Fishpsych Lips PDF - Biological Perspective
Document Details
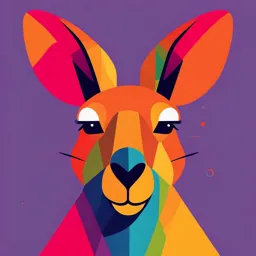
Uploaded by ResplendentSerpentine6285
Tags
Related
- Biological Foundations of Behavior PDF
- Nervous System - Biological Psychology - Neurons PDF
- PSYC100: The Biological Psychology Fall 20224 PDF
- Biological Aspects of Psychology PDF
- FPSH1014 Understanding Self and Others - Biological Psychology PDF
- Biological Psychology PDF Introductory Psychology I Fall 2024
Summary
This textbook chapter details the biological perspective in psychology, explaining the functions of the nervous system, neurons, and related concepts. It covers the structures and importance of the nervous system, specifically neurons, as well as neurotransmitters and their role in communication. The chapter also explores the interaction between nerves, the brain, and glands within the nervous system.
Full Transcript
2 the biological perspective The Ironman® competition consists of a 2.4-mile swim, a 112-mile bike ride, and a 26.2-mile run. Dick Hoyt pulls his son Rick in a boat that is attached to a bungee cord, then he peddles Rick on a special two-seater bicycle, and finally he push...
2 the biological perspective The Ironman® competition consists of a 2.4-mile swim, a 112-mile bike ride, and a 26.2-mile run. Dick Hoyt pulls his son Rick in a boat that is attached to a bungee cord, then he peddles Rick on a special two-seater bicycle, and finally he pushes Rick across the finish line in a customized running chair. Why the unusual approach? Rick was born with spastic quadriplegia and cerebral palsy as the result of anoxia during birth. Unable to walk or talk, Rick learned to communicate by tapping his head against a head piece on his wheelchair when specific letters of the alphabet were highlighted on a specially designed computer screen. He eventually graduated from Boston University with a degree in special education. Current research to help individuals with brain injuries or neurological conditions has moved far beyond the computer that assisted Rick. Some of the most promising areas are in brain–computer interfaces (BCI) that use the brain’s electrical activity to communicate with others. It is possible that in the future, brain activity will enable individuals to control such assistive devices as prosthetic limbs or wheelchairs. What do you see as the brain’s role in our behavior? How much do you think your behavior is influenced by hormones and chemicals in the nervous system? CC Watch the Watch theVideo MyPsychLab.com Videoaton MyPsychLab.com 44 Why study the nervous system and the glands? How could we possibly understand any of our behavior, thoughts, or actions without know- ing something about the incredible organs that allow us to act, think, and react? If we can understand how the brain, the nerves, and the glands interact to control feelings, thoughts, and behavior, we can begin to truly understand the complex organism called a human being. learning objectives What are the nervous system, neurons, What are the different structures of the 2.1 and nerves, and how do they relate to 2.7 hindbrain and what do they do? one another? How do neurons use neurotransmitters What are the structures of the brain 2.2 to communicate with each other and 2.8 that control emotion, learning, memory, with the body? and motivation? How do the brain and spinal cord inter- What parts of the cortex control the dif- 2.3 act, what are some misconceptions about 2.9 ferent senses and the movement of the the brain, and what is neuroplasticity? body? How do the somatic and autonomic ner- What parts of the cortex are respon- 2.4 vous systems allow people and animals 2.10 sible for higher forms of thought, such to interact with their surroundings and as language? control the body’s automatic functions? How do the hormones released by How does the left side of the brain dif- 2.5 glands interact with the nervous system 2.11 fer from the right side? and affect behavior? How do psychologists study the brain What are some potential causes of 2.6 and how it works? 2.12 attention-deficit/hyperactivity disorder? 45 Success Center Neurons and Nerves: Building the Network Study on MyPsychLab Dynamic Study Modules 2.1 What are the nervous system, neurons, and nerves, and how do they relate to Watch the Video on MyPsychLab one another? Study Methods Managing Time This chapter will explore a complex system of cells, chemicals, and organs that work together to produce behavior, thoughts, and actions. The first part of this complex ar- Reading the Text rangement is the nervous system, a network of cells that carries information to and from Lecture Notes all parts of the body. The field of neuroscience is a branch of the life sciences that deals Exam Prep with the structure and functioning of the brain and the neurons, nerves, and nervous tis- Paper Writing sue that form the nervous system. Biological psychology, or behavioral neuroscience, Improve Memory is the branch of neuroscience that focuses on the biological bases of psychological pro- cesses, behavior, and learning, and it is the primary area associated with the biological perspective in psychology. Structure of the Neuron: The Nervous System’s Building Block In 1887, Santiago Ramón y Cajal, a doctor studying slides of brain tissue, first theorized that the nervous system was made up of individual cells (Ramón y Cajal, translation, 1995). Although the entire body is composed of cells, each type of cell has a special pur- pose and function and, therefore, a special structure. For example, skin cells are flat, but muscle cells are long and stretchy. Most cells have three things in common: a nucleus, a cell body, and a cell membrane holding it all together. The neuron is the specialized cell in the nervous system that receives and sends messages within that system. Neurons are one of the messengers of the body, and that means that they have a very special structure, which we will explore in the video The Basics: How the Brain Works, Part 1: The Neuron. CC Watch the Video, The Basics: How the Brain Works, Part 1: The Neuron, at MyPsychLab The parts of the neuron that receive messages from other cells are called the den- drites. The name dendrite means “tree-like,” or “branch,” and this structure does indeed look like the branches of a tree. The dendrites are attached to the cell body, or soma, which is the part of the cell that contains the nucleus and keeps the entire cell alive and functioning. The word soma means “body.” The axon (from the Greek for “axis”) is a fiber 46 the biological perspective 47 attached to the soma, and its job is to carry messages out to other cells. The end of the axon branches out into several shorter fibers that have swellings or little knobs on the ends 2.1 called axon terminals (may also be called presynaptic terminals, terminal buttons, or synaptic knobs), which are responsible for communicating with other nerve cells. (See Figure 2.1.) 