FEG-302-Food Storage Engineering.pdf
Document Details
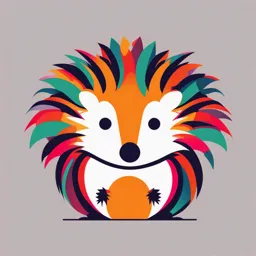
Uploaded by EnrapturedHeptagon
Harrow International School Bengaluru
Tags
Full Transcript
FEG 302 Food Storage Engineering 3 (2+1) (02.12.2019 to 25.04.2020) Theory Syllabus Storage: Importance of scientific storage systems, postharvest physiology of semi‐perishables and perishables, climacteric and no...
FEG 302 Food Storage Engineering 3 (2+1) (02.12.2019 to 25.04.2020) Theory Syllabus Storage: Importance of scientific storage systems, postharvest physiology of semi‐perishables and perishables, climacteric and non-climacteric fruits, respiration, ripening, changes during ripening, ethylene bio-synthesis; Damages: Direct damages, indirect damages, causes of spoilage in storage (moisture, temperature, humidity, respiration loss, heat of respiration, sprouting), destructive agents (rodents, birds, insects, etc.), sources of infestation and control; Storage structures: Traditional storage structures, improved storage structures, modern storage structures; Farm silos: Horizontal silos, tower silos, pit silos, trench silos, size and capacity of silos; Storage of grains: respiration of grains, moisture and temperature changes in stored grains; conditioning of environment inside storage through ventilation; Aeration and stored grain management: purposes of aeration, aeration theory, aeration system design, aeration system operation; Storage pests and control: Damage due to storage insects and pests, its control, seed coating, fumigations, etc.; Damage caused by rodents and its control; Storage of perishables: cold storage, controlled and modified atmospheric storage, hypobaric storage, evaporative cooling storage, conditions for storage of perishable products, control of temperature and relative humidity inside storage; Design of storage structures: Functional and structural design of grain storage structures, pressure theories, pressure distribution in the bin, grain storage loads, pressure and capacities, warehouse and silos, BIS specifications, functional, structural and thermal design of cold stores. Reference: 1. Amalendu Chakraverty, Arun S. Mujumdar G. S. Vijaya Raghavan and Hosahalli S., (2003) Handbook of Postharvest Technology Cereals, Fruits, Vegetables, Tea, and SpicesRamaswamy., Marcel Dekker, Inc. 2. K.M Sahay and K.K.Singh, 2015, A Text Book Unit Operations of Agricultural Processing, Vikas Publishing House, Noida. 3. M. Shafiur Rahman (2007) Handbook of Food Preservation, CRC Press, Boca Raton. 4. Ojha T.P. and Michael A.M., 2012. Principles of Agricultural Engineering Vol-I. Jain Brothers, New Delhi. Lecture Note 1 Storage - Definition, Importance of scientific storage systems Introduction: Food storage is the phase of post-harvest system of raw-material and/or processed product during which produces are kept safe for further use. Food is stored by almost every human society and by many animals. Stored food guarantees food security during off season. Food storage is both a traditional domestic skill and is important industrially Purpose of Food Storage: For distribution to consumers To enable a better-balanced diet throughout the year Reduces waste by preserving unused or uneaten food To preserve pantry food, such as spices or dry ingredients for eventual use in cooking As preparedness for emergencies For religious reasons To protect from animals or theft Objective of Storage Food level - to permit deferred use (on an annual and multi-annual basis) Agricultural level - to ensure availability of seeds (crop cycles) Agro-industrial level - to guarantee regular and continuous supplies (raw materials for processing) Marketing level - to balance the supply and demand of agricultural products (stabilizing market prices) Importance of scientific storage system Background: More than 820 million people in the world every one in nine person — were hungry in 2018, according to United Nation report. Around 700 million faced severe food insecurity the same year, added the report titled The State of Food Security and Nutrition in the World. Approximately 14% of the world’s food is lost between harvest and retail sale, according to the 2019 ‘State of Food and Agriculture’ (SOFA) report. SOFA 2019 identifies “critical loss points” across the food supply chain, explained as points where food losses have the highest magnitude, the greatest impact on food security, and the largest economic dimensions. It finds that harvesting is the most frequently identified critical loss point for all types of food. Inadequate storage facilities and poor handling practices are also main causes of on-farm storage losses. For fruits, roots and tubers, packaging and transportation also appear to be critical. Critical loss points can inform measures to reduce both loss and waste. On loss, the report finds that in lower-income countries, fresh fruit and vegetable loss is attributed to poor infrastructure more than in industrialized countries. For instance, many lower-income countries lose large amounts of food due to poor storage facilities, like warehouses without refrigeration. In the case of high-income countries, even though they possess adequate storage facilities, losses occur during storage largely because of a technical breakdown, poor management of temperature, humidity or overstocking Post-harvest losses account for about 10% of total food grains due to unscientific storage, insects, rodents, micro-organisms etc., In India, annual storage losses have been estimated 14 million tonnes worth of Rs. 7,000 crores in which insects alone account for nearly Rs. 1,300 crores. General estimations have showed that nearly 60 to 65 million tonnes of fruits and vegetables are produced in India. Out of this, 20 to 35 per cent is lost due to lack of proper storage facilities Indian farmers incur Rs. 92,651 crore per year in post- harvest losses, the primary causes of which are poor storage and transportation facilities. Ironically, according to the high-level Dalwai committee