Forces of Attraction - CAPE Chemistry Unit 1 - PDF
Document Details
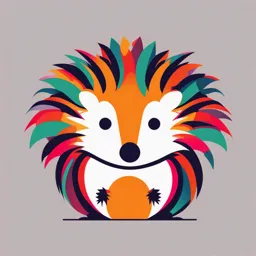
Uploaded by ReceptiveBigfoot1289
Titchfield High School
CAPE
Ms Lawrence
Tags
Related
- Noncovalent Interactions in Biomolecules PDF
- Interparticle Forces of Attraction PDF
- Chemistry 101: Intermolecular Forces of Attraction PDF
- Intermolecular Forces of Attraction - Week 3, Makati High School
- Intermolecular Forces of Attraction (ORGCHEM 2024) Lecture Notes PDF
- Week 2 Forces of Attraction - Our Lady of Fatima University - PDF
Summary
This document is a presentation on forces of attraction in chemistry. It details different types of forces, including intramolecular and intermolecular forces, and describes concepts such as electronegativity and how it affects bonding type (covalent, ionic, metallic).
Full Transcript
FORCES OF ATTRACTION CAPE CHEMISTRY UNIT 1 MS LAWRENCE FORCES OF ATTRACTION The forces of attraction are the forces that exist within molecules or between molecules. These are described as intramolecular and intermolecular forces of attraction. Intramolecul...
FORCES OF ATTRACTION CAPE CHEMISTRY UNIT 1 MS LAWRENCE FORCES OF ATTRACTION The forces of attraction are the forces that exist within molecules or between molecules. These are described as intramolecular and intermolecular forces of attraction. Intramolecular forces are forces of attraction within molecules. These forces are strong and are as a result of chemical bonds. Intermolecular forces are forces of attraction between molecules and are weak forces. FORCES OF ATTRACTION The physical state of matter depends on the strength of the forces of attraction in and between the particles. Solids have strong forces of attraction while liquids have moderate forces of attraction and gases have weak forces of attraction. Physical properties such as boiling point, melting point and solubility depend on the type of forces of attraction present in compounds. FORCES OF ATTRACTION ELECTRONEGATIVITY Electronegativity is a measure of how strongly an atom pulls on electrons when it forms a bond with another atom. The stronger the “pull,” the more the atom attracts electrons towards itself in a bond. Trends in the Periodic Table: Across a Period (left to right): Electronegativity increases. Atoms on the right side (like oxygen or fluorine) have a stronger pull because they want to complete their outer shells. These atoms require few electrons to complete their valence shells and have few inner electron shells between nucleus and valence electrons. These atoms are the most electronegative.. FORCES OF ATTRACTION Trends in the Periodic Table: Down a Group (top to bottom): Electronegativity decreases. Atoms get larger, so their outer electrons are farther from the nucleus, reducing their pulling power. High vs. Low Electronegativity: Atoms with high electronegativity (like fluorine, oxygen, and nitrogen) pull electrons strongly. Atoms with low electronegativity (like metals) don’t pull as hard and may even lose electrons easily. FORCES OF ATTRACTION ELECTRONEGATIVITY The concept of electronegativity was first introduced by Linus Pauling, and it often represented on a scale from 0 to 4, with fluorine being the most electronegative element, its electronegativity is 4.0. Metals have electronegativity less than 2.0. The least electronegative elements are cesium (Cs) and francium (Fr), with electronegativity values of 0.7. Therefore, Fluorine is the most electronegative element, and cesium is the least electronegative element. FORCES OF ATTRACTION Why Electronegativity Matters: Electronegativity helps us understand how electrons are shared in bonds. How?? Since electronegativity tells us how strongly an atom pulls on shared electrons, it also determines how evenly or unevenly those electrons are shared in a bond. When two atoms form a bond, their electronegativity difference decides if the bond is polar or nonpolar. Equal pull between atoms (similar electronegativity) creates a non-polar bond. Unequal pull (different electronegativity) creates a polar bond, with electrons being closer to the atom with higher electronegativity. FORCES OF ATTRACTION Polar Bonds (Unequal Sharing): If one atom has a higher electronegativity than the other, it pulls the electrons closer to itself, causing unequal sharing. This creates a polar bond, where the atom with higher electronegativity gets a slight