Fox Revision Notes PDF
Document Details
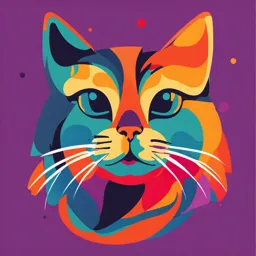
Uploaded by CheaperBlueLaceAgate
Warwick
Fox
Tags
Summary
These are revision notes on organic chemistry, focusing on carbonyl compounds and nucleophilic additions. The notes cover key concepts and examples, including the Felkin-Anh model and chelation control in 1,2 and 1,3 additions. Useful for undergraduate chemistry students.
Full Transcript
Carbonyl compounds are polar due to the difference in electronega6vity between carbon and oxygen, with oxygen being more electronega6ve. This gives carbonyl compounds "carbonyl polarity" (page 1). Due to this polarity, carbonyl compounds are suscep6ble to nucleophilic addi6on reac6ons. Nucleophiles a...
Carbonyl compounds are polar due to the difference in electronega6vity between carbon and oxygen, with oxygen being more electronega6ve. This gives carbonyl compounds "carbonyl polarity" (page 1). Due to this polarity, carbonyl compounds are suscep6ble to nucleophilic addi6on reac6ons. Nucleophiles are aCracted to the par6al posi6ve charge on the carbon atom. This allows compounds like alcohols, amines, hydroxides to react with carbonyl groups (page 2). The carbonyl group absorbs strongly in the infrared region of the electromagne6c spectrum between 1600-1800 cm-1. This carbonyl stretch makes infrared spectroscopy useful for iden6fying carbonyl compounds (page 3). Carbonyl compounds undergo nucleophilic addi6on reac6ons through a step-wise mechanism involving a tetrahedral intermediate. This allows the carbonyl group to take on unshared electron pairs from the nucleophile (pages 4-5). Common carbonyl compounds are discussed as examples, like aldehydes, ketones, esters, acid anhydrides, acid chlorides (pages 6-8). 1,2-directed addi6on key details: 1,2-directed addi6on occurs when a nucleophile adds to the carbon atom adjacent to the carbonyl carbon. This is directed by a subs6tuent on the carbonyl compound that can stabilize the developing nega6ve charge on the carbon being aCacked by the nucleophile. Examples given of direc6ng groups that can cause 1,2-directed addi6on are alkyl groups (R), phenyl (Ph), hydroxyl (OH), and amino (NH2) subs6tuents. The document provides a reac6on scheme to illustrate 1,2-directed addi6on of hydroxide to acetone, showing the hydroxyl group direc6ng the addi6on to the methyl carbon rather than the carbonyl carbon (page 5). It notes this type of addi6on is common for compounds with alpha hydrogens, like aldehydes and ketones with R groups on the carbonyl carbon. Background informa6on on the Felkin-Anh model: The Felkin-Anh model was developed in the 1960s-70s to explain and predict the stereoselec6vity of nucleophilic addi6ons to carbonyl compounds containing stereocenters. It is based on minimizing orbital overlap repulsions during the transi6on state for the addi6on. In the polar model: The incoming nucleophile and leaving group are posi6oned to minimize interac6ons between their lone pairs and the p-orbitals of the developing C-C bond. This posi6ons the nucleophile and leaving group in an equatorial orienta6on in the transi6on state. Results in an6-addi6on and reten6on of configura6on for aldehydes and ketones. In the non-polar model: There are no lone pair repulsions to consider since the nucleophile and leaving group are non-polar. The transi6on state orients the two largest subs6tuents in equatorial posi6ons to minimize steric strain. This leads to syn-addi6on and inversion of configura6on for aldehydes and ketones. So in summary, the Felkin-Anh models were able to ra6onalize and predict the stereoselec6vity of addi6ons based on minimizing either electronic or steric interac6ons in the transi6on state. Let me know if you need any clarifica6on or have addi6onal ques6ons! Chela6on control 1,2 addi6on and its requirements Chela6on control of 1,2-addi6on involves a carbonyl compound containing two coordina6ng atoms (usually