report, an investment of Rs 89,375 crore—a figure marginally lower than the annual post- harvest losses—is all it takes to improve the state of storage and transportation facilities for food crops. Considering the statistics and reports, it can be learnt that food production is surplus and shortage of food supply is mainly due to post harvest losses. The principal share of food losses is because of uncontrolled storage conditions. The organizational means of storing foods can be solution to reduce food storage losses by addressing required food storage conditions viz., temperature, moisture, and humidity, etc. In this direction, the course is appreciated to know the scientific principles involved in food storage system. Read more from: 1. https://www.downtoearth.org.in/news/food/every-9th-person-in-the-world-went- hungry-last-year-un-report-65667 2. https://igmri.dfpd.gov.in/igmri/foodgrain-storage 3. https://www.downtoearth.org.in/news/cool-creation-23223 4. https://www.downtoearth.org.in/news/agriculture/farm-income-can-go-up-by-rs- 1-939-crore-on-one-condition-60814 Lecture Note 2 Post harvest physiology of perishables and semi-perishables Physiological status of produce is influenced by preharvest factors, harvesting factors, pest infestation and leads to change in quality, it is important in determining the shelf-life. Physiology is the branch of biology that deals with the normal functions of living organisms and their parts. The scientific study of the physiology of living plant tissues after they have been denied further nutrition by picking/harvest. Physiological stages of agricultural produce/plant tissues Germination Maturation Senescence Growth Ripening Physiological processes affecting storage life Respiration Phyto-hormone Transpiration effects Ripening Physiological Senescence disorders Respiration Respiration is a process in which stored organic materials (carbohydrates, protein, and fat) are broken down into simple end products with release of energy Oxygen is used in this process and carbon dioxide is produced Respiration influences quality of product by: – Reduced food value (energy value) for the consumer – Reduced flavour due to loss of volatiles – Reduced sweetness – Reduced weight Important for the commodities that desire dehydration Classification of fruits and Vegetables based on respiration rate 1. Very low (Rate of respiration less than10 mg carbon dioxide/kg h at 10 0 C) Eg: Nuts, dates, dried fruits, etc. 2. Low (10 mg carbon dioxide/kg h at 10 0 C) Eg: Potatoes, Onions, Cucumbers, etc. 3. Moderate (10-20 mg carbon dioxide/kg h at 10 0 C) Eg: Peppers, Carrots, eggplant, etc 4. High (20-40 mg carbon dioxide/kg h at 10 0 C ) Eg: Peas, Papaya, Fig, etc. 5. Very High (more than 40 mg carbon dioxide/kg h at 10 0 C) Eg: Mushroom, Green Onions, Melons, etc Factors affecting respiration Temperature Relative humidity Gas composition in the ambient and with in the cell Moisture content of the tissue Wounding or injury Type of the plant parts Stage of development of tissue Surface area to volume of the produce Pre-harvest treatments and pH methods employed Chemical composition of tissue Size of the produce Presence of natural coating on the surface Transpiration: The process where plants absorb water through the roots and then give of water vapour through pores from aerial parts especially leaves. This loss of water from fresh produce pose problem leading to weight loss and shrinkage of produce. Factors affecting transpiration Skin structure Size, shape, and surface area Water vapour pressure difference Air movement Heat of respiration Level of maturity Endothermic effects of evaporation Number of solutes present in the produce Ripening- Process that promotes efficient seed dispersal with developing distinct colour, aroma, flavour and succulence to become palatable to animals. Changes during ripening: Increase in pectinase, carotenoids, ethylene Decrease in phenolic acids, flavonoids, chlorophyll, organic acids Loss of chlorophyll and synthesis and accumulation of pigments Reordering of existing metabolic pathways Production of volatiles, alcohol esters synthesis Change in colour, taste, flavour, texture and palatability Ethylene Ethylene is phytohormone responsible for ripening process. Storage life of the produce can be enhanced by reducing the rate of ethylene production. Later it is required to understand rate of ethylene production in different produce and biosynthesis of ethylene, thus it will be probable to control ethylene production. Classification of produce based on ethylene production rate at 20 0 C 1. Very low (less than 0.1 µL/kg h) Eg: Artichoke, Asparagus, Pomegranate, Leafy vegetables, etc. 2. Low (0.1-1.0 µL/kg h) Eg: Cucumber, Brinjal, Okra, Olive, Pineapple, etc. 3. Moderate (1.0-10.0 µL/kg h) Eg: Banana, Fig, Guava, Melon etc. 4. High (10.0-100.0 µL/kg h) Eg: Apple, Papaya, Cantaloupe, etc 5. Very-high (more than 100 µL/kg h) Eg: Passion fruit, Sapota, etc Adverse effects of ethylene: Woodiness, bitterness, sprouting, yellowing, softening, off- flavour, decay induction Ethylene biosynthesis Ethylene, a gaseous plant hormone, influences plant growth, development, and response to various stresses and pathogen infection Ethylene is synthesized from S-adenosylmethionine (SAM) via 1- aminocyclopropane-1-carboxylic acid (ACC) ACC synthase (ACS) and ACC oxidase (ACO) --- key enzymes in the ethylene biosynthetic pathway, are tightly regulated both transcriptionally and post transcriptionally to modulate ethylene biosynthesis Senescence: Biological Aging is the gradual deterioration of functional characteristics Climacteric and Non-climacteric fruits Climacteric Fruit Non-climacteric Fruit Normally they ripen after harvest Fruit that does not ripen after harvest. Ripen on the plant itself. The quality of fruit changes The quality doesn’t change drastically after harvest significantly after harvest except little characterized by softening, change softening. Do not change to improve in colour and sweetness (except in