2.2 Neurons make up a large part of the brain but they are not the only cells that affect our thinking, learning, memory, perception, and all of the other facets of life that make us who we are. The other primary cells are called glia, or glial cells, which serve a variety 2.3 of functions. Some glia serve as a sort of structure on which the neurons develop and work and that hold the neurons in place. For example, during early brain development, radial glial cells (extending from inner to outer areas like the spokes of a wheel) help This electron micrograph shows a 2.4 guide migrating neurons to form the outer layers of the brain. Other glia are involved stem cell in the process of becoming in getting nutrients to the neurons, cleaning up the remains of neurons that have died, a neuron. communicating with neurons and other glial cells, and providing insulation for neurons. 2.5 Glial cells affect both the functioning and structure of neurons and specific types also have properties similar to stem cells, which allow them to develop into new neurons, both during prenatal development and in adult mammals (Bullock et al., 2005; Kriegstein & 2.6 Alvarez-Buylla, 2009). Glial cells are also being investigated for their possible role in a variety of psychiatric disorders, including major depressive disorder and schizophrenia. It 2.7 appears in some areas of the brain, major depressive disorder is characterized by lower numbers of specific glial cells whereas in schizophrenia, parts of the brain have a greater number (Blank & Prinz, 2013). to Learning Objectives 14.5 and 14.8. Recent 2.8 findings also implicate glial cells in learning and behavior, both by affecting synaptic connectivity during development, and in mice transplanted with human glial cells, faster learning across a variety of learning and memory tasks (Han et al., 2013; Ji et al., 2013). 2.9 2.10 Axon terminal 2.11 Nucleus 2.12 Soma Axon Myelin sheath Dendrites Axon Axon terminal Figure 2.1 The Structure of the Neuron The electron micrograph on the left shows myelinated axons. 48 CHAPTER 2 Two special types of glial cells, called oligodendrocytes and Schwann cells, generate a 2.1 layer of fatty substances called myelin. Oligodendrocytes produce myelin for the neurons in the brain and spinal cord (the central nervous system); Schwann cells produce myelin for the neurons of the body (the peripheral nervous system). Myelin wraps around the 2.2 shaft of the axons, forming an insulating and protective sheath. Bundles of myelin-coated axons travel together as “cables” in the central nervous system called tracts, and in the 2.3 peripheral nervous system bundles of axons are called nerves. Myelin from Schwann cells has a unique feature that can serve as a tunnel through which damaged nerve fibers can reconnect and repair themselves. That’s why a severed toe might actually regain some 2.4 function and feeling if sewn back on in time. Unfortunately, myelin from oligodendro- cytes covering axons in the brain and spinal cord does not have this feature, and these axons are more likely to be permanently damaged. 2.5 The myelin sheath is a very important part of the neuron. It not only insulates and protects the neuron, it also speeds up the neural message traveling down the axon. As shown in Figure 2.1, sections of myelin bump up next to each other on the axon, similar to 2.6 the way sausages are linked together. The places where the myelin seems to bump are ac- tually small spaces on the axon called nodes, which are not covered in myelin. Myelinated and unmyelinated sections of axons have slightly different electrical properties. There are 2.7 also far more ion channels at each node. Both of these features affect the speed the electri- cal signal is conducted down the axon. When the electrical impulse that is the neural mes- 2.8 sage travels down an axon coated with myelin, the electrical impulse is regenerated at each node and appears to “jump” or skip rapidly from node to node down the axon (Koester & Siegelbaum, 2013; Schwartz et al., 2013). That makes the message go much faster down 2.9 the coated axon than it would down an uncoated axon of a neuron in the brain. In the disease called multiple sclerosis (MS), the myelin sheath is destroyed (possibly by the indi- vidual’s own immune system), which leads to diminished or complete loss of neural func- 2.10 tioning in those damaged cells. Early symptoms of MS may include fatigue, changes in vision, balance problems, and numbness, tingling, or muscle weakness in the arms or legs. 2.11 Exactly how does this “electrical message” work inside the cell? 2.12 Generating the Message Within the Neuron: The Neural Impulse A neuron that’s at rest—not currently firing a neural impulse or message—is actually electrically charged. Inside and outside of the cell is a semiliquid (jelly-like) solution in which there are charged particles, or ions. Although both positive and negative ions are located inside and outside of the cell, the relative charge of ions inside the cell is mostly negative, and the relative charge of ions outside the cell is mostly positive due to both diffusion, the process of ions moving from areas of high concentration to areas of low concentration, and electrostatic pressure, the relative electrical charges when the ions are at rest. The cell membrane itself is semipermeable. This means some substances that are out- side the cell can enter through tiny protein openings, or channels, in the membrane, while other substances in the cell can go outside. Many of these channels are gated—they open or close based on the electrical potential of the membrane—more about that in a minute. Inside the cell is a concentration of both smaller positively charged potassium ions and larger negatively charged protein ions. The negatively charged protein ions, however, are so big that they can’t get out, which leaves the inside of the cell primarily negative when at rest. Outside the cell are lots of positively charged sodium ions and negatively charged chloride ions, but they are unable to enter the cell membrane when the cell is at rest because the ion channels that would allow them in are closed. But because the outside sodium ions are positive and the inside ions are negative, and because opposite electrical charges attract each other, the sodium ions will cluster around the membrane. This dif- ference in charges creates an electrical potential. Watch the Video, The Basics: How the Brain Works, Part 1: Action Potential, at MyPsychLab the biological perspective 49 Think of the ions inside the cell as a baseball game inside a stadium (the cell walls). The sodium ions outside the cell are all the fans in the area, and they want to get inside 2.1 to see the game. When the cell is resting (the electrical potential is in a state called the resting potential, because the cell is at rest), the fans are stuck outside. The sodium ions 2.2 cannot enter when the cell is at rest, because even though the cell membrane has all these channels, the particular channels for the big sodium ions aren’t open yet. But when the cell receives a strong enough stimulation from another cell (meaning that the dendrites are 2.3 activated), the cell