Molecular DNA Technology: Genetic Engineering Notes PDF
Document Details
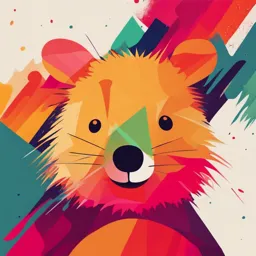
Uploaded by FriendlyTrust
University of KwaZulu-Natal
Tags
Summary
These notes cover genetic engineering and recombinant DNA technology, including the use of restriction enzymes. They describe the process and the tools used in molecular biology for manipulating DNA.
Full Transcript
University of KwaZulu-Natal Department of Biochemistry RDNA202 - Molecular DNA Technology Genetic Engineering 1. INTRODUCTION Genetic engineering is a broad scientific term that refers to artificial manipulation (by removal, addition or modification) of an organisms’ nucle...
University of KwaZulu-Natal Department of Biochemistry RDNA202 - Molecular DNA Technology Genetic Engineering 1. INTRODUCTION Genetic engineering is a broad scientific term that refers to artificial manipulation (by removal, addition or modification) of an organisms’ nucleic acid content. It differs from genetic recombination in that it does not occur through natural processes within the cell, but is human-made. Organisms whose genes have been artificially altered for a desired purpose (either making new metabolites or enhancing function) is often called genetically modified organism (GMO). Recombinant DNA (rDNA) is a form of artificial DNA that is created by combining two or more sequences that would not normally occur together (from different or heterologous sources). In certain instances, it has provided the means to produce large amounts of highly purified normal and mutant proteins for detailed analysis of their function in the organism. rDNA is also referred to as chimeric DNA. The latter term is derived from chimera, a monster in Greek mythology that consisted of a lion’s head, goat’s body and a serpent’s tail. A recombinant protein is a protein that is derived from recombinant DNA. In biology, a clone is a group of genes, cells, or organisms derived from a single common ancestor. The terms DNA cloning, molecular cloning and gene cloning all refer to the same process: the transfer of a DNA fragment (insert or foreign DNA) of interest from one organism to an autonomous- or self-replicating genetic element (vector) such as a bacterial plasmid. The DNA of interest can then be propagated in both high quantities in a foreign host cell and isolated in pure form so as to facilitate the study of genes. This technology has been around since the 1970s, and it has become a common practice in molecular biology laboratories today. The basic principles of recombinant DNA, like the structure of DNA itself, are surprisingly simple. 2 Figure 1: By fragmenting DNA of any origin (human, animal, or plant) and inserting it in the DNA of rapidly reproducing foreign cells, billions of copies of a single gene or DNA segment can be produced in a very short time. The DNA fragment of interest from an organism such as a human is incorporated into the 'plasmid DNA' of a bacterial cell. A plasmid is a circular self-replicating DNA molecule that is separate from the bacterial DNA. When the recombinant plasmid is introduced into bacteria, the newly inserted segment will be replicated along with the rest of the plasmid. 3 Recombinant DNA technology is used to achieve the following: i. Study the arrangement, expression and regulation of genes ii. Modification of gene expression either to enhance or suppress a particular product iii. Artificially produce multiple copies of a nucleic acid segment iv. Introduction of genes from one organism to another, thus creating a transgenic organism with desirable or altered characteristics. v. Creation of transgenic organism so that they can be commercially employed as cell factories. 2) ENZYMES USED IN CLONING 2.1) Restriction endonucleases (RE) Restriction enzymes (RE) are endonucleases that recognize a specific, rather short, nucleotide sequence on a double-stranded DNA molecule, called a restriction site, and cleave the DNA at this recognition site or elsewhere. They are of bacterial origin These enzymes were so named because of their ability to ‘restrict’ growth of bacteriophages in certain bacterial strains. There are three major classes of restriction endonucleases. Their grouping is based on the types of sequences recognized, the nature of the cut made in the DNA, and the enzyme structure. Type I and III restriction endonucleases are not useful for gene cloning because they cleave DNA at sites other than the recognition sites and thus cause random cleavage patterns. In contrast, type II endonucleases are widely used for mapping and reconstructing DNA in vitro because they recognize specific sites and cleave just at these sites (Table 1). 