negative charge (because it has more electron density around it), while the other atom gets a slight positive charge. Example: In H₂O (water), oxygen has a higher electronegativity than hydrogen, so it pulls the electrons closer to itself, making oxygen slightly negative and hydrogen slightly positive. FORCES OF ATTRACTION Nonpolar Bonds (Equal Sharing): If the two atoms have similar or equal electronegativity, they pull on the electrons with nearly the same strength. As a result, the electrons are shared equally between them, and the bond is nonpolar. Example: In an H₂ molecule, both hydrogen atoms have the same electronegativity, so they share electrons equally. INTRAMOLECULAR FORCES Covalent, Ionic & Metallic COVALENT BONDING A covalent bond consists of the simultaneous attraction of two nuclei for one or more pairs of electrons. In other words, a covalent bond is formed when two atoms share one or more electron pairs. Based on the number of pairs of shared electrons, the covalent bonds formed can be classified into three: Single bond: one pair of electrons is shared Double bond: share two pairs of electrons Triple bond: share three pairs of electrons COVALENT BONDING When atoms combine to form a covalent molecule, their atomic orbitals combine or overlap to form a covalent bond. Molecular orbital When atomic orbitals overlap and combine, molecular orbitals are formed. Depending on the nature of the overlap, molecular orbitals may be sigma molecular orbitals or pi molecular orbitals. Sigma molecular orbitals produce sigma bonds while pi molecular orbitals produce pi bond. SIGMA BONDS When two atomic orbitals overlap linearly along the axis, the bond formed is called a sigma (σ) bond. The orbital overlap is always along the axis of the nuclei so that the bond is centered directly between the two nuclei. 1. The first possibility is the overlap of the two s orbitals. ss overlapping examples: In forming a sigma bond between two hydrogen atoms (H₂), each hydrogen atom contributes its 1s orbital for the sigma bond. SIGMA BONDS SIGMA BONDS 2.The second possibility is the overlap of an s and a p orbital Sigma bonds can form between an s orbital and a p orbital. Example: In forming a sigma bond between hydrogen and chlorine in HCl, the hydrogen’s 1s orbital overlaps with the chlorine’s 3p orbital. SIGMA BONDS 3. The third possibility is the end-on overlap of two p orbitals Sigma bonds can form between two p orbitals. Example: In forming a sigma bond between two carbon atoms in ethane (C₂H₆), the two carbon atoms contribute sp³ hybridised orbitals for sigma bond formation PI (Π) BOND Pi bonds are formed by the side-to-side overlap of parallel p orbitals that are perpendicular to the internuclear axis. Pi bonds are typically found in double bonds (one sigma, one pi) and triple bonds (one sigma, two pi). Pi bonds are weaker than sigma bonds. Representation: In a Lewis structure, a double bond (σ + π) is represented by a double line (e.g., C₂H₄: C=C), and a triple bond (σ + 2π) is represented by a triple line (e.g., C₂H₂: C≡C) Electronegativity and Polarity: In covalent bonds, the degree of polarity depends on the electronegativity difference between the atoms. If the difference is significant (0.4–1.7), the bond is polar covalent, where electrons are shared unequally. A small or zero difference in electronegativity (0–0.4) results in a nonpolar covalent bond with equal electron sharing IONIC BONDING Ionic bonds form when there is a large difference in electronegativity between the two atoms (generally above 1.7 on the Pauling scale). I.e. Greater difference in electronegativity values between the atoms indicates a higher likelihood of ionic bonding. The higher the electronegativity difference, the more likely it is that one atom will completely transfer its electrons to the other, resulting in a highly polar bond. Ionic compounds are, therefore, polar by nature. IONIC BONDING An ionic bond is a chemical bond that is formed when one or more electrons are transferred from one atom to another atom, resulting in the formation of oppositely-charged ions. The positive ion (cation) is typically a metal, and the negative ion (anion) is usually a non-metal. These oppositely-charged ions are attracted to each other and form an ionic bond due to the electrostatic force between them. Electron Transfer Process IONIC BONDING During the formation of an ionic