membrane opens up those particular channels, one after the other, all down its surface, allowing the sodium ions (the “fans”) to rush into the cell. That causes the inside of the cell to become mostly positive and the outside of the cell to become 2.4 mostly negative, because many of the positive sodium ions are now inside the cell—at the point where the first ion channel opened. This electrical charge reversal will start at the part of the axon closest to the soma, the axon hillock, and then proceed down the axon 2.5 in a kind of chain reaction. (Picture a long hallway with many doors in which the first door opens, then the second, and so on all the way down the hall.) This electrical charge reversal is known as the action potential because the electrical potential is now in action 2.6 rather than at rest. Each action potential sequence takes about one thousandth of a sec- ond, so the neural message travels very fast—from 2 miles per hour in the slowest, short- 2.7 est neurons to 270 miles per hour in other neurons. (See Figure 2.2 on the next page.) Now the action potential is traveling down the axon. When it gets to the end of the axon, something else happens: the message will get transmitted to another cell (that 2.8 step will be discussed momentarily). Meanwhile, what is happening to the parts of the cell that the action potential has already left behind? How does the cell get the “fans” back outside? Remember, the action potential means that the cell is now positive inside 2.9 and negative outside at the point where the channel opened. Several things happen to return the cell to its resting state. First, the sodium ion channels close immediately after the action potential has passed, allowing no more “fans” (sodium ions) to enter. The cell 2.10 membrane also literally pumps the positive sodium ions back outside the cell, kicking the “fans” out until the next action potential opens the ion channels again. This pumping 2.11 process is a little slow, so another type of ion gets into the act. Small, positively charged potassium ions inside the neuron move rapidly out of the cell after the action potential passes, helping to more quickly restore the inside of the cell to a negative charge. Now 2.12 the cell becomes negative inside and positive outside, and the neuron is capable of “firing off ” another message. Once the sodium pumps finish pumping out the sodium ions, the neuron can be said to have returned to its full resting potential, poised and ready to do it all again. To sum all that up, when the cell is stimulated, the first ion channel opens and the electrical charge at that ion channel is reversed. Then the next channel opens and that charge is reversed, but in the meantime the first ion channel has been closed and the charge is returning to what it was when it was at rest. The action potential is the sequence of ion channels opening all down the length of the cell’s axon. So if the stimulus that originally causes the neuron to fire is very strong, will the neuron fire more strongly than it would if the stimu- lus were weak? Neurons actually have a threshold for firing, and all it takes is a stimulus that is just strong enough to get past that threshold to make the neuron fire. Here’s a simple version of how this works: Each neuron is receiving many signals from other neurons. Some of these signals are meant to cause the neuron to fire, whereas others are meant to prevent the neuron from firing. The neuron constantly adds together the effects of the “fire” mes- sages and subtracts the “don’t fire” messages, and if the fire messages are great enough, the threshold is crossed and the neuron fires. When a neuron does fire, it fires in an all-or-none fashion. That is, neurons are either firing at full strength or not firing at 50 CHAPTER 2 Figure 2.2 The Neural Impulse Negatively charged 2.1 Action Potential Axon inner cell membrane In the graph, voltage readings + - + are shown at a given place on the + -- - + The Neuron at Rest + -- + + -- + 2.2 neuron over a period of 20 or 30 During the resting potential, + -- + + + + + + + + + + + + + -- + milliseconds (thousandths of a the neuron is negatively - - - - - - - - - - - - - - - - - - - - - - - - + + + + + + + + + + + + + + - + second). At first the cell is resting; charged inside and - + -- + + -- + positively charged outside. + -- + it then reaches threshold and an + -- 2.3 + - + action potential is triggered. After a + - brief hyperpolarization period, the cell returns to its resting potential. Nerve cell body Positive sodium ion Synaptic knob 2.4 Sodium ions, along Nerve with potassium ions, 2.5 - - - - - - impulse move outside -- - membrane -- - + + - ++ ++ + + - -- - -- - - + - - - - - + + 2.6 - + - - - - - + + -- - -- - + + - - ++ ++ + + - - Movement - - Sodium - - - - - - 2.7 of sodium - ions enter - ions next segment of axon The Neural Impulse The Neural Impulse Continues 2.8 The action potential occurs when As the action potential moves down the positive sodium ions enter into the axon toward the axon terminals, the cell cell, causing a reversal of the electrical areas behind the action potential return 2.9 charge from negative to positive. to their resting state of a negative charge as the positive sodium ions are pumped to the outside of the cell, and the positive potassium ions rapidly leave. 2.10 Action potential 40 2.11 Electrical charge (millivolts) 0 2.12 Threshold –50 –70 Resting potential Resting potential Hyperpolarization all—there’s no such thing as “partial” firing of a neuron. It would be like turning on a light switch—it’s either on or it’s off. Once the switch is turned to the on position, the light will come on. When it’s turned to the off position, the light is off. So, what’s the difference between strong stimulation and weak stimulation? A strong message will cause the neuron to fire repeatedly (as if someone flicked the light switch on and off as quickly as possible), and it will also cause more neurons to fire (as if there were a lot of lights going on and off instead of just one). Now that we know how the message travels within the axon of the cell, what is that “something else” that happens when the action potential reaches the end of the axon? the biological perspective 51 Sending the Message to Other Cells: The Synapse 2.1 2.2 How do neurons use neurotransmitters to communicate with each other and with the body? 2.2 Look once again at the axon terminals in Figure 2.1. Figure 2.3 shows an axon terminal enlarged to giant scale. Notice that the presynaptic terminal is not empty. It has a number 2.3 of little sac-like structures in it called synaptic vesicles. The word vesicle is Latin and means a “little blister” or “fluid-filled sac.” Inside the synaptic vesicles are chemicals suspended in fluid, which are molecules 2.4 of substances called neurotransmitters. The name is simple enough—they are inside a neuron and they are going to transmit a message. (Neurons have traditionally been viewed as containing a single type of neurotransmitter but is now accepted that neurons 2.5 may release more than