oxygen, nitrogen, sulfur) that can bind to the metal atom of a metal salt/complex. This allows the metal atom to chelate (form rings) with both coordina6ng atoms simultaneously. Some key points about chela6on control of 1,2-addi6on: The carbonyl compound must have two coordina6ng atoms adjacent to each other to allow chela6on with a metal ion. Common examples are beta-keto esters and beta-diketones, which can chelate with their carbonyl and ester/ketone oxygen atoms. Chela6on rigidifies the transi6on state geometry and directs nucleophilic aCack to one face over the other. It directs 1,2-addi6on to occur on the side of the carbonyl group opposite to the metal chelate ring. This minimizes eclipsing interac6ons between subs6tuents. The metal ion (usually Zn, Mg, Al) forms a 6-membered ring chelate complex with the carbonyl compound that directs stereoselec6vity. The metal salt/complex must be soluble to allow complexa6on with the carbonyl substrate in solu6on before the reac6on occurs. It provides high levels of diastereocontrol, oden >20:1 selec6vity, by rigidly imposing one transi6on state geometry. 1,3 directed addi6on 1,3-directed addi6on to carbonyl compounds occurs when a nucleophile adds to the carbon atom located two bonds away from the carbonyl carbon. This is directed by a subs6tuent on the carbonyl compound that can stabilize the developing nega6ve charge on the 1,3-carbon being aCacked. Some key details about 1,3-directed addi6on: Direc6ng groups that can cause 1,3-addi6on are carboxylic acid deriva6ves (esters, acids, acid chlorides), since the carbonyl oxygen is able to delocalize the nega6ve charge. The reac6on follows a six-membered transi6on state analogous to 1,2-addi6on, with the direc6ng group stabilizing the developing nega6ve charge. An example reac6on would be addi6on of hydroxide to ethyl acetate. The ester oxygen directs OH addi6on to the methyl carbon rather than the carbonyl carbon. 1,3-Addi6on is common for beta-keto esters and other carbonyl compounds with electron-withdrawing subs6tuents on the beta carbon. It proceeds through a stepwise mechanism with a tetrahedral intermediate as in 1,2addi6on. The direc6ng group influences both the regiochemistry (1,3- vs 1,2-addi6on) and stereochemistry (syn vs an6) of the reac6on. 1,3-directed reduc6ons 1,3-directed reduc6ons of carbonyl compounds follow similar principles to 1,3-directed nucleophilic addi6ons, with the key difference being the use of a reducing agent rather than a nucleophile. Some specific points about 1,3-directed reduc6ons: Common reducing agents used are lithium aluminum hydride (LAH) and sodium borohydride (NaBH4). As with addi6ons, the carbonyl compound must have an electron-withdrawing subs6tuent (EWG) that can stabilize the developing nega6ve charge on the 1,3carbon. Examples of EWGs that direct 1,3-reduc6on are esters, nitriles, amides, sulfonates. The carbonyl oxygen or nitrogen delocalizes the charge. The reac6on proceeds via ini6al aCack of hydride at the carbonyl carbon. This is followed by 1,3-migra6on of the EWG and hydride to the 1,3-carbon. This results in stereospecific reduc6on of the 1,3-carbon while retaining stereochemistry at other centers. It is a useful method to reduce carbonyl compounds regioselec6vely in the presence of other carbonyls. Condi6ons must be controlled to avoid over-reduc6on by the hydride source. 