bond, atoms give and receive electrons to obtain a stable electron configuration, generally achieving a full outer shell of electrons (the octet rule). The metal atom loses one or more electrons, thus becoming a positively charged cation, whereas the non-metal atom gains those electrons, becoming a negatively charged anion. This electron transfer leads to a stable bond since the electrostatic attraction between the oppositely-charged ions holds them together. Examples of common ionic compounds include sodium chloride (NaCl), potassium iodide (KI), and magnesium oxide (MgO). In sodium chloride, sodium has a low electronegativity and loses and electron, while chlorine has a high electronegativity and gains an electron. Sodium chloride (NaCl): Sodium (Na) has one electron in its outer shell, while chlorine (Cl) has seven electrons in its outer shell. Sodium loses one electron to chlorine, forming a positively charged sodium ion (Na⁺) and a negatively charged chloride ion (Cl⁻). IONIC BONDING The electrostatic attraction between the ions results in the stable ionic compound NaCl. IONIC BONDING Potassium iodide (KI): Potassium (K) has one electron in its outer shell, while iodine (I) has seven electrons in its outer shell. Potassium loses one electron to iodine, forming a positively charged potassium ion (K⁺) and a negatively charged iodide ion (I⁻). The electrostatic attraction between the ions forms the stable ionic compound KI. IONIC BONDING Magnesium oxide (MgO): Magnesium (Mg) has two electrons in its outer shell, while oxygen (O) has six electrons in its outer shell. Magnesium loses its two electrons to oxygen, forming a positively charged magnesium ion (Mg²⁺) and a negatively charged oxide ion (O²⁻). The electrostatic attraction between the ions results in the stable ionic compound MgO. METALLIC BONDING Metal atoms usually have 1, 2, or 3 electrons in their outer shell (valence shell). These electrons are easily lost, which makes metals stable and gives them a tendency to lose electrons, making them electropositive. When a metal loses these electrons, they become "free electrons." METALLIC BONDING In metallic bonding, metal atoms come together and release their valence electrons, creating a “sea” of free-moving electrons shared by all the metal atoms. The metal atoms that lost electrons become positively charged ions (cations). These cations are arranged in a lattice, or organized structure, while the sea of electrons flows freely around them. METALLIC BONDING Though the positive ions repel each other, the attraction between the cations and the free electrons creates a strong, stable bond that holds the metal structure together. This shared electron "sea" also gives metals their unique properties, like the ability to conduct electricity and be shaped easily. METALLIC BONDING The electrons freely move within these molecular outermost shells, and therefore each electron becomes detached from its parent atom. The electrons are said to be delocalized. The metal is held together by the strong forces of attraction between the positive nuclei and the delocalized electrons METALLIC BONDING Consider the formation of metallic magnesium, when nine atoms combine, there will be eighteen electrons donated to the sea of electrons METALLIC BONDING Consider the joining of nine Aluminum atoms, there will be twenty- seven electrons donated to the sea METALLIC BONDING Generally, the more electrons donated towards the sea of electrons, the stronger the metallic bond. Also, the smaller the cations, the more tightly they are packed in the lattice of cations, so increasing the strength of the metallic bonds. Aluminium is therefore expected to have a higher melting point and is stronger than magnesium METALLIC BONDING Metallic bonds don't show polarity like ionic or covalent bonds because the electrons are delocalized—they aren’t shared or transferred between specific atoms. Metals have low electronegativity, meaning they easily lose electrons to create a cloud of electrons that move freely around the positively charged metal ions. This creates stability without any directional polarity. CO-ORDINATE OR DATIVE COVALENT BONDING In normal covalent bonding each atom donates one electron to the covalent bond. However, in some cases it is possible for one atom to donate both electrons to create the covalent bond. When a covalent bond is formed by the donation of