one neurotransmitter. For simplicity and unless otherwise speci- fied, our discussion throughout the text will assume a single, predominant neurotrans- mitter is being released.) Next to the axon terminal is the dendrite of another neuron (see 2.6 Figure 2.3). Between them is a fluid-filled space called the synapse or the synaptic gap. Instead of an electrical charge, the vesicles at the end of the axon (also called the pre- 2.7 synaptic membrane) contain the molecules of neurotransmitters, and the surface of the dendrite next to the axon (the postsynaptic membrane) contains ion channels that have receptor sites, proteins that allow only particular molecules of a certain shape to fit into This electron micrograph shows a 2.8 it, just as only a particular key will fit into a keyhole. motor neuron making contact with How do the neurotransmitters get across the gap? Recall the action potential mak- muscle fibers. ing its way down the axon after the neuron has been stimulated. When that action po- 2.9 tential, or electrical charge, reaches the synaptic vesicles, the synaptic vesicles release their neurotransmitters into the synaptic gap. The molecules then float across the synapse and many of them fit themselves into the receptor sites, opening the ion channels and allow- 2.10 ing sodium to rush in, activating the next cell. It is this very activation that stimulates, or releases, the action potential in that cell. It is important to understand that the “next cell” may be a neuron, but it may also be a cell on a muscle or a gland. Muscles and glands 2.11 have special cells with receptor sites on them, just like on the dendrite of a neuron. 2.12 Nerve impulse Axon terminal of presynaptic neuron Synaptic vesicles Neurotransmitter Surface of postsynaptic neuron Figure 2.3 The Synapse The nerve impulse reaches the axon terminal, triggering the release of neurotransmitters from the synaptic vesicles. The molecules of neurotransmitter Sodium ions cross the synaptic gap to fit into the receptor sites that fit the shape of the molecule, opening the ion Receptor site channel and allowing sodium ions to rush in. 52 CHAPTER 2 So far, we’ve been talking about the synapse as if neurotransmitters always cause 2.1 the next cell to fire its action potential (or, in the case of a muscle or gland, to contract or start secreting its chemicals). But the neurons must have a way to be turned off as well as on. Otherwise, when a person burns a finger, the pain signals from those neurons would 2.2 not stop until the burn was completely healed. Muscles are told to contract or relax, and glands are told to secrete or stop secreting their chemicals. The neurotransmitters found 2.3 at various synapses around the nervous system can either turn cells on (called an excitatory effect) or turn cells off (called an inhibitory effect), depending on exactly what synapse is being affected. Although some people refer to neurotransmitters that turn cells on as ex- 2.4 citatory neurotransmitters and the ones that turn cells off as inhibitory neurotransmitters, it’s really more correct to refer to excitatory synapses and inhibitory synapses. In other words, it’s not the neurotransmitter itself that is excitatory or inhibitory, but rather it is 2.5 the effect of that neurotransmitter that is either excitatory or inhibitory at the receptor sites of a particular synapse. 2.6 Neurotransmitters: Messengers of the Network The first neurotransmitter to be identified was named acetylcholine (ACh). It is found at the synapses between neurons and muscle cells. Acetylcholine stimulates the skeletal 2.7 muscles to contract but actually slows contractions in the heart muscle. If acetylcholine receptor sites on the muscle cells are blocked in some way, then the acetylcholine can’t get 2.8 to the site and the muscle will be incapable of contracting—paralyzed, in other words. This is exactly what happens when curare, a drug used by South American Indians on their blow darts, gets into the nervous system. Curare’s molecules are just similar enough 2.9 to fit into the receptor site without actually stimulating the cell, making curare an antagonist (a chemical substance that blocks or reduces the effects of a neurotransmit- ter) for ACh. 2.10 What would happen if the neurons released too much ACh? The bite of a black widow spider does just that. Its venom stimulates the release of excessive amounts of ACh and causes convulsions and possible death. Black widow spider venom is an agonist (a 2.11 chemical substance that mimics or enhances the effects of a neurotransmitter) for ACh. ACh also plays a key role in memory, arousal, and attention. For example, ACh is 2.12 found in the hippocampus, an area of the brain that is responsible for forming new mem- ories, and low levels of ACh have been associated with Alzheimer’s disease, the most common type of dementia. to Learning Objective 6.12. We will focus more on agonists and antagonists later in the chapter. Dopamine (DA) is a neurotransmitter found in the brain, and like some of the other neurotransmitters, it can have different effects depending on the exact location of its activity. For example, if too little DA is released in a certain area of the brain, the re- sult is Parkinson’s disease—the disease currently being battled by former boxing champ Muhammad Ali and actor Michael J. Fox (Ahlskog, 2003). If too much DA is released in other areas, the result is a cluster of symptoms that may be part of schizophrenia (Akil et al., 2003). to Learning Objective 14.8. Watch the Video, In the Real World: Neurotransmitters, at MyPsychLab Serotonin (5-HT) is a neurotransmitter originating in the lower part of the brain that can have either an excitatory or inhibitory effect, depending on the particular synapses being affected. It is associated with sleep, mood, anxiety, and appetite. For e xample, low levels of 5-HT activity have been linked to depression. to Learning Objective 14.5. Although ACh was the first neurotransmitter found to have an excitatory effect The venom of the black widow at the synapse, the nervous system’s major excitatory neurotransmitter is glutamate. Like spider causes a flood of acetylcholine to be released into ACh, glutamate plays an important role in learning and memory, and may also be involved the body’s muscle system, causing in the development of the nervous system and in synaptic plasticity (the ability of the convulsions. the biological perspective 53 brain to change connections among its neurons). However, an excess of glutamate results in overactivation and neuronal damage, and may be associated with the cell death that 2.1 occurs after stroke, head injury, or in degenerative diseases like Alzheimer’s disease and Huntington disease ( Julien et al., 2011; Siegelbaum et al., 2013). 