4 Table 1: Major classes of restriction endonucleases. Use in Class Abundance Recognition site Composition recombinant DNA research Type I Less common than Cut both strands at a Three-subunit complex: Not useful type II nonspecific location > individual recognition, 1000 bp away from endonuclease, and recognition site methylase activities Type II Most common, Cut both strands at a Endonuclease and Very useful approximately 240 specific, usually methylase are separate, enzymes commercially palindromic, recognition single-subunit enzymes available site (4-8 bp) Type III Rare Cleavage of one strand Endonuclease and Not useful only, 24-26 bp methylase are separate downstream of the two-subunit complexes 3′recognition site with one subunit in common 2.1.1) Restriction endonuclease nomenclature Restriction endonucleases are named for the organism in which they were discovered, using a system of letters and numbers. For example, HindIII (pronounced “hindee-three”) was discovered in Haemophilus influenza (strain d). The Hin comes from the first letter of the genus name and the first two letters of the species name; d is for the strain type; and III is for the third enzyme of that type. SmaI is from Serratia marcescens and is pronounced “smah-one”. EcoRI (pronounced “echo-r-one”) was discovered in Escherichia coli (strain R). BamHI is from Bacillus amyloliquefaciens (strain H). Over 3000 type II restriction endonucleases have been isolated and characterized to date. Approximately 240 are available commercially for use by molecular biologists. 5 2.1.2) Recognition sequences for type II restriction endonucleases In the presence of the essential cofactor Mg2+, the enzyme cleaves the DNA on both strands at the same time within or in close proximity to the recognition sequence (restriction site). Each orthodox type II restriction endonuclease is composed of two identical polypeptide subunits that join together to form a homodimer. These homodimers recognize short symmetric DNA sequences of 4–8 base pairs. Six base pair cutters are the most commonly used in molecular biology research. Usually, the sequence read in the 5′ → 3′ direction on one strand is the same as the sequence read in the 5′ → 3′ direction on the complementary strand (palindrome). The enzyme cuts the DNA duplex by breaking the covalent, phosphodiester bond between the phosphate of one nucleotide and the sugar of an adjacent nucleotide, to give free 5′-phosphate and 3′-OH ends (Figure 2). The exact mechanism by which restriction endonucleases achieve DNA cleavage has not yet been proven experimentally for any type II restriction endonuclease. Some enzymes, such as EcoR1, generate a staggered cut, in which the single- stranded complementary tails are called “sticky” or cohesive ends because they can hydrogen bond to the single-stranded, complementary tails of other DNA fragments. Other type II enzymes, such as SmaI, cut both strands of the DNA at the same position and generate blunt ends with no unpaired nucleotides when they cleave the DNA. PO4− PO4− 5’ sticky or cohesive ends Figure 2: Cleavage patterns of some common restriction endonucleases. 6 PO4− PO4− Blunt ends PO4− PO4− 5’ sticky or cohesive ends PO4− PO4− 5’ sticky or cohesive ends 5’--G-A-G-C-T-C--3’ SacI 5’--G-A-G-C-T-OH PO4−-C--3’ 3’--C-T-C-G-A-G--5’ 3’--C-PO4− OH-T-C-G-A-G--5’ 3’ sticky or cohesive ends Figure 2 continued: Cleavage patterns of some common restriction endonucleases. 2.2) Ligases DNA ligases catalyze formation of a phosphodiester bond between the 5′- phosphate of a nucleotide on one fragment of DNA and the 3′-hydroxyl of another (Figure 3). This joining of linear DNA fragments together with covalent bonds is called ligation. Unlike the type II restriction endonucleases, DNA ligase requires ATP as a cofactor. If restriction-digested fragments of DNA are placed together under appropriate conditions, the DNA fragments from two sources can anneal to form recombinant molecules by hydrogen bonding between the complementary base pairs of the sticky ends. However, the two strands are not covalently bonded by phosphodiester bonds. 