both electrons from one atom, a dative or co-ordinate covalent bond is formed. In order for a dative bond to form an atom must be present with at least one lone pair of electrons that can be donated easily and there must be another atom with available space in its orbital to accommodate the electron pair. CO-ORDINATE OR DATIVE COVALENT BONDING Consider the following molecules: 1. BF3 Molecule The boron of BF3 has only six valence electrons and requires two more electrons to satisfy the octet rule. CO-ORDINATE OR DATIVE COVALENT BONDING 2. NH3 Nitrogen has a lone pair of electrons which can be donated easily. CO-ORDINATE OR DATIVE COVALENT BONDING When BF3 and NH3 combine, a new compound with the formula NH3:BF3 is produced. Here the nitrogen atom donates its lone pair of electrons to the B atom completing its octet. CO-ORDINATE OR DATIVE COVALENT BONDING Similarly, when ammonia acts as a base by accepting a proton, it uses its lone pair to form a covalent bond with H+. INTERMOLECULAR FORCES -Van der Waals forces -Permanent dipole – dipole forces -Hydrogen Bonding INTERMOLECULAR FORCES Molecules contain weaker intermolecular forces which are forces between the molecules There are three types of intermolecular forces: Induced dipole – dipole forces also called van der Waals or London dispersion forces Permanent dipole – dipole forces are the attractive forces between two neighbouring molecules with a permanent dipole Hydrogen Bonding are a special type of permanent dipole - permanent dipole forces VAN DER WAALS FORCES Van der Waals also called Induced dipole - dipole forces are the weakest type of intermolecular forces, occurring between non-polar atoms or molecules. These forces arise from temporary shifts in the distribution of the electron cloud within an atom or molecule. Normally, the electron cloud in a non-polar atom or molecule is evenly spread, but because electron charge cloud in non-polar molecules or atoms are constantly moving, there are moments when the cloud becomes more concentrated on one side. This creates a temporary dipole—a brief separation of positive and negative charges. This temporary dipole can induce a similar dipole in a nearby/ neighbouring atom or molecule. VAN DER WAALS FORCES When this happens, the δ+ end of the dipole in one molecule and the δ- end of the dipole in a neighbouring molecule are attracted towards each other. Because the electron clouds are moving constantly, the dipoles are only temporary VAN DER WAALS FORCES This temporary dipole can change quickly. One moment, the dipole might be on one side of the atom, and a moment later, it could shift to the other side. This means that there isn’t a fixed positive or negative side like there is in molecules that are permanently polar. Since these dipoles are only temporary and keep changing, there is no lasting or permanent dipole in the atom or molecule. However, even though these dipoles shift, the attractions between the temporary dipoles of different molecules still exist, allowing Van der Waals forces to occur. VAN DER WAALS FORCES An important trend occurs as the number of electrons in a system increases: the size of the temporary partial charges also increases, leading to stronger attractions between molecules. This Causes Changes in States of Matter The stronger Van der Waals forces can change the state of a substance. For example, gases can turn into liquids and liquids into solids as these forces get stronger. This phenomenon is evident in the halogens of Group VII in the Periodic Table, where there is a transition from gas (Cl₂) to liquid (Br₂) to solid (I₂) as Van der Waals forces strengthen due to increasing electron count. VAN DER WAALS FORCES This increase in attraction is crucial for understanding the conversion of gases into liquids and liquids into solids during changes in state. Chlorine (Cl₂) has fewer electrons, so its Van der Waals forces are weak, and it stays as a gas. Bromine (Br₂) has more electrons than chlorine, so its Van der Waals forces are a bit stronger, making it a liquid. Iodine (I₂) has the most electrons, so it has the strongest Van der Waals forces among these three, making it a solid. PERMANENT DIPOLE ATTRACTIONS Permanent dipole attractions exist between polar molecules. Polar molecules are those in which the atoms in the covalent bond differ in electronegativity. A permanent dipole occurs in these molecules due