2.2 Another neurotransmitter is gaba-aminobutyric acid or GABA. Whereas glutamate is the major neurotransmitter with an excitatory effect, GABA is the most common neu- rotransmitter producing inhibition in the brain. GABA can help to calm anxiety, for 2.3 example, by binding to the same receptor sites that are affected by tranquilizing drugs and alcohol. In fact, the effect of alcohol is to enhance the effect of GABA, which causes the general inhibition of the nervous system associated with getting drunk. This makes 2.4 alcohol an agonist for GABA. to Learning Objective 4.8. (See Table 2.1 for a list of some neurotransmitters and their functions.) A group of substances known as neuropeptides can serve as neurotransmit- 2.5 ters, hormones, or influence the action of other neurotransmitters (Schwartz & Javitch, 2013). You may have heard of the set of neuropeptides called endorphins— pain-controlling chemicals in the body. When a person is hurt, a neurotransmitter that 2.6 signals pain is released. When the brain gets this message, it triggers the release of en- dorphins. The endorphins bind to receptors that open the ion channels on the axon. This 2.7 causes the cell to be unable to fire its pain signal and the pain sensations eventually lessen. For example, you might bump your elbow and experience a lot of pain at first, but the pain will quickly subside to a much lower level. Athletes may injure themselves during 2.8 an event and yet not feel the pain until after the competition is over, when the endorphin levels go down. The name endorphin comes from the term endogenous morphine. (Endogenous means 2.9 “native to the area”—in this case, native to the body.) Scientists studying the nervous system found receptor sites that fit morphine molecules perfectly and decided that there must be a natural substance in the body that has the same effect as morphine. Endor- 2.10 phins are one reason that heroin and the other drugs derived from opium are so addic- tive—when people take morphine or heroin, their bodies neglect to produce endorphins. 2.11 When the drug wears off, they are left with no protection against pain at all, and every- thing hurts. This pain is one reason why most people want more heroin, creating an ad- dictive cycle of abuse. to Learning Objective 4.8. 2.12 Table 2.1 Some Neurotransmitters and Their Functions Neurotransmitters Functions Acetylcholine (ACh) Excitatory or inhibitory; involved in arousal, attention, memory, and controls muscle contractions Norepinephrine (NE) Mainly excitatory; involved in arousal and mood Dopamine (DA) Excitatory or inhibitory; involved in control of movement and sensations of pleasure Serotonin (5-HT) Excitatory or inhibitory; involved in sleep, mood, anxiety, and appetite Gaba-aminobutyric Major inhibitory neurotransmitter; involved in sleep and inhibits acid (GABA) movement Glutamate Major excitatory neurotransmitter; involved in learning, memory formation, nervous system development, and synaptic plasticity Endorphins Inhibitory neural regulators; involved in pain relief 54 CHAPTER 2 2.1 If the neurotransmitters are out there in the synaptic gap and in the receptor sites, what happens to them when they aren’t needed anymore? 2.2 Cleaning Up the Synapse: Reuptake and Enzymes The neurotransmitters have to get out of the receptor sites before the next stimulation can 2.3 occur. Some just drift away through the process of diffusion, but most will end up back in Presynaptic neuron the synaptic vesicles in a process called reuptake. (Think of a little suction 2.4 tube, sucking the chemicals back into the vesicles.) That way, the synapse is Dopamine cleared for the next release of neurotransmitters. Some drugs, like cocaine, affect the nervous system by blocking the reuptake process. See Figure 2.4 2.5 Cocaine Dopamine for a visual representation of how dopamine is affected by cocaine. Synapse reuptake sites There is one neurotransmitter that is not taken back into the vesi- Dopamine cles, however. Because ACh is responsible for muscle activity, and muscle 2.6 receptors activity needs to happen rapidly and continue happening, it’s not possible Postsynaptic neuron to wait around for the “sucking up” process to occur. Instead, an enzyme* Figure 2.4 Reuptake specifically designed to break apart ACh clears the synaptic gap very quickly (a process 2.7 called enzymatic degradation.) There are enzymes that break down other neurotrans- of Dopamine Dopamine is removed from the mitters as well. synapse by reuptake sites. Cocaine 2.8 acts by blocking dopamine I think I understand the synapse and neurotransmitters now, but reuptake sites, allowing dopamine how do I relate that to the real world? to remain active in the synapse 2.9 longer. Knowing how and why drugs affect us can help us understand why a doctor might prescribe a particular drug or why certain drugs are dangerous and should be avoided. Because the chemical molecules of various drugs, if similar enough in shape to the neu- 2.10 rotransmitters, can fit into the receptor sites on the receiving neurons just like the neu- rotransmitters do, drugs can act as agonists or antagonists. Drugs acting as agonists, for 2.11 example, can mimic or enhance the effects of neurotransmitters on the receptor sites of the next cell. This can result in an increase or decrease in the activity of the receiving cell, depending on what the effect of the original neurotransmitter (excitatory or inhibitory) 2.12 was going to be. So if the original neurotransmitter was excitatory, the effect of the ago- nist will be to increase that excitation. If it was inhibitory, the effect of the agonist will be to increase that inhibition. Another deciding factor is the nervous system location of the neurons that use a specific neurotransmitter. Watch the Video, What’s In It For Me?: Your Brain on Drugs, at MyPsychLab For example, some antianxiety medications, such as diazepam (Valium®), are clas- sified as benzodiazepines ( to Learning Objective 15.8.) and are agonists for GABA, the primary inhibitory neurotransmitter in the brain. Areas of the brain that you will learn about later that play a role in controlling anxiety, agitation, and fear include the amygdala, orbitofrontal cortex, and the insula (LeDoux & Damasio, 2013; Zilles & Amunts, 2012). By increasing the inhibitory (calming) action of GABA, the benzodiaz- epines directly calm these specific brain areas ( Julien et al., 2011; Preston et al., 2008). Other drugs act as antagonists, blocking or reducing a cell’s response to the action of other chemicals or neurotransmitters. Although an antagonist might sound like it has only an inhibitory effect, it is important to remember that if the neurotransmitter that the an- tagonist affects is inhibitory itself, the result will actually be an increase in the activity of the cell that would normally have been inhibited; the antagonist blocks the inhibitory effect. Lastly, some drugs yield their agonistic or antagonistic effects by impacting the amount of neurotransmitter in the synapse. They do so by interfering with the regular reuptake or enzymatic degradation process. Remember that the neurotransmitter sero- tonin helps regulate and adjust people’s moods, but in some people the normal process of *enzyme: a complex protein that is manufactured by cells. the biological perspective 55 adjustment is not working properly. Some of the drugs used to treat depression are called SSRIs (selective serotonin reuptake inhibitors). SSRIs block the reuptake of serotonin, 2.1 leaving more serotonin available in the synapse to bind with receptor sites. Over several weeks, the individual’s mood improves. Although the reason for this improvement is not 2.2 as simple as once believed (i.e., low levels of serotonin = low levels of mood) or fully understood, SSRIs are effective for depression, anxiety, and obsessive-compulsive disorder (Hyman & Cohen, 2013; Julien et al., 2011, Stahl, 2013). 