Genetic and Epigenetic Control Mechanisms 2024 PDF
Document Details
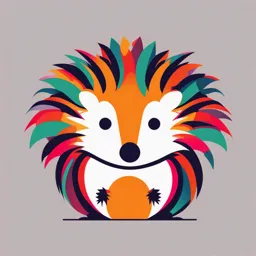
Uploaded by NoiselessIguana9011
Lokman Hekim Üniversitesi
2024
Selen Güçlü Durgun, PhD
Tags
Summary
This document covers genetic and epigenetic control mechanisms, emphasizing gene regulation in prokaryotes and eukaryotes. It also explains the role of epigenetic mechanisms, such as DNA methylation and histone modifications, in gene expression. The content is aimed towards a postgraduate audience at Lokman Hekim University.
Full Transcript
/ Genetic and Epigenetic Control Mechanisms Selen Güçlü Durgun, PhD Faculty of Medicine Learning Outcomes Define the importance of gene regulation for organisms Define and differentiate prokaryotic and eukaryotic control mechanisms Describe bacterial operon systems...
/ Genetic and Epigenetic Control Mechanisms Selen Güçlü Durgun, PhD Faculty of Medicine Learning Outcomes Define the importance of gene regulation for organisms Define and differentiate prokaryotic and eukaryotic control mechanisms Describe bacterial operon systems as genetic control mechanisms Describe key epigenetic mechanisms in eukaryotic cells, including DNA methylation and histone modification, and their role in regulating gene expression. Describe the connection between the regulation of gene expression and observed differences between individuals in a population https://www.slideshare.net/slideshow/operon-conceptpdf/258376933#1 2 All cells within an organism share the same genome, yet they can exhibit distinct characteristics and functions due to various gene regulation mechanisms. 3 4 How does a cell «know» what type of cell it is? Genetic Information: Each cell carries specific genes in its DNA that determine its characteristics and functions. Transcription Factors: These proteins regulate which genes are active, guiding the cell's identity. Cell Signaling: Environmental and internal signals influence the cell's development and differentiation. Cell Communication: Interactions with neighboring cells provide information that shapes cell identity. Epigenetic Modifications: Changes to DNA that affect gene expression can define the cell's identity over time. 5 At one time, did we really all look alike? We all start as a single cell and soon grow into an embryo. Think about the amazing complexity that must be going on inside the embryo, and the tremendous amount of growth and development still to come. https://www.fortislife.com/products/focus-on-stem-cell-biology 6 How is genetic expression regulated? It is now clear that gene expression varies widely in bacteria under different environmental conditions. Bacteria have been especially useful research organisms in genetics for a number of reasons. For one thing, they have extremely short reproductive cycles, and literally hundreds of generations, giving rise to billions of genetically identical bacteria, can be produced in overnight cultures. In addition, they can be studied in “pure culture,” allowing mutant strains of genetically unique bacteria to be isolated and investigated separately. Although most bacterial gene products are present continuously at a basal level (a few copies), the concentration of these products can increase dramatically when required. Clearly, fundamental regulatory mechanisms must exist to control the expression of the genetic information. 7 Cells detect environmental signals through several mechanisms: 1. Sensory Proteins: Receptor proteins located on the cell membrane detect environmental signals (hormonal, chemical, physical) and initiate intracellular signaling pathways. 2. Signal Transduction Pathways: When receptors are activated, signaling pathways inside the cell (such as kinases and phosphatases) come into play, interacting with various proteins and enzymes that regulate cellular responses. 3. Second Messenger Systems: The binding of the initial signal to its receptor can trigger the production of secondary messenger molecules (e.g., calcium ions, cAMP). These molecules interact with various target proteins to accelerate cellular responses. 4. Transcription Factors: Environmental signals can activate transcription factors that regulate gene expression. These factors bind to specific regions of DNA, controlling the opening and closing of relevant genes. 5. Epigenetic Changes: Environmental signals can induce epigenetic modifications (such as DNA methylation or histone modifications) that affect gene expression in a more permanent manner. Through these mechanisms, cells can respond quickly and effectively to changes in their environment. 8 Cells determine and communicate their needs through several key mechanisms: 1. Signal Transduction: Cells detect chemical signals from their environment, such as hormones or growth factors, which inform them about their requirements. 2. Internal Status Indicators: Cells assess their needs based on internal indicators, such as energy levels and metabolite concentrations. For example, if ATP levels are low, a cell may increase glucose uptake to boost energy production. 