FFP1 Electron Transport Chain (ETC) PDF
Document Details
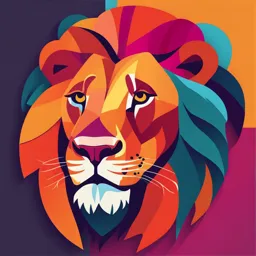
Uploaded by SumptuousSugilite7063
RCSI Medical University of Bahrain
2024
Royal College of Surgeons in Ireland
Dr Jeevan Shetty
Tags
Summary
These lecture notes cover the electron transport chain (ETC) for first-year medical students at the Medical University of Bahrain. The document includes learning outcomes, diagrams, and information about oxidative phosphorylation. These notes are from October 2024.
Full Transcript
Royal College of Surgeons in Ireland – Medical University of Bahrain FFP1:Electron Transport Chain (ETC) Module: FFP1 Code :DEM-MED1-101-FFP1 Class: MedYear1 semester 1 Lecturer: Dr Jeevan Shetty Date: 15th October 2024 Royal College of Surgeons in Ireland – Medical University of Bah...
Royal College of Surgeons in Ireland – Medical University of Bahrain FFP1:Electron Transport Chain (ETC) Module: FFP1 Code :DEM-MED1-101-FFP1 Class: MedYear1 semester 1 Lecturer: Dr Jeevan Shetty Date: 15th October 2024 Royal College of Surgeons in Ireland – Medical University of Bahrain Learning Outcomes Relate oxidative phosphorylation to the overall map of metabolism Describe the subcellular localisation and organisation of the components of the Electron Transport Chain and ATP Synthase. Discuss the chemiosmotic theory and the coupling of Electron Transport to ATP generation. Explain how the proton gradient drives ATP synthesis Explain the normal and pathophysiological outcomes of uncoupling. Describe OxPhos diseases and how iron and vitamin deficiencies give rise to defects in electron transport Pre-class Activity – Can watch this video & Glycolysis_TCA https://www.youtube.com/watch? v=Ak17BWJ3bLg&ab_channel=DongemBiology Electron Transport Chain (ETC) Final common pathway - electrons derived from different fuels of the body flow to oxygen (O2), reducing it to H2O Carbohydrates Triglycerides Proteins Coenzymes NADH and FADH2 produced in catabolism and the TCA cycle. Glucose Fatty acids Amino acids Reduced Coenzymes donate a pair of Small carbon chains electrons to electron carriers. Electrons passed down ETC losing TCA NADH & cycle FADH2 energy & generating ATP(oxidative phosphorylation-OXPHOS) Electron Transport Chain Energy not converted to ATP used to transport Ca2+ & generate heat Coenzymes NAD+ + 2H+ + 2e- NADH + H+ FAD + 2H+ + 2e- FADH2 These electrons contain a How cells lot of energy. Unlock them Electron transport chain e- in Electron transport chain ADP + Pi ATP Enzyme: ATP Synthase ATP out Mitochondria ETC is located in the inner mitochondrial membrane. Outer membrane porous to small NADH and FADH2 are used to generate molecules. energy to make ATP. Inner membrane impermeable to most ions, small & large molecules. Glycolysis takes place in the cytoplasm & produce NADH NADH & FADH2 need to reach the ETC complex for ATP synthesis. TCA cycle in the mitochondrial Mitochondrion Mitochondria from lung tissue matrix (except succinate DH (Transmission electron micrograph which is also part of ETC Complex II) 5 Membrane transport of other molecules (mitochondrial inner membrane) Specific transport systems for other molecules also exist Eg. ADP and Pi are needed for ATP synthesis ADP is transported in in exchange for ATP Substrate shuttles-Transport between cytosol and mitochondria Substrate shuttles (transport proteins) Some NADH is generated by glycolysis in the cytosol permit passage of specific molecules BUT from cytosol to the mitochondrial matrix. The inner mitochondrial membrane lacks an NADH transport protein Only the electrons from cytosolic NADH are transported into the mitochondrion, by shuttle systems Electrons from cytosolic NADH transferred to an intermediate which crosses the mitochondrial membrane and then transfers those electrons back to mitochondrial NAD+ or FAD 1) Glycerol 3-phosphate shuttle –e from cytosolic NADH