2.3 This section covered the neuron and how neurons communicate. The next section looks at the bigger picture—the nervous system itself. 2.4 Explore the Concept at MyPsychLab 2.1 2.2 2.5 CO N CEPT MAP The nervous system is a network of cells that carries information to and from all parts of the body; neuroscience is the field of study that deals with the structure of the brain and components of the nervous system 2.6 oligodendrocytes: Neurons and Nerves produce myelin in the myelin insulates axons 2.7 central nervous system glial cells: provide physical and speeds up transmission and metabolic support Schwann cells: of neural message to neurons; communicate produce myelin in the with other cells; specific peripheral nervous system 2.8 types have stem cell-like properties brain is comprised dendrites 2.9 of neurons soma have specialized and neurons: axon axon terminals components glial cells specialized 2.10 cells in nervous have an made possible by balance between ions in and outside of the cell system; send electrical charge membrane is semipermeable; inside is negatively charged as and receive at rest—the resting compared to outside 2.11 messages potential fire in an within that change in the electrical all-or-none fashion system are affected by charge can result in an neurotransmitters action potential; cell fires; cell firing is dependent (see Table 2.1); inside becomes positive on sum of excitatory 2.12 chemicals that have relative to outside and inhibitory messages an effect on neurons received by the cell neurotransmitters (NTs) move across synapse and activate are separated by a gap ion channel receptor sites on adjacent cells; some NTs are called the synapse; agonistic/excitatory, others are antagonistic/inhibitory when nerve impulse reaches axon terminals, neurotransmitter action stopped by exiting synapse; neurotransmitter neurotransmitters end back up in the releasing cell is released into through reuptake or broken down by enzymes synaptic space Practice quiz How Much Do You Remember? Answers available in answer key. Pick the best answer. 1. Which part of the neuron carries messages to other cells? 4. Neurotransmitters must pass from an axon terminal to the a. axon c. soma next dendrite by crossing a fluid-filled space called the b. dendrite d. myelin a. synaptic gap. c. neuron. 2. Which one of the following is NOT a function of glial cells? b. reuptake inhibitor. d. glial cell. a. getting nutrients to the neurons 5. The venom of a black widow spider acts like a(n) b. generating action potentials __________ by mimicking the effects of acetylcholine. c. cleaning up the remains of dead neurons a. agonist c. protagonist d. providing insulation b. antagonist d. glial cell 3. When a neuron’s resting potential is occurring, the neuron is 6. Which of the following is associated with pain relief? __________ charged on the inside. a. acetylcholine c. serotonin a. positively c. both positively and negatively b. glutamate d. endorphins b. negatively d. neutrally 56 CHAPTER 2 2.1 An Overview of the Nervous System Now that we have looked at the cells that make up the nervous system and ways in which they process and communicate information, take a look at Figure 2.5. This figure shows 2.2 the organization of the various parts of the nervous system and will help in understand- ing how all the different parts work together in controlling the way people and animals 2.3 think, act, and feel. Watch the Video, The Basics: How the Brain Works, Part 2: Nervous System, at MyPsychLab Figure 2.5 An Overview of the Nervous system 2.4 Nervous System 2.5 Central nervous system Peripheral nervous system The brain and spinal cord Transmits information to and from the central 2.6 nervous system 2.7 Brain Spinal cord Autonomic Somatic nervous system nervous system Interprets and Pathway 2.8 stores information connecting the Automatically Carries sensory and sends orders brain and the regulates glands, information to muscles, glands, peripheral internal organs and controls and organs nervous system and blood vessels, movement 2.9 pupil dilation, of the skeletal digestion, and muscles blood pressure 2.10 2.11 Parasympathetic Sympathetic Sensory system Motor system division division (afferent) (efferent) Maintains body Prepares the Carries messages Carries messages 2.12 functions under body to react from senses to from CNS to ordinary and expend CNS muscles and conditions; energy in times glands saves energy of stress the Central Nervous System: The “Central Processing Unit” 2.3 How do the brain and spinal cord interact, what are some misconceptions about the brain, and what is neuroplasticity? The central nervous system (CNS) is composed of the brain and the spinal cord. Both the brain and the spinal cord are composed of neurons and glial cells that control the life- sustaining functions of the body as well as all thought, emotion, and behavior. The Brain The brain is the core of the nervous system, the part that makes sense of the information received from the senses, makes decisions, and sends commands out to the muscles and the rest of the body, if needed. Many different areas of the brain are involved in preparing us for an appropriate response to the information received, and the brain is responsible for cognition and thoughts, including learning, mem- ory, and language. Later parts of this chapter will cover the brain in more detail, but for now, you should know the brain is organized into different regions, each with primary functions. While the neurons in each of the different areas work in much the same way, it is the groups of cells and the connections between them and other the biological perspective 57 parts of the brain or components of the nervous system, and our experiences, that influence the 2.1 various functions found in specific brain areas (Amaral & Strick, 2013; Heimer, 1995; Squire & Kandel, 2009). 