their eating characteristics avocado, which will ripen only after detached from the plant) Exhibits a peak in respiration Does not exhibit a peak More ethylene is produced during Little / No ethylene production ripening Significant increase in CO2 No significant increase in CO2 production production Decrease in internal oxygen More concentration Low concentration of ethylene 0.1- Not much response is seen to 1.0 µL/L/day is sufficient to hasten exogenous application of ethylene. ripening Changes in rate of respiration and ethylene concentration in climacteric and non- climacteric fruits In non-climacteric fruits rate of respiration and ethylene concentration decreases with physiological stages whereas in climacteric fruits rate of respiration and ethylene concentration increases after maturation stage reaching peak in ripening stage and decreases before senescence. Thus, it is significant to control rate of respiration and ethylene concentration during ripening stage in case of climacteric fruits to enhance storage life. For more information: 1. Amalendu Chakraverty, Arun S. Mujumdar G. S. Vijaya Raghavan and Hosahalli S., (2003) Handbook of Postharvest Technology Cereals, Fruits, Vegetables, Tea, and SpicesRamaswamy., Marcel Dekker, Inc. pp 455-484 2. M. Shafiur Rahman (2007) Handbook of Food Preservation, CRC Press, Boca Raton. pp. 19-48 Lecture Notes 3 Damages: Direct damages, indirect damages, causes of spoilage in storage Damage is a physical harm that impairs the value, usefulness, or normal function of food commodity. It is a clear deterioration in the product. Direct Damages (General damages): Harm due to physiological process, storage condition and/or pests (Due to transpiration, moisture losses and there will be loss of weight) Loss of weight Dormancy Nutrients degradation/loss Contamination with foreign bodies Damage of storage structure Indirect damage (Consequential damages): Harm due to consequence of general damages. (Pest infestation is direct damage, infested pest distribution of parasite of man is indirect damage) Create heating and/or migration of moisture May create distribution of parasites to man Causes customer’s resistance/repulsion Types of damages in Agricultural produce: Factor Mechanism Stage Affected Resulting Loss Mechanical Rupture Harvest Moisture loss Bruising Harvest, Transport, Storage Access to pests and diseases Crushing Transport, Storage Total loss Physiological Transpiration All stages before processing Water loss Respiration Dry matter loss Sun scorch In field after lifting Tissue degradation Greening Toxins (potatoes) Chilling Cold storage Loss of palatability Inversion of starch End of dormancy Increased transpiration and respiration Sprouting Storage Pathogenic Necrosis and tissue Storage Downgrading bacteria & degradation fungi Insect Storage (fresh or processed Access for decay infestation products) organisms Rodent & Pecking Storage Access for decay bird damage organisms Causes of spoilage in storage: Shelf life life/storage life of commodity is influenced by pre-harvest factors, harvesting factors, post-harvest handling practices and storage conditions. (Pre-harvest factors viz package of agricultural practices, cultivar, genetic variation, type of soil, type of irrigation method, cropping system, fertigation, etc., harvesting factors viz., harvesting stage, harvesting indices, harvesting method and harvesting time, post-harvest handling includes pre-cooling, curing, chemical treatments, transportation system, and storage conditions viz temperature, humidity and aeration, etc.). The storage conditions are discussed in detail. 1. Moisture Moisture may change during storage due to breakdown of some packaging materials (paper degradation and metal rusting) 2. Temperature Arrhenius equation The optimal temperature range is in the cool to moderate range Grain germination rates will decline as the temperature increases Chemical constituent’s breakdown rates increase as the temperature increases Canned foods should not be allowed to freeze (Freezing will bulge cans and may cause seam failures) Dry foods can be frozen 3. Humidity Humidity in the storage environment should be low otherwise moisture absorbs from the storage area leads to molds and bacteria growth 4. Transpiration and Respiration loss Transpiration is the process by which fresh fruits and vegetables lose moisture Moisture loss is driven by a difference in water vapour pressure between the product surface and the environment Respiration is the chemical process by which fruits and vegetables convert sugars and oxygen into carbon dioxide, water, and heat 5. Heat of respiration is due to the respiration process which releases energy in the form of heat. Losses due heat due to respiration can be reduced either by decreasing the rate of respiration and/or by aerating the structure to release produced heat 6. Sprouting: Sprouting is the physiological process where in seeds initiate to grow. Sprouting of stored produce is undesirable, as sprout contain more moisture making produce perishable. Produce can be stored in lower temperature, lesser humidity and dark place to reduce sprouting. Chemical treatments, irradiation, and controlled atmospheric storage are also proven to be significant in controlling sprouting in stored produce. Read More from: K.M Sahay and K.K.Singh, 2015, A Text Book Unit Operations of Agricultural Processing, Vikas Publishing House, Noida. Lecture Note 4. Destructive agents (rodents, birds, insects, etc.), sources of infestation and control, factors responsible to enhance infestation Pests A destructive insect or other animal that attacks crops, food, livestock, etc. Pests gain access to the grain storage from the standing crop in the field to various stages of grain processing and storage Majority of insect pests belong to the orders Coleoptera and Lepidoptera Stored grain insect pests can be categorized – on the basis of their feeding behaviour--------Internal and External feeder – Major and Minor pests based on the severity