a different amino acid - Nonsense: a type of point mutation in which the changing of a base results in a stop codon and translation is stopped early - Silent: a type of point mutation in which the the changing of a base results in a triplet code that codes for the same amino acid as before - Transition: a type of frameshift mutation in which pyrimidine (single ringed base) replaces another pyrimidine or a purine (double ringed base) replaces another purine - Transversion: a type of frameshift mutation in which a pyrimidine is swapped for a purine What causes mutations? 1. Spontaneous (occurs naturally) - DNA polymerase can make mistakes during replication - Replication slippage: the template strand of DNA can form loops during replication, and DNA polymerase can skip over these loops, leaving them out and resulting in a daughter strand with less DNA - This is the cause of Fragile-X syndrome and Huntington’s disease - Tautomeric shifts: There are different isomers, called tautomers, of both purines and pyrimidines, and sometimes they will spontaneously switch from one isomer to another. This random switch can change how the bases bond and allow non-complementary base-pairing - Depurination: a nucleotide randomly loses a purine. The place from which the purine was lost is called an apurinic site and DNA polymerase will usually fill in this site with an adenine - Deamination: a nucleotide randomly loses an amino group. When this happens, the site where the amino group was is filled with something else like oxygen. This can change the bases (if cytosine is deaminated and an oxygen is added, it changes to uracil). 2. Induced (occurs due to outside factors) - Occur due to either chemical mutagens, a type of radiation, or transposable DNA elements - Base analogs are chemical mutagens that can replace purine or pyrimidines and increase tautomeric shifts and UV radiation sensitivity - Deaminating agents are chemical mutagens that remove amino groups - Alkylating agents are chemical mutagens that add bulky carbon chains like methyls or ethyls to bases. The groups that are added are called bulky adducts, and they mess with base pairing and distort the double helix. The addition of these can cause frameshift mutations - Hydroxylating agents are chemical mutagens that add hydroxyl groups (OH groups) to bases - Other chemical mutagens can oxidize molecules (bleach and hydrogen peroxide can do this) and fit between base pairs, which distorts the double helix - UV radiation can create pyrimidine dimers that distort the DNA - Ionizing radiation such as X rays, gamma rays, and cosmic rays ionize molecules that can go on to produce reactive oxygen molecules that damage DNA - Transposable DNA elements can move between genomes and cause inversions, translocations, double strand breaks, and chromosome damage - Carcinogens are a type of mutagen that cause cancer The Ames test uses bacteria to figure out if a particular chemical can cause DNA mutations in another test organism Gene regulation in Eukaryotes 1. Transcriptional regulation - Activator proteins bind regulatory sequences to stimulate transcription - Repressor proteins bind other sequences to stop or slow translation. In eukaryotes, both activator and repressor proteins are in large group complexes - Transcription is also regulated by DNA sequences called enhancers and silencers. Both of these are cis-acting, which means they are non-coding DNA regions that regulate transcription of nearby genes. Trans-acting elements, in contrast, are coding regions of DNA that code for regulators - Enhancers can be located anywhere in relation to a gene and allow for maximum level of transcription to be reached. They can enhance any gene nearby - Silencers block enhancers so that they can only “enhance” the target gene - Transcription can be regulated depending on the chromatin structure. Compact chromatin structure inhibits transcription and DNA repair - Methylation of the DNA inhibits transcription 2. mRNA processing- not important for this exam 3. Regulation of mature mRNA - RNA-induced gene silencing is a type of RNA interference (RNAi) in which short RNA molecules regulate translation. Examples include siRNA and microRNA - siRNA: form when there is a viral infection and produce double stranded RNA. the enzyme dicer cleaves this double stranded RNA - microRNA: noncoding RNA that inhibits gene expression - RISC: RNA induced silencing complex - RITS: RNA induced transcriptional silencing complex 4. Translational regulation- not important for this exam 5. Post-translational regulation- not important for this exam Gene regulation in Bacteria Bacterial genes need either continuous transcription or regulated transcription and most of the regulation of bacterial genes occurs during transcription. There are two types of transcriptional regulation: 1. Negative control- a repressor protein binds to a regulatory DNA sequence and inhibits transcription - Repressor proteins generally have two active sites. The DNA binding domain binds DNA (wow!) and the allosteric domain binds a molecule. The binding of this molecule changes the shape of the DNA binding domain, which can either activate or deactivate the repressor protein. 