2.2 The Spinal Cord The spinal cord is a long bundle of neurons that serves two vital func- 2.3 tions for the nervous system. Look at the cross- section of the spinal cord in Figure 2.6. Notice that it seems to be divided into two areas, a 2.4 lighter outer section and a darker inner section. If it were a real spinal cord, the outer section The look on this young woman’s face would appear to be white and the inner section clearly indicates that she has experienced 2.5 pain in her finger. Pain is a warning signal would seem gray. That’s because the outer sec- that something is wrong—in this case that tion is composed mainly of myelinated axons touching the thorns on the stem of the 2.6 and nerves, which appear white, whereas the in- rose was a bad idea. What might be some ner section is mainly composed of cell bodies of of the problems encountered by a person neurons, which appear gray. The purpose of the who could feel no pain at all? 2.7 outer section is to carry messages from the body up to the brain and from the brain down to the body. It is simply a message “pipeline.” To the 2.8 brain The inside section, which is made up of cell bod- 2. Sensory neurons excite interneurons ies separated by glial cells, is actually a primitive sort in the dorsal gray Cross-section of of “brain.” This part of the spinal cord is responsible portion of the spinal cord 2.9 for certain reflexes—very fast, lifesaving reflexes. To spinal cord. understand how the spinal cord r eflexes work, it is im- Sensory 2.10 portant to know there are three basic types of neurons: neuron afferent (sensory) neurons that carry messages from the senses to the spinal cord, efferent (motor) neurons 2.11 that carry messages from the spinal cord to the muscles and glands, and interneurons that connect the afferent neurons to the motor neurons (and make up the inside of the spinal cord and much of the brain 2.12 itself ). (See Figure 2.6.) Touch a flame or a hot stove with your fin- ger, for example, and an afferent neuron will send the pain message 3. Interneurons excite motor neurons in up to the spinal column where it enters into the central area of the ventral gray the spinal cord. The interneuron in that central area will then portion of the receive the message and send out a response along an effer- 4. Motor nerves spinal cord. exit the spinal ent neuron, causing your finger to pull back. This all happens cord, excite the very quickly. If the pain message had to go all the way up to muscle, and the brain before a response could be made, the response initiate a movement. time would be greatly increased and more damage would be done to your finger. So having this kind of reflex arc controlled by the spinal cord alone allows Figure 2.6 The Spinal Cord for very fast response times. (A good way to avoid Reflex mixing up the terms afferent and efferent The pain from the burning heat is to remember “afferent neurons access of the candle flame stimulates the the spinal cord, efferent neurons exit.” afferent nerve fibers, which carry The pain m essage does eventually get to 1. Flame stimulates the message up to the interneurons the brain, where other motor r esponses pain receptors in the middle of the spinal cord. (sensory neurons). The interneurons then send a may be triggered, like saying “Ouch!” message out by means of the and putting the finger in your mouth. efferent nerve fibers, causing the Explore the Concept The Nerve Impulse hand to jerk away from the flame. in Afferent and Efferent Neurons, at MyPsychLab 58 CHAPTER 2 2.1 psychology in the news 2.2 Fact or Fiction: Focus on the Brain, but Check Your Sources! 2.3 As stressed in Chapter One, critical thinking is a valuable skill and one that is very useful in the study of psychology. One of the basic points highlighted was that not all evidence is equal in quality. to Learning Objective 1.14. Have you 2.4 ever heard a claim about the brain that you were not sure was true? Whether you get your news from blogs or Web sites, listen to podcasts, or watch the 2.5 local or national news on your television, computer, or smartphone, you have probably read/ heard/seen a segment that addresses some aspect of the human brain. Some recent and not-so-recent brain-related news items have included: 2.6 “Older brains can’t make new cells” “Listening to classical music makes you smarter—also known as The Mozart Effect” 2.7 “Autism is caused by childhood vaccinations” “People only use 10 percent of their brain” Items adapted from Neuromyths, BrainFacts.org, Society for Neuroscience. 2.8 Available at http://www.brainfacts.org/neuromyths/ Do any of the above “headlines” look familiar? Given the inherent interest in many of these 2.9 topics and the number of people who reportedly “know” these facts, they have often been considered the truth. But in fact, all of the above items have been found to be false or have not been supported by conclusive, scientific evidence. So how do you go about evaluating brain- 2.10 related news the next time it flashes across the headlines or shows up on your news feed? Think back to the research methods and discussed in Chapter One. to Learning 2.11 Objectives 1.6–1.11. Did the news item provide details about the actual study? If it did, what were the data collection methods used in the study? Do the methods used support the reported findings? Was a representative sample used? Did the researchers conduct an 2.12 experiment or did the news item confuse correlation with causation? Some of the best sources are the original research studies, assuming the researchers collected data in an unbiased manner and followed established research methods. Findings that are published in peer-reviewed journals (where research is reviewed by other experts in that area) are especially helpful, as are edited books with contributions by experts in the associated field. The challenge for students early in their psychological studies is that it is not so easy to fully understand the methods, statistics, or terminology used in the studies. For students, aside from your textbooks and professors, other good resources are the educational materials provided by professional organizations. For psychology in general, the American Psychological Association (APA) and the Association for Psychological Science (APS) are two great sources of information. In addition to various peer-reviewed journals and other educational publications, they both have informative Web sites. For information more specifically related to the brain and neuroscience, one of the best sources of research and educational material is the Society for Neuroscience (SfN). The four misconceptions mentioned earlier are based on eight myths about the brain that are covered in one of their educational Web sites, Neuromyths, and can be viewed on their Web site, http://www.brainfacts.org/neuromyths/ So the next time you see a news item about the brain, check your sources and remain mindful that some sources are certainly more helpful than others. Useful Web sites: American Psychological Association (APA)—www.apa.org Association for Psychological Science (APS)—www.psychologicalscience.org the biological perspective 59 Society for Neuroscience (SfN)—www.sfn.org BrainFacts.org—www.brainfacts.org 2.1 Questions for Further Discussion 2.2 1. How might your personal experience with a brain-related disorder (e.g., grandparent with Alzheimer’s disease) affect your ability to critically evaluate a claim related to the disorder (e.g., “Cure for Alzheimer’s Found!”)? 2.3 2. As a student in psychological science, how would you explain the need for replicability in research to a family member that is not as familiar with the scientific method? 