1,3-Reduc6ons are widely used in synthesis to install stereocenters with high regioand stereoselec6vity. For hydrobora6on (pages 1-3): It involves the addi6on of BH3 to an alkene to form an organoborane intermediate. Stereochemistry of the alkene is retained in the product ader hydrobora6on. Hydrobora6on is highly regioselec6ve, favoring an6-Markovnikov addi6on across the C=C. Oxida6on or hydrolysis of the organoborane converts it to the corresponding alcohol. Mild condi6ons and high regioselec6vity make it useful for func6onal group interconversion. For cyclopropana6on (pages 4-6): It involves the carbene addi6on across a C=C to form a 3-membered ring. The Simmons-Smith reac6on uses diiodomethane (CH2I2) as the carbene source. Diazoalkanes (R-N=N+) are another common carbene precursor. Stereochemistry of the alkene is generally retained in the cyclopropane product. It is highly regioselec6ve for the more subs6tuted alkene carbon. Cyclopropanes are useful synthe6c intermediates that can be opened or func6onalized. Here are some more details about the hydrobora6on reac6on: Hydrobora6on involves the stereospecific addi6on of BH3 to the pi bond of an alkene (page 1). It forms an organoborane intermediate that has the boron atom bonded to the two carbons of the original alkene (page 1). The reac6on is highly regioselec6ve, favoring an6-Markovnikov addi6on. BH3 adds to the less subs6tuted alkene carbon (page 1). Oxida6on or hydrolysis of the organoborane converts it to the corresponding alcohol. Oxida6on with H2O2/NaOH gives the an6-Markovnikov alcohol (page 2). Hydrobora6on-oxida6on allows the conversion of alkenes to alcohols while retaining stereochemistry and regioselec6vely forming the an6-Markovnikov product (page 2). Mild condi6ons and high selec6vity make it useful for func6onal group interconversion in organic synthesis (page 3). The mechanism involves ini6al forma6on of a pentacoordinate boron transi6on state leading to stereospecific addi6on (page 3). 9BBN is more selec6ve for some major isomer of product 1. >95:99:95% regioisomeric control, adding predominantly to one face of the alkene. BH3 may give lower, >85% selec6vity. 2. In some cases, 9-BBN can give nearly exclusive (>99%:85% of the major isomer. The 15% minor isomer forms from a less stable but s6ll somewhat feasible transi6on state. In contrast, 9-BBN's bulky bicyclic ring rigidly enforces one transi6on state conforma6on over others. This minimizes destabilizing eclipsed interac6ons between subs6tuents to an even greater degree. When subs6tu6on differences on the alkene are small, 9-BBN can s6ll give >95% selec6vity. But when the differences are large, it effec6vely restricts the reac6on solely to the most stable transi6on state geometry. This extremely restricted, lowest energy transi6on state allowed by 9-BBN's structure results in selec6vity ra6os approaching 100%, like >99%: 90% in many cases). Note on the use of amine protec6ng groups as chiral templates: Amines are commonly used as nucleophiles in organic reac6ons, but must be protected to avoid side reac6ons. Chiral amine protec6ng groups can serve as templates to induce and control chirality during reac6ons of protected amines (page 6). Examples given include N-diphenylphosphinoyl (DPP) and Ndiphenylphosphoramidate (DPPA) protec6ng groups. These groups are bulky and introduce both steric......... effects that can orient the substrate in the transi6on state to favor one enan6omer. For example, DPP-protected amines par6cipate in highly enan6oselec6ve Strecker reac6ons through template control by the DPP group (page 7 reac6on scheme). The chirality of the amine protec6ng group template is transferred to the new stereocenter with high enan6oselec6vity (ee > 90% in many cases). The Evans auxiliary is a useful chiral auxiliary for controlling stereochemistry in reac6ons of alkenes. Some key points: The Evans auxiliary is a chiral sulfinyl group (-S(O)R) that can be aCached to an alkene (pages 2-3). It directs incoming nucleophiles or electrophiles to aCack from the less hindered siface of the alkene via the gauche effect. This allows highly diastereoselec6ve addi6ons across the alkene, such as hydrobora6on, with de >95% (page 3 example). Ader the addi6on, the sulfinyl auxiliary can be removed without racemiza6on, providing access to enan6oenriched alcohols, amines, etc. The Evans auxiliary is par6cularly useful for controlling stereochemistry in reac6ons of trisubs6tuted alkenes, which would otherwise occur with liCle selec6vity. It