2. Positive control- an activator protein binds to a regulatory DNA sequence and initiates transcription - Most activator proteins are inactive until they are bonded with an allosteric compound at the allosteric domain. - Regulatory DNA binding proteins have amino acids that can either initiate or inhibit transcription 3. The heat shock response- a type of control at the transcription level in which abnormally hot temperatures activate expression of specialized heat stress genes - An alternative sigma factor (subunit of RNA polymerase) is expressed because the usual one is unstable at hot temperatures - This new sigma factor (sigma 32) recognizes specific DNA sequences that code for heat stress genes - Chaperone proteins are proteins that inhibit sigma 32 at normal temperatures. At hot temperatures, they release sigma 32 so that it can go activate heat stress genes 4. Riboswitches- noncoding mRNA segments that control the expression of downstream genes by binding a metabolite Lac and Trp Operons An operon is a region of linked genes that are all under control of one promoter. A promoter is a section of DNA where transcription is initiated. An operator is a binding site for a repressor 1. Lac operon- encodes for the enzyme Beta-galactosidase, which degrades lactose into glucose and galactose - The Lac operon is usually off- when glucose is available, it is more energetically favorable to use it rather than lactose. Only if lactose is present and glucose is not does the Lac operon turn on - Presence of glucose inhibits production of an enzyme called adenylate cyclase. Adenylate cyclase produces cAMP. When glucose levels are low, cAMP levels are high. cAMP then binds to a molecule called “CRP” and this CRP-cAMP complex binds to the lac promoter and turns on the lac operon. This is positive control (of the operon) - When the CRP-cAMP complex is not bound to the promoter, transcription of lacZYA is very low. This is called catabolite repression - The operon itself consists of three genes: lacZ, lacY, and lacA - Is repressed by a gene called lacI, located upstream. It prevents transcription of lacZYA. This is negative control - Also has an inducer molecule, allo-lactose. Allo-lactose binds to lacI, preventing it from inhibiting transcription. This is positive control - A partial diploid bacteria has one copy of the lac operon with a mutant promoter sequence (P- rather than P+) and a plasmid with a mutant lacI repressor (I-) 2. Trp operon- encodes for the amino acid tryptophan - - Tryptophan can be found naturally in the environment, or it can be made by the trp operon. If it is found naturally, tryptophan will inhibit the trp operon because the cell does not need to make any more The operon itself consists of five genes, trp E, D, C, B and, A It contains a promoter and an operator Tryptophan acts as a corepressor by switching the repressor protein on. This is called feedback inhibition Attenuation is a mechanism by which transcription is prevented from finishing. It makes RNA polymerase stop transcribing early when tryptophan levels are high. Attenuation is controlled by the trpL region Translation tRNA- transfer RNA - Bind to mRNA and carry a codon’s corresponding amino acid - Has cloverleaf structure - Before translation can happen, tRNAs must be bonded to their respective amino acids. They are bonded by the enzyme synthetase. There is a different synthetase for each amino acid - Because codons that code for the same amino acids generally only differ in the last base, tRNAs can exhibit a property called a third base wobble, where the bond between the second and third base is weaker Ribosomes- carry tRNAs and bond together the codons - Have a large and small subunit. In eukaryotes theses subunits are bigger - P site of the ribosome holds tRNA - A site binds a new tRNA - E site is where tRNA leaves Steps of translation 1. Initiation - In prokaryotes, the ribosome binds at the Shine-Dalgarno sequence, which precedes the start codon. It basically lines the ribosome up to begin translation - Initiation factors help facilitate translation - The two ribosomal subunits join 2. Elongation - Elongation factor protein attach to a tRNA, which pairs to the ribosomal A site - Peptide bonds are formed between amino acids by enzyme peptidyl transferase - tRNA travels like: A site → P site → E site 3. Termination - Release factors bind a stop codon on ribosomal A site Protein structure levels 1. Primary- sequence of amino acids 2. Secondary- alpha helix and beta pleated sheet 3. Tertiary- 3D protein 4. Quaternary- one or more 3D polypeptides