transferred to mitochondrial FAD 2) Malate-aspartate shuttle -–e from cytosolic NADH transferred to mitochondrial NAD+ Electron Transport Chain (ETC) H = 1 é + 1 proton (H+) Respiratory chain components Complexes I-IV Each complex accepts & donates e-’s to mobile carriers (cyt C & CoQ) Carriers can receive e-’s & donate e-’s Electrons ultimately combine with O2 & hydrogens to form H2O Freely diffusible components NADH (mitochondrial matrix) Coenzyme Q (Ubiquinone) (mobile within lipid bilayer) Cytochrome C (peripheral membrane protein -outer membrane surface) Molecular O2 ETC Components All members of the chain (except coenzyme Q) are large protein complexes Coupled to metal ions e.g. Iron and Iron- Sulfur centres, Porphyrin rings (Fe ion) or copper ions (cytochromes) Fe3+ Fe2+ Fe3+ + e- - e- Makes them excellent electron carriers Hydrogen carriers e.g FMN & FAD Respiratory chain components IV. Cytochrome C oxidase: (a+a3) 3 multi-protein complexes localised in the inner mitochondrial membrane Copper & cytochrome e- carriers Transfers e- from cytochrome C to O2 I. NADH-Q reductase complex II. Succinate ubiquinone III. Cytochrome C reductase (NADH dehydrogenase): Fe- oxidoreductase or succinate Sulphur centre for e- transport. dehydrogenase complex: Transfers e- from succinate to Cytochrome (Fe ion) centre for e- Catalyzes the transfer of e from - transport NADH to CoQ (Ubiquinone) CoQ. no energy is lost in Transfers e- from ubiquinol (reduced form of ubiquinone (CoQH2 or this process, and no UQH2) to cytochrome C H+ is pumped at Free Energy is released as electrons Energy Release passed from complex to complex. The free energy released is used to pump protons (H+) across membrane. 3 sites of proton pumping when ѐ enter from NADH so 1NADH yields 3ATP 2 sites of proton pumping when ѐ enter from FADH2, 1 FADH2 yields 2ATP Electron transport tightly coupled to proton pumping. 4 H+ = 1 ATP 10H+ = 2.5 ATP (3) from 1 NADH The transfer of electrons down Oxidative Phosphorylation the ETC is energetically favored because NADH is a strong Electrons from NADH/FADH2 passed to the electron electron donor and O2 is an avid electron acceptor transport chain, oxygen is final acceptor of electrons As electrons passed down complexes on chain energy released Released energy used to pump H+ across the membrane This establishes a proton gradient across the inner mitochondrial membrane Protons are moved back into the mitochondrial matrix through ATP synthase ATP synthase makes ATP from energy of H+ moving through H+ channel Chemiosmotic Hypothesis a.k.a Mitchell Hypothesis Describes how ATP is generated from the free energy released from the transport of electrons in the ETC. Step 2 2 steps: 1. Protons pumped from: mitochondrial matrix intermembranous space 2. The proton gradient formed is used for ATP synthesis Step 1 Chemiosmotic Hypothesis Step 1: The sites of proton pumping = 3 complexes of ETC The inner mitochondrial Low [H+] membrane is impermeable to protons, thus when protons are pumped out a gradient is created. High [H+] PROTON MOTIVE FORCE Chemiosmotic Hypothesis Step 2: Using H+ gradient to drive ATP Step 2 synthesis H+ move back to the mitochondrial matrix through specific proton channel. Complex V: Fo unit - integral membrane protein that contains the proton channel, attached to F1 unit (ATP synthase). H+ passage through F0 causes rotation. F0 Rotation conformational change F1 allowing binding of ADP + Pi Summary-Electron transport & oxidative phosphorylation 4 3 Oxidative Phosphorylation Summary- Electron transport & oxidative phosphorylation 4 3 Oxidative Phosphorylation ATP production(Aerobic)- Glucose complete oxidation Reaction path Intermediate ATP Glycolysis 2 ATP 2 2 NADH +H+ 6 Pyruvate Acetyl CoA 2 NADH +H+ 6 TCA cycle 6 NADH +H+ 18 2 FADH2 4 2 ATP 2 Total (max) 38 /glucose 3 molecules of ATP per NADH+H+ - electrons from NADH enter at