introduces chirality and steric bulk to direct asymmetric induc6on in these challenging substrates. Here are some notes on using the Evans auxiliary to control stereochemistry in alkyla6on reac6ons of alkenes: Alkyla6ons, like hydrobora6on, proceed by addi6on across the alkene double bond. In an alkyla6on, the nucleophile is an alkyl/organometallic reagent rather than hydride. Common alkyla6ng agents discussed include Grignard reagents, organolithium/copper reagents, and silyl/boron reagents (page 4). As in hydrobora6on, the Evans auxiliary directs incoming alkyl groups to aCack from the less hindered si-face of the alkene (page 4). This allows for highly diastereoselec6ve alkyla6ons, forming up to 4 new stereocenters in highly......... fashion (page 5 examples). The auxiliary can then be removed, providing enan6oenriched alkyla6on products useful for synthesis. Alkyla6ons are par6cularly challenging without chiral control, so the Evans auxiliary is very useful for this class of reac6ons. Here are some notes on using chiral auxiliaries to control selec6ve enolate forma6on: Enolates are important nucleophilic intermediates in organic synthesis, but can form non-selec6vely from prochiral ketones. Chiral auxiliaries like the TMS (trimethylsilyl) ketene acetal and MOM (methoxymethyl) ketene acetal can be aCached to control enolate forma6on (page 8). These auxiliaries chelate to the carbonyl oxygen, rigidifying the transi6on state and favoring forma6on of only one enolate diastereomer. For example, the (R)-TMS auxiliary leads to >95% de of the (Z)-enolate (page 8, top reac6on). Highly diastereoselec6ve alkyla6ons, aldol reac6ons, etc. can then be performed on the controlled enolate intermediate (page 8). Ader reac6ons, the auxiliary can be removed without racemiza6on, providing enan6oenriched products. This method of chiral auxiliary-controlled enolate forma6on expands the scope of carbonyl chemistry. Here are some notes on using chiral auxiliaries to control aldol reac6ons: Aldol reac6ons between carbonyl compounds generate new stereocenters, but can proceed with low diastereoselec6vity. Chiral auxiliaries aCached to one carbonyl reactant can induce asymmetric induc6on by controlling the stereochemistry of enolate forma6on (page 9). For example, the Evans oxazolidinone auxiliary directs the an6-aldol addi6on in reac6ons with aldehydes (page 9, top). The TMS ketene acetal auxiliary also controls an6-selec6ve aldol addi6ons with aldehydes when aCached to the ketone (page 9, boCom). High diastereoselec6vity is achieved, oden >20:1 ra6o of an6- to syn-aldol products. The auxiliary can then be removed, providing useful aldol substrates for further func6onaliza6on or synthesis. This chiral auxiliary control of aldol reac6ons is very useful for genera6ng mul6ple stereocenters in a predictable manner. Here are more detailed notes on the hydrobora6on of chiral alkenes from pages 1-3 of the document: The hydrobora6on reac6on proceeds with high stereoselec6vity, retaining the configura6on of the original alkene (p. 1) BH3 adds to the less hindered, less subs6tuted alkene carbon in an an6-Markovnikov fashion (p. 1) Oxida6on of the organoborane intermediate with NaOH/H2O2 occurs with stereochemical reten6on, providing the an6 alcohol (p. 2) With BH3, typical regioselec6vity is 80:20 and diastereoselec6vity is 93:7 for disubs6tuted alkenes (p. 2) 9-BBN is more sterically hindered and gives improved selec6vity, such as 95:5 regioselec6vity and 99:1 diastereoselec6vity (p. 3) The mechanism involves a transi6on state that maintains alkene stereochemistry during boron addi6on (p. 3) Here are some addi6onal mechanis6c details about the hydrobora6on reac6on based on pages 1-3 of the document: Hydrobora6on involves the stereospecific addi6on of BH3 or 9-BBN (9borabicyclo[3.3.1]nonane) to the π bond of the alkene (p. 1) It forms an organoborane intermediate where the boron atom is bonded to the two original alkene