of damage they cause Classification based on feeding behavior External feeders – Group of insects feed on germ and endosperm from outside – May attack whole seed and damage the germinal portion or feed on the seeds, which have already been damaged/ infested by other insects or broken mechanically – Insect pests or their stages are generally visible among the seeds Internal feeders – Insects mostly lay eggs inside or on the surface of grains, spend a part or entire larval and pupal life inside the grains and only emerge as adults Secondary feeders Contribute significant loss of germination which is not detectable outside Insects and mites which develop after the infestation of other pests as they feed on cut and broken seeds, moulds and detritus, dead insects, animal wastes e.g., Common grain mite, Cheese mites, etc. Damage by insects result in Loss of germination Contamination like webbing and ball formation Fungal activity Hotspot formation Moisture migration Insects, mites and pests attack both the stored material and wooden components of the storage structure Weevils are the commonest insects in grains Attack seeds and bore through them, and lay eggs in the seeds and storage structures Reduce seed weight, quality, nutritional value and viability Some of the common storage pests Rice weevil Sitophilus oryzae Lesser grain borer Rhyzopertha dominaca Khapra beetle Trogoderma granarium Red flour beetle Tribolium castaneum Cigarette beetle Lasioderma serricorne Drug store beetle Stegobium paniceum Pulse beetles Callosobruchus spp. Angoumois grain moth Sitotroga cereallela Rice moth Corcyra cephalonica Saw toothed grain beetle Oryzaephilus surinamensis Long headed flour beetle Latheticus oryzae Almond moth Cadra cautella Rice Weevil: Sitophilus oryzae (Coleoptera: Curculionidae) Pests of paddy, wheat, millet, barley, maize, sorghum and other cereals Hot and humid climate favour its development In case of infestation, only pericarp of the kernel is left behind, while rest of the mass is eaten up Grain with emergence hole becomes quite hollow and can float in water Completes its life cycle within a month at 28 ± 1°C and 70% RH Severely damaged lots of grains resemble mouldy grains Lesser grain borer Rhyzopertha dominaca (Coleoptera: Bostrychidae) Second in importance to rice weevil as destroyer of the stored grains It is mostly found in warmer regions of the world and damages wheat, barley, maize, paddy, sorghum and other products Profuse powdery substance is the characteristic of its damage Khapra beetle Trogoderma granarium (Coleoptera: Dermestidae) It prefers low humidity and high temperature Serious pest of wheat but can also damage jowar, rice, maize, sorghum, oilseeds and pulses Under abnormal conditions, larva can survive without food for few years Red flour beetle Tribolium castaneum (Coleoptera: Tenebrionidae) Pest of flour mills, feeds on cereals, flour, starchy material, fruit nuts, millets and prepared cereal foods Usually feed on broken grains and results in dust formation Infested flour emits sour and pungent smell, which is due to some secretions of beetles Cigarette beetle: Lasioderma serricorne (Coleoptera: Anobiidae) It feeds on a large number of food varying from spices, chocolate, cocoa and tobacco leaves Insect prefers higher temperature. Drug store beetle Stegobium paniceum (Coleoptera: Anobiidae) It is distributed in tropical, subtropical and temperate regions Primary pest of turmeric, ginger, pepper, coriander seeds, cumin seeds, etc. Adults and grubs feed/ destroy the grains/ seeds Pulse beetle Callosobruchus maculatus (Coleoptera: Bruchidae) Important pest of grain legumes both in storage and field. attacks peas, chickpea, pigeon pea, black gram, horse gram, cowpea etc. Seed germination is spoiled Saw toothed grain beetle Oryzaephilus surinamensis (Coleoptera: Silvanidae) Generally associated with starchy food and found in warm places Larvae feed on endosperm of broken grains or starchy food. Rice moth Corcyra cephalonica (Lepidoptera: Pyralidae) Distributed in all rice growing areas of the world and serious pest of stored paddy, rice and other cereals Flourishes well in humid climates and also attacks wheat, maize, sorghum, barley, millets, soybean and oilseeds Rodents Rodents are mammals that parasite on stored materials and attack storage structures Eat germs of grains and waste the remaining parts Vectors Contaminate stored materials with their faeces, urine and carcasses Birds/avian Reduce standing water Clean areas Never feed pest birds Trim/prune trees away from structures Add obstructions to edges Clean gutters Netting Spikes or porcupine wire Traps Nest removal Professional Control Sources of infestation 1. Field May attack the crop in the field itself Grains affected by these insects when brought to the storage centres will be subjected to their continuous attack May be visible or invisible Preventative measure: Stocks should be fumigated immediately on receipt. 2. Gunnies The insects hiding in the seams of the gunnies will attack the freshly harvested stocks Preventative measure: Gunnies should be fumigated before packing the freshly harvested grains 3. Transport Carrying infested stocks on the previous occasion, the leftover insects may attack the stocks now loaded into them. Preventative measure: The transport used for carrying the newly harvested stocks should invariably be sprayed 4. Godown Insects present in the cracks and crevices of the wall or that hibernate in the structures, may emerge out and attack the grains Preventative measure: Cracks and crevices should be plugged Empty godown should be thoroughly cleaned and fumigated 5. Stocks Cross infestation takes place Preventative measure: First in first out (FIFO) Monitoring the stocks regularly Factores influencing Storage life of commodity The produce of lesser moisture content stored at lower temperature in dry environment has longer storage-life. Lecture Note 5 Traditional Storage Structures Traditional storage structures are either aerial or constructed using plant materials, soil or mud. 1. Aerial structure: Bundles of grain panicles, maize cobs, onion and garlic bulb are suspended in a well-ventilated part of the house or above fireplace where insects may be less and moisture content of the grain may be reduced. 