7 DNA ligase is required to seal the gaps, covalently bonding the two strands and regenerating a circular molecule. The DNA ligase most widely used in the lab is derived from the bacteriophage T4. T4 DNA ligase will also ligate fragments with blunt ends, but the reaction is less efficient and higher concentrations of the enzyme are usually required in vitro. Source one DNA Source two DNA 5’--G-G-A-T-C-C--3’ 5’--G-G-A-T-C-C--3’ 3’--C-C-T-A-G-G--5’ 3’--C-C-T-A-G-G--5’ Cleavage with BamHI to generate fragments with sticky ends 5’--G G-A-T-C-C--3’ 3’--C-C-T-A-G G--5’ Base pairing of complimentary tail regions Gap 5’--G G-A-T-C-C--3’ 3’--C-C-T-A-G G--5’ Gap T4 DNA Ligase 5’--G-G-A-T-C-C--3’ 3’--C-C-T-A-G-G--5’ Ligated source one-two DNA Figure 3: Recombinant DNA molecules can be formed from DNA cut with restriction endonucleases that sticky or cohesive ends, such as BamHI. The sticky tails allow DNA fragments from two different sources to anneal. “Source 1” DNA and “source 2” DNA are then covalently linked by treatment with DNA ligase to create a recombinant DNA molecule. Note that the BamHI site is regenerated in the process. 8 2.3) Terminal deoxynucleotidyl transferase (terminal transferase) These enzyme catalyses the addition of deoxynucleotides to the 3′OH ends of DNA molecules. How is it different to the requirements of DNA polymerase III? Source one DNA Source two DNA 5’--C-C-C G-G-G--3’ 3’--G-G-G C-C-C--5’ Add poly (dT) tails Terminal deoxynucleotidyl transferase Add poly (dA) tails 5’--C-C-C-T-T-T G-G-G--3’ 3’--G-G-G A-A-A-C-C-C--5’ Base pairing of complimentary tail regions Gap 5’--G-G-G-T-T-T G-G-G--3’ 3’--C-C-C A-A-A-C-C-C--5’ Gap T4 DNA Ligase 5’--G-G-G-A-A-A-G-G-G--3’ 3’--C-C-C-T-T-T-C-C-C--5’ Ligated source one-two DNA Figure 4: Recombinant DNA molecules can be formed from DNA cut with restriction endonucleases that leave blunt ends, such as SmaI. Without end modification, blunt end ligation is of low efficiency. The efficiency is increased through using the enzyme terminal deoxynucleotidyl transferase to create complementary tails by the addition of poly(dA) and poly(dT) to the cleaved fragments. These tails allow DNA fragments from two different sources to anneal. Source 1 DNA and source 2 DNA are then covalently linked by treatment with DNA ligase to create a recombinant DNA molecule. Note that the SmaI site is destroyed in the process. 9 2.4) Phosphatases In cloning, the ligase reaction is used to covalently join passenger DNA to the vector DNA. However, it must be noted that the vector DNA can also self- anneal and be ligated without any foreign or passenger DNA inserts. This is not desirable. A 10: 1 ratio of passenger DNA to vector DNA to increase the possibility of passenger DNA – vector DNA annealing reactions and subsequently being ligated. Alternatively, calf intestinal phosphatase is employed to remove the 5′ phosphate from linearized vector DNA. Thus vector DNA will not ligate to itself since the 5′ phosphate is required for this reaction. Since the passenger DNA has 5′ phosphate, there will be some ligation due to the two 5′ phosphates provided passenger DNA. However no ligation will take place at the two other ligation points where the 5′ phosphates are provided by the vector DNA. This chimeric DNA with two unsealed gaps are still able to circularize and can therefore be transformed into cloning host where repair enzymes seal the gaps. 2.5) Large fragment of DNA polymerase I (Klenow fragment) This 76 kD enzyme is produced by cleavage of intact DNA polymerase I with subtilisin. This enzyme component has 5′-3′ polymerase activity and 3′-5′ exonuclease activity but lacks 5’-3′’ exonuclease activity of the intact enzyme. The Klenow fragment is employed for a variety of purposes including: - DNA sequencing using the Sanger dideoxy system. - Filling the recessed 3′ termini created by digestion of DNA with restriction enzymes. - Labeling the termini of DNA fragments using [32P] dNTPS in end-filling reactions. - Second strand synthesis in cDNA cloning. 10