carbons in an an6-Markovnikov fashion (p. 1) The reac6on is believed to proceed through a cyclic transi6on state involving overlap of the empty p-orbital on boron with the HOMO of the alkene π-bond (p. 2) This cyclic transi6on state maintains the stereochemistry of the original alkene during boron addi6on, resul6ng in high stereoselec6vity (p. 2) Oxida6on of the organoborane with oxidizing agents like NaOH/H2O2 or H2O2/tetrabutylammonium fluoride regenerates the alkene and forms the an6Markovnikov alcohol product (p. 2) 9-BBN is more sterically hindered and forces the transi6on state to be even more chair-like, improving selec6vity compared to BH3 (p. 3) Here are some addi6onal details on the mechanism of the hydrobora6on reac6on: The reac6on is believed to proceed through a cyclic transi6on state involving overlap of the empty p-orbital on boron with the HOMO of the alkene π-bond (p. 2) This forms a 6-membered chair-like cyclic transi6on state that maintains the alkene stereochemistry during boron addi6on (p. 2) In the transi6on state, the boron atom is posi6oned equatorially while the two alkene carbons are in axial posi6ons (p. 2) This chair-like arrangement minimizes eclipsing interac6ons between the large alkene subs6tuents, lowering the transi6on state energy and favoring the an6-Markovnikov product (p. 2) 9-BBN is bulkier than BH3, which forces the transi6on state to be even more chairlike through increased A1,3-strain if the boron added to the subs6tuted alkene carbon (p. 3) This steric effect strongly favors the transi6on state where boron adds to the less subs6tuted alkene carbon, enhancing regio- and diastereoselec6vity (p. 3) Oxida6on then converts the organoborane intermediate back to the alkene, retaining the stereochemistry set in the ini6al transi6on state (p. 2) Here are more detailed notes on C=C cyclopropana6on reac6ons: Using CH2I2 (Simmons-Smith reac6on) or diazoalkanes generates a carbene that adds across the C=C via a [2+1] cycloaddi6on (p. 4) The reac6on occurs with high diastereoselec6vity, favoring the cis-cyclopropane product. This suggests a chair-like transi6on state maintaining alkene Z/E stereochemistry (p. 4) AlMe3 is oden used as a catalyst, possibly forming a π-complex that orients the alkene for backside carbene aCack (p. 4) Osmium tetroxide (OsO4) dihydroxylates the alkene in a [2+2] cycloaddi6on to form a cyclic osmate ester (p. 5) Workup with sodium periodate or hydrogen peroxide cleaves the osmate ester to give the vicinal diol stereospecifically (p. 5) m-Chloroperoxybenzoic acid (MCPBA) epoxidizes alkenes in a similar [2+2] cycloaddi6on. Cleavage of the epoxide gives iden6cal diastereoselec6vity to OsO4 (p. 5) A chiral template is a temporarily aCached chiral auxiliary that controls the stereoselec6vity of a reac6on at a pro-chiral or achiral center (p. 6) Common templates include bulky ligands, protec6ng groups, or ligands that form complexes with metal catalysts (p. 6) The template binds to the pro-chiral substrate and forces the transi6on state to favor approach of reactants from one face through steric/electronic effects (p. 6) This can yield greater than 95% selec6vity in reac6ons like alkyla6ons (p. 6) Ader the reac6on, the template is removed without racemiza6on. Since the ini6al selec6vity was high, the product will also have high enan6omeric excess (ee) (p. 6) One drawback is templates some6mes require harsh condi6ons for removal, destroying the product (p. 6) A beCer template would be easily removed under mild condi6ons while maintaining the stereochemistry of any byproducts, allowing them to be recycled (p. 6) Here are some addi6onal details that could be expanded on regarding chiral templates: Common protec6ng groups used as templates include CBZ (benzyloxycarbonyl) and BOM (benzyloxymethyl) groups for amines (p. 6) These are bulky and can direct alkyla6ons/acyla6ons of amines to one face, while s6ll being removable under mild