3. Receptors: Specific receptors on the cell membrane detect the presence of nutrients and other molecules. These receptors help cells gauge the quantity of essential substances they need. 4. Gene Expression: Cells can adjust gene expression based on their requirements. For instance, if a nutrient is lacking, genes that code for proteins needed to synthesize that nutrient may be activated. 5. Metabolic Feedback: Cells receive feedback from their metabolic products. If a certain metabolite accumulates, it can signal that the cell should not produce more of that substance or that it needs more of another. These mechanisms enable cells to dynamically assess their needs and respond appropriately. 9 Gene regulation is significantly influenced by environmental factors, which can affect how genes are expressed. Here are a few key ways environmental factors play a role: 1. Nutrient Availability: The presence or absence of certain nutrients can activate or repress specific genes, influencing metabolic pathways. 2. Temperature: Changes in temperature can lead to shifts in gene expression, particularly in organisms that rely on external temperatures for physiological processes. 3. Stressors: Environmental stressors such as toxins, UV radiation, or pathogens can trigger stress response pathways, leading to the activation of genes that help the organism cope. 4. Hormonal Signals: Environmental cues can lead to the release of hormones that bind to receptors and initiate gene expression changes, impacting growth, development, and metabolism. 5. Epigenetic Changes: Environmental factors can induce epigenetic modifications, such as DNA methylation and histone modification, which can alter gene expression without changing the underlying DNA sequence. Overall, these interactions illustrate how gene regulation is a dynamic process, shaped by both internal genetic mechanisms and external environmental conditions. Met cesyen DNA mod f cat on Aset lot ensh shan 10 11 Regulation of Gene Expression in Bacteria 12 Bacteria Regulate Gene Expression in Response to Environmental Conditions The regulation of gene expression has been extensively studied in bacteria, particularly in E. coli. Geneticists have found that bacteria use efficient systems to turn genes on and off, depending on what the cell needs. These systems help bacteria respond to changes in their environment and control important activities like DNA replication, repair, and cell division. Gao, B., & Sun, Q. (2021). Programming gene expression in multicellular organisms for physiology modulation through engineered bacteria. Nature Communications, 12(1), 14 2689. Inducable enzymes Microorganisms control the production of gene products. In 1900, it was discovered that yeast makes enzymes to break down lactose only when lactose is present. This led to the idea of inducible enzymes, which are made when needed. In contrast, constitutive enzymes are produced all the time, no matter the environment. 15 Bacterial Operons Are Coregulated Gene Clusters Being physically close in the genome, these genes are regulated such that they are all turned on or off together. Grouping related genes under a common control mechanism allows bacteria to rapidly adapt to changes in the environment. 16 The Operon Model An operon is a coordinately regulated unit of transcription in bacteria. The operon model was proposed by Jacob, Monod, and Wollman based on their genetic and biochemical studies on lactose-requiring mutations of E. coli. https://biology.arizona.edu/molecular_bio/problem_sets/mol_genetics_of_prokaryotes/01t.html 17 An operon is a unit of the bacterial chromosome consisting of the following components: The regulatory gene codes for a regulatory protein. The lac repressor, encoded by the lac I gene, is the regulatory protein of the lac operon. The operator is the region of DNA of the operon that is the binding site for the regulatory protein. The promoter is the DNA sequence of the operon recognized by DNA-dependent RNA polymerase. The initiation site for RNA synthesis is immediately downstream of the promoter. The gene for DNA- dependent RNA polymerase is not part of the operon, since the RNA polymerase enzyme transcribes all bacterial operons. The operon encodes one or more genes (structural genes) for inducible enzymes. The lac operon encodes enzymes necessary for lactose metabolism, including ß-galactosidase, ß-galactoside permease, and ß-galactoside transacetylase. The inducer is a small molecule that is a signal within the cell that lactose is available for metabolism. The natural inducer is allolactose, an isomer of lactose. https://biology.arizona.edu/molecular_bio/problem_sets/mol_genetics_of_prokaryotes/02t.html18 Regulation of gene expression, whether inducible or repressible, can involve negative or positive control. In negative control, a regulator protein is a repressor; when it binds to DNA, transcription is inhibited. Lactose In positive control, a regulatory protein is an activator; when it binds to DNA, transcription is stimulated. Some operons are inducible: their transcription is normally off and must be turned on. Other operons are repressible: their transcription is normally on and must be turned off. Both control types, or a mix, can regulate inducible or repressible systems. Tryptophan For example, the enzymes for lactose digestion and tryptophan synthesis are controlled by negative regulation. 