complex 1 2 molecules of ATP per FADH2 – electrons from FADH2 enter at complex II Conversion in the electron transport chain 1 glucose molecule results in either 36 ATP (glycerol-P) or 38 ATP (malate-aspartate) depending which shuttle system is used to transfer e from the NADH generated in glycolysis into the mitochondrion Uncoupling Proteins Location: inner mitochondrial membrane Create a “proton leak” Energy is released as heat (non-shivering thermogenesis) Thermogenin (UCP1) responsible for heat production in brown adipose tissue. Brown fat uses 90% respiratory energy for heat production in response to cold. (non- shivering thermogenesis) Humans have little brown fat except for neonates. Uncoupling oxidative phosphorylation Compounds that increase the permeability of the inner mitochondrial membrane - No ATP synthesis - energy is released as heat. Exogenous (synthetic) uncouplers include – 2,4 dinitrophenol; pentachlorophenol (a pesticide) – Aspirin(Salicylate) overdose – leads to fever healthgiants.com Endogenous uncouplers include Hyperthyroidism – Bilirubin at high concentrations Can cause severe brain damage in infants (kernicterus) – Excess free fatty acids at high concentrations – Thyroid hormones (T3) - (1) by sympathetic stimulation, – (2) by increasing acylcarnitine production, thereby activating mitochondrial respiration/uncoupling, and – (3) by directly stimulating the transcription of UCP1 Heat intolerance Inhibition of any complex of ETC leads to inhibition of Inhibition of ETC ATP synthesis & cell death. Toxins Doxorubicin inhibits at CoQ Rotenone (poison) inhibits Complex I. CN- and CO inhibit Complex IV – compete with O2 for Revision exercise binding Oligomycin: This drug binds to the Fo domain of ATP synthase, closing the H+ channel Deficiencies Fe deficiency inhibits any of the Fe-containing complexes Deficiency in vitamins B2 or B3 - Severe lethargy, https://www.ijsr.net/archive/v10i11/SR211106093603.pdf complications affecting the cardiovascular, nervous, muscular & gastrointestinal systems. Niacin (Vitamin B3) is a precursor for NAD, which is a coenzyme for Complex I. Riboflavin (Vitamin B2) is a precursor for FAD and CO FMN, its deficiency inhibits at Complex I poisoning Mitochondrial Diseases “Oxphos” diseases result from mutations in genes encoding proteins of the various complexes Mutations could be in nuclear or mitochondrial DNA (so “mitochondrial disease” does not necessarily exhibit “mitochondrial inheritance”) Tend to affect tissues with highest energy demand: nervous tissue, kidney, heart, skeletal muscle... Common disorders – Parkinson's – Cardiomyopathies – MELAS (mitochondrial encephalopathy, lactic acidosis and stroke-like episodes) Progressive neurodegeneration Repeated episodes of lactic acidosis and myopathy Cells often contain mutant and wild-type mtDNA and expression is variable Mitochondrial Diseases Leber hereditary optic neuropathy (LHON) Caused by mitochondrial inheritance four times more males are affected than females Point mutations in one of the subunits for NADH-Q reductase, QH2 (I), cytochrome c reductase (III) or cytochrome oxidase (IV). Sudden onset of blindness in young adults.(patient’s 20s or 30s) Blindness - Bilateral central vision loss caused by retinal detachment Result: Impaired e- flow through respiratory chain & ATP synthesis. The optic nerve has high energy demand & relies almost entirely on oxidative phosphorylation. Summary Acetyl CoA from various cellular sources enters the TCA Revision exercise and generates reducing equivalents in the form of NADH + H+ and FADH2 Electrons pass down the electron transport chain, resulting in the generation of a proton gradient Protons travel down the gradient through the ATP synthase, generating ATP. Additional Resources Reading: Lippincott’s 6, 9 Meisenberg and Simmons Ch. 21 Watching: Electron transport chain video ATP synthase video