2. Storage on ground: a. Commodity placed on wattle mat or on floor stabilized by natural materials- this is to reduce the moisture content of commodity. b. Open timber platform: The produce can be contained in earthen wares, wooden containers (eg: Sanduka) or bamboo baskets. c. Others: Gourds after being dried, plant leaves, twigs, roots, straw are used to construct as supporting material and is plastered using mud or dung eg: Bukhari, mud bin, Morai, etc 3. Underground structures: The pit is dig and material is stored underground eg: Hagevu Traditional types of storage structures capacities between 1 to 50 tonnes. Eg Morai, Bukhari, Kothar, Mud Kothi, Muda, Kanaj, Kuthla, etc. Morai Morai type of structure is used for the storage of paddy, maize and sorghum (jowar) in the rural areas of eastern and southern regions of India. Its capacity varies from 3·5 to 18 tonnes. These structures are very similar to the shape of an inverted cone. They are placed on a raised platform supported on wooden or masonry pillars. The improved type of structure consists of a circular wooden plank floor supported on pillars by means of timber joints. The planks are joined together with lap joints. All around the wooden floor a 22 gauge corrugated metal cylinder of 90 cm height is nailed to it. The edge of the cylinder is flushed with the bottom end of the floor. Inside the cylinder, 7·5 cm diameter ropes made of paddy straw or similar material are placed, beginning from the floor level upto a height of 90 cm. Then bamboo splits are placed vertically along the inner surface without leaving any gap between them. The height of the bamboo splits is equal to the total height of the structure. Keeping the bamboo splits in position, the grain is poured in up to the height of the metal cylinder. By then the bamboo splits are held erect in position. Now the winding of the rope as well as the pouring in of grain are done simultaneously. This process continues till the required height is attained. The top most ring of the rope is secured in position by tying to the lower four rings. To provide a smooth surface, about 1 cm thick layer of mud plaster is applied over the rope. A conical roof is placed on the top of the structure having an ample overhang all around. Bukhari Bukhari type storage structures are cylindrical in shape and are used for storage of sorghum, wheat, paddy, Bengal gram, maize etc. Bukhari structures generally have capacities between 3.5 to 18 tonnes, however, smaller capacity structures also exist. This may be made by mud alone or by mud and bamboo. The cylindrical storage structures are raised above the ground by wooden or masonry platform. The floor of the bin is made either by timber planks or by bamboo splits, plastered over with mud mixed with dung and paddy straw. The walls of the structure are made of timber or bamboo frame work and bamboo matting. Over the walls, mud-straw plaster is applied on both sides. An overhanging cone type roof is provided on the cylindrical structure. The roof is generally made of bamboo framework and straw. In improved bukhari type structure, the basic shape remains the same but the material and method of construction have been improved to make the structure safer and more durable. The circular floor of structure is either made of wooden planks joined by lap joints or by a double layer of bamboo splits closely set at right angles to each other. Over the floor, about 5 cm thick mud plastering is provided. The walls of structure are made of two sets of strong bamboo framework. The inter-space is filled with mud. The walls on both sides are plastered with mud. The roof is conical and made of bamboo frame-work and covered with paddy straw or similar other thatching material. The top of the conical roof is covered with 4 to 5 cm thick mud layer to provide additional protection from rains. The structure is raised on timber or masonry pillars to a height of about 1.5 m from ground level. Rat proofing cones are placed on all the four pillars to avoid rats entering the storage structure. Kothar These are used to store paddy, maize, sorghum, wheat etc. Their capacity varies between 9 to 35 tonnes. The storage structure is box like made of wood and raised on pillars. Both the floor and walls are made of wooden planks whereas the thatched or tiled roof is placed over it to protect the grains from the sun or rain. The improved Kothar structure is generally made of 5 cm thick wooden planks and beams. The walls and floor are made in such a way that no gap exists between the planks. The gabled roof on the top may be made of planks or corrugated metal sheets and should be sufficiently overhang on all sides. The storage structure is raised on timber post to a height of about 1.5 m above the ground. Rat proofing cones are provided on all posts to avoid entry of rats in the structure Mud Kothi (Mud bin) These storage structures are quite common in rural areas for storage of grains and other seeds. The capacity of such storage structures varies from 1 to 50 tonnes. These are made from mud mixed with dung and straw. These Kothies are generally rectangular in shape but cylindrical Kothi is also common in some region. There are many sizes and dimensions of Kothi made for storing grains. Muda type of storage Structure These are in use for storing grains in the rural areas of Bihar. The capacity of muda varies between 1 to 3 tonnes. It is being made of "Narai" ropes. The shape of muda is cylindrical and being made in various sizes. Kanaj These storage structures are very common in the rural areas of Karnataka and Maharashtra for storage of grains. The capacity of Kanaj varies between 1 to 20 tonnes. It is being made by bamboo splits. The shape of storage structure is cylindrical. The