2.4 If the spinal cord is such an important link between the body and the brain, what happens if it is damaged? 2.5 Damage to the Central Nervous System Damage to the central nervous system was once thought to be permanent. Neurons in the brain and spinal cord were not seen as 2.6 capable of repairing themselves. When people recovered from a stroke, for example, it was assumed that it was primarily due to healthy brain cells taking over the functions of the damaged ones. Scientists have known for a while now that some forms of central nervous 2.7 system damage can be repaired by the body’s systems, and in recent years great strides have been made in repairing spinal cord damage. The brain actually exhibits a great deal of neuroplasticity, the ability to constantly change both the structure and function of many 2.8 cells in the brain in response to experience and even trauma (Neville & Bavelier, 2000; Rossini et al., 2007; Sanders et al., 2008). The video, Special Topics: The Plastic Brain: Over- view of Neuroplasticity explains this process in more detail. 2.9 2.10 2.11 2.12 CC Watch the Video, Special Topics: The Plastic Brain: Overview of Neuroplasticity at MyPsychLab Scientists have been able to implant nerve fibers from outside the spinal cord onto a damaged area and then “coax” the damaged spinal nerves to grow through these “tunnels” of implanted fibers (Cheng et al., 1996). Researchers are also examining the effects of implanting Schwann cells from the peripheral nervous system to the central nervous sys- tem to aid in treating spinal cord injuries (Deng et al., 2013). The brain can change itself quite a bit by adapting neurons to serve new functions when old neurons die or are dam- aged. Dendrites grow and new synapses are formed in at least some areas of the brain, as people learn new things throughout life (Sanes & Jessell, 2013a, 2013b). Researchers are constantly looking for new ways to repair the brain. One avenue of research has involved scientists investigating the possibility of transplanting stem cells to 60 CHAPTER 2 repair damaged or diseased brain tissue. Stem cells can become any cell in the body and are part of the central nervou 2.1 may offer promise for addressing diseases such as Parkinson’s and Alzheimer’s, or the re- groups of neurons near the sp pair of damaged spinal cords or brain tissue. If stem cells can be implanted into areas that The Autonomic Nervous Sy have been damaged, the newly developed neurons may assume the roles that the original this system are more or less a 2.2 (now damaged) neurons can no longer perform. division of the peripheral ner autonomic division controls e 2.3 Okay, that takes care of the central nervous system, except for muscles. The autonomic nervo the detail on the brain. How does the central nervous system com- sion and the parasympathetic d municate with the rest of the body? representation of how all the 2.4 back at Figure 2.5.) The Peripheral Nervous System: Nerves on the Edge The Sympathetic Divisi 2.5 How do the somatic and autonomic nervous systems allow people and animals system is primarily located on 2.4 to interact with their surroundings and control the body’s automatic functions? top of the ribcage to the waist 2.6 sympathetic division is in symp Brain The term peripheral refers to things that are not in the center or is usually called the “fight-or- (CNS) that are on the edges of the center. The peripheral nervous sys- with all kinds of stressful even 2.7 tem or PNS (see Figure 2.7 and also refer back to Figure 2.5) is these events might be anger ( made up of all the nerves and neurons that are not contained in ously) or even extreme joy or the brain and spinal cord. It is this system that allows the brain division’s job is to get the bod 2.8 and spinal cord to communicate with the sensory systems of the a fight-or-flight moment at le eyes, ears, skin, and mouth and allows the brain and spinal cord Fly or Fight? to learn more ab to control the muscles and glands of the body. The PNS can be 2.9 Spinal divided into two major systems: the somatic nervous system, cord Nerves (CNS) which consists of nerves that control the voluntary muscles of the 2.10 (PNS) body, and the autonomic nervous system (ANS), which consists of nerves that control the involuntary muscles, organs, and glands. The Somatic Nervous System One of the parts of a neuron 2.11 is the soma, or cell body (remember that the word soma means “body”). The somatic nervous system is made up of the sensory pathway, which comprises all the nerves carrying messages from 2.12 the senses to the central nervous system (those nerves contain- ing afferent neurons), and the motor pathway, which is all of the nerves carrying messages from the central nervous system to the voluntary, or skeletal,* muscles of the body—muscles that allow people to move their bodies (those nerves composed of efferent neurons). When people are walking, raising their hands in class, lifting a flower to smell, or directing their gaze toward the person they are talking to or to look at a pretty picture, they are using the somatic nervous system. (As seen in the discussion of spinal cord reflexes, although these muscles are called the “voluntary muscles,” they can move involuntarily when a reflex response oc- curs. They are called “voluntary” because they can be moved at will but are not limited to only that kind of movement.) Involuntary** muscles, such as the heart, stomach, and intestines, together with glands such as the adrenal glands and the pancreas, are all controlled by clumps of neurons located on or near the spinal column. (The words on or near are used quite deliberately here. The neurons inside the spinal column Figure 2.7 The Peripheral Nervous *skeletal: having to do with the bones of the body, or skeleton. System **involuntary: not under deliberate control. the biological perspective 61 come any cell in the body and are part of the central nervous system, not the peripheral nervous system.) These large n’s and Alzheimer’s, or the re- groups of neurons near the spinal column make up the autonomic nervous system. 2.1 n be implanted into areas that The Autonomic Nervous System The word autonomic suggests that the functions of ume the roles that the original this system are more or less automatic, which is basically correct. Whereas the somatic 2.2 division of the peripheral nervous system controls the senses and voluntary muscles, the autonomic division controls everything else in the body—organs, glands, and involuntary vous system, except for muscles. The autonomic nervous system is divided into two systems, the sympathetic divi- 2.3 ral nervous system com- sion and the parasympathetic division. (See Figure 2.8 on the next page.) (For a schematic representation of how all the various sections of the nervous system are organized, look back at Figure 2.5.) 2.4 the Edge The Sympathetic Division The sympathetic division of the autonomic nervous 2.5 system is primarily located on the middle of the spinal column—running from near the top of the ribcage to the waist area. It may help to think of the name in these terms: The