19 In positive regulation, a transcriptional activator protein binds a region at the 5' end of a gene, called an operator, to promote transcription. In the absence of the activator protein, there is no transcription. In negative regulation, a repressor binds the operator sequence to prevent transcription. Only in the absence of repressor protein can the gene be transcribed. 20 Lactose Metabolism in E. coli Is Regulated by an Inducible System The gene activity is repressed when lactose is absent but induced when it is available. In the presence of lactose, the concentration of the enzymes responsible for its metabolism increases rapidly from a few molecules to thou- sands per cell. The enzymes responsible for lactose metabolism are thus inducible, and lactose serves as the inducer. 21 Genetic Control Mechanism In bacteria, genes that code for enzymes with related functions, like those for lactose metabolism, are often grouped together in clusters on the chromosome. These gene clusters are controlled by a single regulatory region, called an operon, located just upstream (5') of the cluster. This regulatory region is referred to as a cis-acting site because it is on the same DNA strand as the genes it controls. Trans-acting factors, which are molecules that bind to the cis-acting site, regulate transcription of the gene cluster. These factors can either turn off transcription (negative regulation) or turn it on (positive regulation), controlling whether the genes are expressed and their products synthesized. 22 The discovery of a regulatory gene and regulatory site within the gene cluster was crucial for understanding how gene expression is controlled. These regulatory elements do not encode enzymes for lactose metabolism, which is the role of the three structural genes in the cluster. The three structural genes along with the adjacent regulatory site form the lactose (lac) operon. This entire gene cluster works together to enable a quick response to the presence or absence of lactose. 23 The lac operon of E. coli contains genes involved in lactose metabolism. It's expressed only when lactose is present and glucose is absent. Two regulators turn the operon "on" and "off" in response to lactose and glucose levels: the lac repressor and catabolite activator protein (CAP). 24 The lac repressor acts as a lactose sensor. It normally blocks transcription of the operon, but stops acting as a repressor when lactose is present. The lac repressor senses lactose indirectly, through its isomer allolactose. Negative regulation Allolactose is inducable element 25 The Catabolite-Activating Protein (CAP) Exerts Positive Control over the lac Operon: Catabolite activator protein (CAP) acts as a glucose sensor. It activates transcription of the operon, but only when glucose levels are low. CAP senses glucose indirectly, through the "hunger signal" molecule cAMP. Glucose – Positive repressible control Activator (CAP) cAMP + Transcription + If there is glucose around cAMP level will be Glucose + low. cAMP – CAP (activator) will not bind Transcription - Efficiently. If there is no efficient activator, transcription will be lower 26 More recent studies have shown a different system where the presence of a specific molecule inhibits gene expression. These molecules are often the end products of biosynthetic pathways. For example, bacteria can make the amino acid tryptophan, but if there’s already enough tryptophan in the environment, it’s wasteful to produce more. 28 The Tryptophan (trp) Operon in E. coli Is a Repressible Gene System In this case, tryptophan itself helps block the transcription of mRNA needed for its production. Unlike the inducible system for lactose, this system is called repressible. Negative control like lactose system- there is repressor 29 30 31 Summary 1. Research on the lac operon in E. coli pioneered our understanding of gene regulation in bacteria. 2. Genes involved in the metabolism of lactose are coordinately regulated by a negative control system that responds to the presence or absence of lactose. 3. The catabolite-activating protein (CAP) exerts positive control over lac gene expression by interacting with RNA polymerase at the lac promoter and by responding to the levels of cyclic AMP in the bacterial cell. 4. Unlike the inducible lac operon, the trp operon is repressible. In the presence of tryptophan, the repressor binds to the regulatory region of the trp operon and represses transcription initiation. 32 Summary Negative-inducable Negative- Repressive 33 Transcriptional Regulation in Eukaryotes 34 35 While transcription and translation are tightly coupled in bacteria, in eukaryotes, these processes are spatially and temporally separated, and thus independently regulated. Gene expression is regulated at many steps in eukaryotes, including DNA packaging into chromatin, transcription, RNA processing, RNA export from the nucleus, RNA stability, RNA localization, translation, and posttranslational modifications. Chromatin remodeling, as well as modifications to DNA and histones, play important roles in regulating gene expression in eukaryotes. Eukaryotic transcription initiation requires the assembly of transcription regulatory proteins at cis-acting DNA sites known as promoters, enhancers, and silencers. Transcriptional activators and repressors influence the association of the general transcription factors into pre-initiation complexes at gene promoters, and they may influence chromatin remodeling or modifications. The genome-wide identification of transcription factor binding sites and chromatin modifications in the human genome in a manner that is cell-type specific has proven to be a powerful tool for understanding transcriptional regulation and how it relates to disease. 