walls of storage structure are sealed with mud plaster on both sides. The roof of the structure is conical and thatched. The roof overhang on all sides. Kuthla These storage structures are very much common in rural areas of Bihar and Uttar Pradesh. These structures are kept inside and made of burnt mud. SWOT Analysis of Traditional Storage Structures Strengths: Utilization of farm waste Low-cost Easy to construct and less time involved in construction Independent ownership Weakness: Temporary, less capacity Cannot withstand adverse weather conditions Opportunities: Improving the construction with technical support Enhancing employability of rural youth by training construction and maintenance Improves revenue Threats: Flammable Prone to pests and theft Reference: K.M Sahay and K.K.Singh, 2015, A Text Book Unit Operations of Agricultural Processing, Vikas Publishing House, Noida. pp 169-170 Lecture No. 6 Improved Storage Structures Improved storage structures are the storage structures for storage of food grains. In this type of storage structures there are some improvements made in traditional storage structures. This type of storage structures having a higher storage capacity and long-term storage of food grains than traditional storage structures. These storage structures having capacities is generally 1.5 to 150 tonnes. The storage of grain is generally done in one of the following storage structures in the different rural and urban regions of India in bulk, bag as well as bag and bulk storage. Pusa Bin This is made of mud, to make it moisture proof, a plastic film is used on inner side of the bin. A platform of mud bricks is made, first. On this platform, a sheet of 700-gauge plastic is spread in such a way that it overlaps the platform on all sides by atleast 6 cm. On the plastic sheet, a layer of 7 cm thick kachcha bricks is then laid. Walls are made of kachcha bricks and these are sealed with mud plaster. Now the walls are raised to proper height and a wooden frame is placed on it. The upper roof of the structure is made of burnt bricks. For unloading of grains, an inclined wooden or steel pipe is fixed in such a way that grains may come out of structure by gravity. The mouth of pipe is closed by a cover. The inside of all the four walls and roof are covered with a plastic sheet. On the top, an open space of about 50 cm x 50 cm is left for loading of grains. Leaving this open space, the roof is sealed by mud. After the bin is filled with grains, the top open space is well covered by a plastic sheet so that air may not enter the bin. Brick and cement bin (Grain House) These storage structures are very strong and therefore, the effect of season on them is negligible. The bin is made on a platform raised at 60 cm above the ground. A ladder is provided on one side of the bin for loading of the grains. A hole of about 60 cm diameter is provided on the roof for the purpose of loading the material i.e. grains. The walls of bin are about 23 cm thick with cement plastered on both the sides. Roof is made of R.C.C. The base of bin is made inclined and an outlet is provided for unloading of grains. The capacity of such bin is usually between 1.5 to 60 tonnes. For cleaning of bin and complete unloading, a provision of iron rings steps is provided inside the bin for person can enter and exit the bin. Bunker type Storage Bunker storage structure is used for long term storage of a larger volume of grains. The structure is successful as a means of storing grains safely, securely and economically. By controlling insects and the moisture, the losses in stored grains can be reduced upto 0.5%. In this type of storage structure, the grain is stored on a plastic sheet which is spread over ground and top covered with plastic sheet. A drain is also provided for drainage of rain water. 1. Trench for burying cover, 2. Plastic ground sheet, 3. Top cover, 4 drain Cover and Plinth Storage (CAP Storage) The word plinth is means plinth from the bottom and cover means cover from the top. This type of open storage is considered as intermediate storage and serves the purpose of storage of food grains in bags for short period. This type of storage facility is cheaper as compared to conventional bag storage godowns. The cover is rectangular in shape having five sides and made from polyethylene film of 1000 gauge, leaving the bottom side open. The cover is used for protecting stack of bags. Normally the stack is built over a space of 9.11 x 6.1 m with a height of 18 bags which gives the storage capacity of around 150 tonnes. The cover having a dimension of 9.4 m x 6.4 m x 5.5 m normally weighs around 52 kg. Sometimes smaller covers are used for covering the stacks in covered varandah of conventional godowns. Such covers are called "Varandah covers". For storage of food grains under varandah covers, the stacks are built to a height up to 7 bags having an average capacity of 24 tonnes. The following steps are normally followed in the construction of a 'CAP' storage (i) Select a high elevated ground and make it level. (ii) Wooden sleepers are spread with one or two layers of bamboo mat cover on the top as dunnage. (iii)The Gutters are provided all around the area to drain off rain water easily. (iv) The stacking is done to the height upto 18 bags on the dunnage and is covered with polyethylene. (v) The stacks arc covered with polyethylene covers and tied with ropes to prevent from blowing off with high velocity wind. Bag Storage Structure These structures are generally used for the storage of 25 to 500 tonnes of grain. The length of the structure is about twice the width or greater than that. A typical floor plan of such a structure large enough to store about 6000 bags (500 tonnes) of grain. Bags of different capacities (35, 50, 75 and 100 kg) with or without inside plastic lining are used now a days as improvised version of traditional bagging system. The standard size of a 100 kg bag is 100 cm x 60 cm x 30 cm i.e. length of bag is 100 cm, width of bag is 60 cm and