condi6ons (hydrogenolysis) (p. 6) Chiral ligands also serve as excellent templates, forming complexes with metal catalysts that orient substrates in an enan6oface selec6ve manner (p. 6) Examples include BINAP, DIOP, SEGPHOS ligands used for asymmetric hydrogena6ons, alkyla6ons etc. (p. 6) The strength of interac6on between the template and substrate, as well as any subs6tuents that can induce further asymmetric induc6on, influence the degree of enan6oselec6vity achieved (p. 6) For example, electron-withdrawing groups increase the ligand-substrate complex stability for higher ee (p. 6) An ideal template would be easily aCached and removed, recyclable, and impart very high enan6oselec6vity through a rigid, well-defined transi6on state (p. 6) Evans auxiliary: It is derived from an alpha-amino acid, allowing single enan6omers from natural sources (p. 7) Ader deprotona6on, the sodium coordinates to the carbonyl and carboxylate oxygens, keeping the enolate flat (p. 7) This rigid, chiral chelate directs approach of electrophiles to the Re-face through steric hindrance (p. 7) Only the Z-enolate geometry is stabilized by sodium chela6on. The E-form would place the large subs6tuents in a destabilizing 1,3-diaxial interac6on (p. 7) In the transi6on state, having the methyl group axial minimizes steric clash with the auxiliary (as you noted, due to the slightly flaCer, more distorted chelate complex allowing more room) (p. 7) This results in excellent diastereocontrol, oden >20:1 de for alkyla6ons (p. 7) How the SM aldol auxiliary works. Here are a few more details: The sulfonamide carbonyl and ester carbonyl arrange in an an6parallel orienta6on due to opposing dipoles (p. 8) A weaker base like LDA is used to deprotonate selec6vely the α-hydrogen, avoiding auxiliary deprotona6on (p. 8) The triflate (beCer leaving group than tosylate) is then displaced, forming the Zenolate (p. 8) The methyl subs6tuent prefers the posi6on distal to the bulky auxiliary group to minimize steric clashes (p. 8) Boron chelates to the incoming aldehyde through a 6-membered cyclic transi6on state rather than involving the auxiliary (p. 8) This results in excellent diastereoselec6vity, oden >20:1 de for aldol addi6ons (p. 8) The aldehyde approaches from the least hindered si-face directed by the auxiliaryenolate chelate (p. 8) Here is a full explana6on of how chiral auxiliaries work to control stereochemistry: Chiral auxiliaries are temporarily aCached func6onal groups that induce chirality and control stereoselec6vity in organic reac6ons. They work by forming rigid, chiral complexes with reac6ve intermediates that direct approaching reagents. Common types of auxiliaries include sulfonamides, TADDOL deriva6ves, and protec6ng groups on amines or alcohols. They are chosen based on the substrate and desired transforma6on. The auxiliary aCaches to the pro-chiral or achiral center prior to the key bond-forming step. It then chelates to or otherwise complexes with the reac6ve intermediate. For enolates, the auxiliary oden chelates to the alkoxide oxygen and carbonyl group to form a flat, rigid Z-enolate. This fixes the substrate in a chiral pocket that blocks one face while exposing the other. For example, the Evans auxiliary forms a 6-membered chelate complex with sodium that directs alkyla6ons to proceed from the top Re face with excellent diastereoselec6vity. In aldol reac6ons, the SM auxiliary arranges with opposing carbonyl dipoles and its boron chelate directs hydride addi6on from the least hindered si face. Once the new stereocenter is set, the auxiliary is removed without racemiza6on. This allows access to enan6oenriched products from pro-chiral or meso star6ng materials. In summary, chiral auxiliaries induce stereoselec6vity by forming rigid complexes that block one reac6ve face of intermediates while direc6ng approaching reagents to the other face. This powerful approach enables high levels of stereocontrol in synthesis.