36 Unlike genetic changes (mutations), epigenetic changes are reversible and do not change the sequence of DNA bases, but they can change how your body reads a DNA sequence. 37 DNA methylation involves the attachment of small chemical groups called methyl groups (each consisting of one carbon atom and three hydrogen atoms) to DNA building blocks. When methyl groups are present on a gene, that gene is turned off or silenced, and no protein is produced from that gene. 38 39 40 Eukaryotic Gene Expression Is Influenced by Chromatin Modifications Open and Closed Chromatin Histone Modifications and Chromatin Remodeling DNA Methylation https://www.nature.com/articles/nrg887 41 Eukaryotic Transcription Initiation Requires Specific Cis-Acting Sites Promoters and Promoter Elements Enhancers, Insulators, and Silencers 42 43 Eukaryotic Transcription Initiation Is Regulated by Transcription Factors That Bind to Cis-Acting Sites 44 Gene expression is also regulated by mRNA stability and degradation However, two features of mRNAs provide protection against exoribonucleases: a 7-methylguanosine (m7G) cap at the 5′ end and a poly- A tail at the 3′ end. Maintenance or removal of the cap and poly-A tail are thus critical steps in determining the stability or decay of an mRNA. 45 Noncoding RNAs Play Diverse Roles in Posttranscriptional Regulation Eukaryotic genomes encode many long noncoding RNAs (lncRNAs), typically defined as being longer than 200 nucleotides. Unlike mRNAs, lncRNAs do not have start and stop codons and are not translated into proteins, although they undergo similar processing, including capping, polyadenylation, and splicing. The human genome is estimated to contain around 17,000 lncRNAs. lncRNAs play diverse regulatory roles, particularly in post-transcriptional processes. They can bind to chromatin-regulating complexes to influence gene expression and interact with transcription factors. lncRNAs can hybridize with mRNAs or pre-mRNAs, affecting alternative splicing by binding to splice sites, which can exclude exons from the final transcript. Additionally, lncRNA–mRNA hybridization can generate double-stranded RNA that triggers RNA interference (RNAi), leading to mRNA degradation via Dicer and RISC. Furthermore, lncRNAs can regulate mRNA stability, decay, and translation, showcasing their critical roles in gene regulation. 46 Gene regulation mechanisms by lncRNAs The major transcriptional and post-transcription gene regulation events influenced by lncRNAs. (1) LncRNAs control the binding of chromatin-modifying complexes to specific genomic loci and regulate gene expression at epigenetics level. (2) LncRNAs compete for binding with splicing regulatory proteins (e.g. SR) to modulate alternative splicing of pre-mRNAs. (3) LncRNAs alter the activity of transcription factors (TF) by acting as co-activators or repressors on the regulation of gene expression. ( (4) Formation of lncRNAs-mRNA hybrid protects mRNA from decay and increases mRNAs stability. (5) lncRNAs functions jointly with other proteins, e.g. Staufen1 to promote mRNA decay. (6) lncRNAs enhance ribosome binding to mRNA and thus promote translation. (7) lncRNAs recruit translation repressors, e.g. Rck, leading to translation repression. (8) Some lncRNAs product miRNAs for regulating gene expression. 47 Application of Long Noncoding RNAs in Osteosarcoma: Biomarkers and Therapeutic Targets July 2017 Summary 1. The regulation of gene expression in eukaryotes differs in several ways from that of bacteria. 2. Eukaryotic gene regulation at the chromatin level may involve gene-specific chromatin remodeling, histone modifications, or DNA modifications. 3. Eukaryotic transcription is regulated by cis-acting regulatory elements such as promoter, enhancer, and silencer elements. 4. Transcription factors influence transcription by binding to promoters, enhancers, and silencers. 5. Transcription factors regulate transcription by promoting or inhibiting the association of general transcription factors with the core promoter and by modifying chromatin structure. 49 54 https://www.pbslearningmedia.org/resource/tdc02.sci.life.cell.genecontrl/gene-control/ https://www.nature.com/scitable/topicpage/operons-and-prokaryotic-gene-regulation-992/ 55 https://www.youtube.com/watch?v=i9a-ru2ES6Y 56 https://www.youtube.com/watch?v=3S3ZOmleAj0 57 https://www.nature.com/articles/hdy201054 https://biomodal.com/blog/epigenetics-unlocking-the-secrets-of-gene-regulation/ https://www.youtube.com/watch?v=Gs3llepaaB0 https://www.youtube.com/embed/eYrQ0EhVCYA ** https://www.ncbi.nlm.nih.gov/books/NBK532999/ https://www.youtube.com/watch?v=h_1QLdtF8d0 58 https://www.youtube.com/watch?v=7kkkqlQEqHI 59 [email protected] 60