height of filled bag is 30 cm. This bag can store 93 Kg of Wheat and 75 Kg of Paddy. Metal Bin Bins made of steel, Aluminium R.C.C are used for storage of grains inside and outside the house. These bins are fire and moisture proof. The bins have long durability and produced on commercial scale. The capacity ranges from 50 kg to 10 tonnes. References: K.M Sahay and K.K.Singh, 2015, A Text Book Unit Operations of Agricultural Processing, Vikas Publishing House, Noida. pp 170-172 Lecture No.7 Modern Storage Structures Modern storage structures are the structures of increased durability, higher capacity and fulfilling most of the ideal requirements* *Requirements of an Ideal Storage Structure Easy to clean Protection from rodents, birds and other animals Protection against variations of temperature and humidity Provision for periodical inspection Provision for application of pesticides through spraying or fumigation Away from possible sources of infection Convenient & easy to receive and transport All holes, pipes and ducts and other openings shall be guarded Shall have smooth, crack free internal surfaces and shall have no unnecessary cavities and projections Designed to facilitate its sealing for fumigation or have facility to seal a portion where fumigation has to be carried out, or it may be made completely airtight if required Godowns should have good ventilation arrangement to prevent moisture accumulation in pockets Shall be designed to make it possible to control moisture using non-hygroscopic material, by sound wall, roof and floor construction, by the use of vapour barriers, and by the use of aeration Shall be so oriented that it will receive the minimum solar radiation. Reflective external surfaces, insulating materials, sun shades, a minimum of glass surfaces Controlled ventilation and aeration, to reduce the internal temperature may be used Able to extend the facilities and control the storage atmosphere Modern Storage structures are either of silo type or shed type Silo type of storage structures The modern facilities for storing grains in bulk are 'silo'. Silos are constructed from steel or reinforced concrete. There are a cluster of adjoining silos in any modern large capacity processing plant. These silos are generally circular with conical bottom. Bulk storage bins for storing grains can be made from reinforced concrete, plain or corrugated galvanised sheet, mild steel black sheet, aluminium sheet, fibre glass, brick, ferro cement, asbestos sheet etc. But in India mild steel bins and R.C.C. bins are quite common. Silos can be used in storing liquid foods such as milk, oil and brine, etc The advantages of modem storage bins are less expensive and easier handling and quality control lesser space requirement savings of cost of bags provision of automation and mechanization for quicker handling and maintaining quality of stored product protection from losses due to birds and rodents. Silos/bins are classified into two groups depending upon the relative dimensions of the container. To what extent of relative dimensions of depth and diameter or width, the structure behaves as a deep or shallow bin is decided from the concept of the plane of rupture. The following terminology is useful for design of storage structures Plane of rupture is that surface down which a wedge of material bounded by one wall face, the free surface, and the plane of rupture would start sliding if bounding wall were to move. Angle of repose- When a granular product is dumped through a circular opening on a level horizontal surface, the product takes a shape of inverted cone. The angle between the horizontal and inclination of heap is called the natural angle of repose. The angle of repose is an indicator of the product's ability to flow. Angle of rupture- If Φ is the angle of repose of material, then the angle of rupture may be taken with the horizontal. Silo is classified as, (1) shallow bins and (2) deep bins. Shallow bins A bin whose relative dimensions are such that the plane of rupture meets the grain surface before it strikes the opposite side. A grain bin referred to as a shallow bin when the depth of grain is less or equal to the equivalent diameter. The equivalent diameter is taken as four times the hydraulic radius of the bin. Hd < 4R , Where Hd is depth of grain, R is Hydraulic radius. Squat silos are the example of shallow bin. A squat silo has a wall height to diameter ratio 0.5 or even less. Squat silo can compete with sheds for low-cost quality storage. Squat silos are structurally efficient, thus can be built from lighter materials and costs are generally lower for a given storage volume. The structural efficiency is achievable by the walls carrying their loads principally in tension and by the conical roof carrying its load in tension, compression and shear. Squat silos as large as having 48 metres diameter, 10.5 metres high walls and 25 metres high at roof apex are being built. Deep bins A bin in which plane of rupture meets the opposite side before it emerges from the grain. A grain bin referred to as a deep bin when the depth of grain is greater than the equivalent diameter. The equivalent diameter is taken as four times the hydraulic radius of the bin. Hd >= 4R , Hd is depth of grain, R is Hydraulic radius A vertical silo is example of deep bin. Vertical silos can be circular, hexagonal, or rectangular. They tend to be more expensive than sheds. In case of space limitation vertical silos can be used because it requires lesser space. There are two types of vertical silos used i.e. Flat bottom and Hopper bottom vertical silos. Hopper bottom silo is quite common for storing grains. Prefabricated galvanized metal silos are available in many sizes. Flat bottom vertical silos are also common. These may be fabricated by metal sheets, galvanized metal or reinforced. In hopper bottom concrete. The hopper bottom is more expensive than flat bottom bin. Hopper slope angles of 60° - 70° are necessary to achieve reliable mass flow. Shed (Warehouses) Horizontal