to an uneven distribution of electron density. This happens when one atom is more electronegative than the other, causing a partial negative charge on one end and a partial positive charge on the other. The attraction between the positive end of one polar molecule and the negative end of another is a stronger intermolecular force compared to Van der Waals forces. PERMANENT DIPOLE ATTRACTIONS Note: The molecule will always have a negatively and positively charged end. Forces between two molecules with permanent dipoles are called permanent dipole-dipole forces. The δ+ (delta positive) end of the dipole in one molecule and the δ- (delta negative) end of the dipole in a neighboring molecule are attracted to each other. PERMANENT DIPOLE ATTRACTIONS HYDROGEN BONDING Hydrogen bonding is the strongest form of intermolecular bonding that occurs between molecules. Hydrogen bonding is a type of permanent dipole – permanent dipole bonding. Unlike other atoms, hydrogen has no inner electrons, which means there’s less electron repulsion when it interacts with the non-bonding (lone) electron pairs on nearby electronegative atoms. This lack of inner electron shielding makes hydrogen bonds stronger than typical dipole-dipole attractions. HYDROGEN BONDING Hydrogen bonding occurs when a hydrogen atom is covalently bonded to one of the highly electronegative atoms: fluorine (F), oxygen (O), or nitrogen (N). When hydrogen is covalently bonded to an O, N or F, the bond becomes highly polarized. The H becomes so δ+ (partially positively charged) charged that it can form a bond with the lone pair of an O, N or F atom in neighboring molecule. For example, in water, each oxygen atom has two lone pairs, allowing water molecules to form two hydrogen bonds. HYDROGEN BONDING In water (H₂O), each hydrogen atom of one water molecule forms a hydrogen bond with the oxygen atom of a nearby water molecule. Each water molecule can form four hydrogen bonds with surrounding water molecules. This is because each water molecule has two lone pairs and two polar hydrogens. The ratio of lone pairs to polar hydrogens is ideal for all of the lone pairs and polar hydrogens to form hydrogen bonds. HYDROGEN BONDING In ammonia (NH₃), the hydrogen atoms in one molecule can form hydrogen bonds with the nitrogen atoms in neighboring ammonia molecules. In the case of ammonia, each ammonia molecule has one lone pair and three polar hydrogens. The ratio of lone pairs to polar hydrogens limits the amount of hydrogen bonds that can be formed. This means that each ammonia molecule can form only two hydrogen bonds with surrounding ammonia molecules. One hydrogen bond can be formed with the lone pair and one can be formed with only one of the polar hydrogens. This accounts for why water has a higher boiling point than ammonia. HYDROGEN BONDING Hydrogen bonding plays a crucial role in the unique properties of water and ice. These bonds lead to unexpectedly high melting and boiling points for water. While a typical polar molecule would have a boiling point around -100°C, water boils at 100°C due to strong hydrogen bonds between its molecules. This significant energy requirement to break these bonds allows water to remain liquid under normal atmospheric conditions, enabling the existence of oceans, lakes, and rivers. HYDROGEN BONDING The effects of hydrogen bonding extend to water's high surface tension and viscosity. This high surface tension enables small objects, such as razor blades and insects like water striders, to float on water's surface. In plants, hydrogen bonding facilitates capillary action, allowing water to travel through xylem vessels in roots and stems. Moreover, water has a high specific heat capacity, requiring substantial energy to raise its temperature. This property means that oceans heat up slowly in summer and cool down slowly in winter, helping to stabilize the Earth's surface temperature. HYDROGEN BONDING Research Question: "Investigate how the molecular structure of ice, influenced by hydrogen bonding, affects its density and behavior in water bodies. In your research, consider the following: 1. What specific structural characteristics of ice contribute to its lower density compared to liquid water? 2. How does this property of ice impact ecosystems, particularly during winter months in lakes and ponds? 3. Can you find examples of how this phenomenon affects aquatic life?