sheds have been used to provide low- cost, large volume storage. Very large volume sheds have also been constructed by Food Corporation of India (FCI), Central Warehouse Commission and some private organization in PPP model for storing agricultural products. Sheds are usually made of steel or corrugated sheet construction with flat concrete floors. Grain loads on the walls have to be supported by girts and heavy vertical buttresses designed to resist their loads in bending. Roofs have to be supported on a network of purlins and rafters. Belt conveyors can be used in bulk sheds for loading and unloading grains. For larger sheds having 50,000-60,000 tonnes capacity a suspended drag-chain conveyor can be used. A typical bulk warehouse would be 15 m Wide, 60 m long and 6 m high at the edges. A building of this size would have a storage capacity of 2,840 tonnes. The same size building for bag storage has only 1440 tonnes storage capacity. A 5000 tonnes conventional godown will go up about 5750 tonnes capacity by storing grains in bag and bulk combine. there is increase 17% over rated capacity. (1500 tonnes in form of bag and 4250 tonnes in bulk). Wheat can be held in bags under dry climatic conditions for a period up to 2 years. (This period is shortened to 8 to 12 months in humid conditions). Wheat can be kept in bulk stored up to 5 years. Considerations during design of godowns. The godowns side walls are of brick or stone masonry and sloped roofing in asbestos or Corrugated Galvanized Iron (CGI) sheets over steel trusses. Generally, a godown has a capacity of 5000 tonnes and consists of 3 compartments, each having a span of not less than 21·7 m with a clear height of 5·4 m. Air circulation is maintained through steel ventilators and air inlets of rolled steel sections. Requirement of steel and cement is about 50 and 300 MT respectively. Bagged food grains are arranged in stacks with a base of 6 m x 9 m with a stack height varying from 4 to 5 m, leaving 27 per cent free space of the floor area for alleyways generally. It should be leaving a free space of 2 m between the stacks and 0.8 to 1.0 m between wall to stack for easily moving of person for observation. There should be 2.4 x 2.4 m size two large doors of opposite direction and top ventilators are used. Each door is provided with a light overhanging hood of 3.6 m long and 2.4 m wide. A ground ventilator having an opening of 30 x 30 cm is provided below each corresponding top ventilator. The top of ventilator is kept at height of 60 cm above the floor level. It is also provided with iron rods, wire netting and shutter. The thickness of wall is kept minimum of 37.5 cm and maximum of 45 cm. The height of wall on which trusses are placed is generally kept about 5.5 m. The roof is either gabled or flat. The gable roof is covered with corrugated metal sheet with maximum precautions taken to make it leak proof. However, flat roof is more durable as it is made of either reinforced brick or reinforced concrete of about 10 to 12.5 cm thickness. A Numerical regarding Design of Storage Structure Design a bag storage structures for storing 250 tonnes of Paddy. Assume reasonable data where ever necessary. Solution. Design capacity of the storage structure = 250 T = 250,000 kg Capacity of a bag of 100 x 60 x 30 cm = 75 kg of Paddy Hence, number of bags required for storage of 250 T paddy i.e, 250,000 kg of paddy = 3340 bags required for storage of 250 T paddy. Bags are arranged in number of Stacks. Let there be 10 bags in length and 10 bags in width in one stack. So, No of bags/layer = 10 x 10 = 100 If there are 12 layers in a stack, total number of bags/stack = 100 x 12 = 1200 Hence, the number of stacks required = 2.78 ≈ 3 Stacks required for storage of 250 T of paddy Space required by each stack Length of stack l = 10 x 1.0 = 10.0 m Width of stack w = 10 x 0.6 = 6.0 m Height of stack h = 12 x 0.3 = 3.6 m The clear distance between the walls and the end of stack = 0.8 m The clear distance between the stacks i.e. between two stack = 2.0 m Hence, the length of floor L is L = (3 x 6.0) + (2 x 2.0) + (2 x 0.8) = 23.6 m ≈ 24.0 m The width of floor W is W = (10 x 1.0) + (2 x 0.8) = 11.6 m ≈ 12.0 m Therefore, the overall dimensions of the godown may be taken as 24.0 m length, 12.0 m width and the height of the walls may be kept as 5.0 m above the floor level. Reference: K.M Sahay and K.K.Singh, 2015, A Text Book Unit Operations of Agricultural Processing, Vikas Publishing House, Noida. Lecture Note 8 Farm Silos- Pit Silo and Trench Silo Pit silos It is a circular deep well which is lined all along the side and bottom to prevent the water entering it. They can be made of bricks or stores or concrete with either lime or cement. Up to a depth of 15m a 22.5m wall is provided with plastering the entire inner surface. A simple roof over the silo can be provided to protect the silage from sun and rain. Diameter of the silo is determined by the quantity of silage feed daily. The rate removal should at the rate of 10cm/day. The diameter is limited to 6 m and its depth is kept 2-3 times the diameter. Design criteria Rate of removal - 10 cm/day Silage fed per day - 3 kg/100kg of body weight Maximum diameter - 6m Depth - 2-3 times diameter 1m3 of silage - 650 kg Trench silos It can be either lined one or unlined one. Unlined silo is easy to construct but give more spoilage of silage and caving in of sides due to rain. A lined trench silo is advantageous and the lining can be made with brick concrete or cement plaster with reinforcing wire mesh. Filling packing and sealing are similar to pit silo. The drainage water should not be allowed to collect near the trench and so drains should be made around the trench. The cross section depends on number of animals to be fed and the length is determined by the number of days, the silage is fed in a year. Reference: 1. Ojha T.P. and Michael A.M., 2012. Principles of Agricultural Engineering Vol-I. Jain Brothers, New Delhi. Lecture No. 9 Effect of temperature and moisture on stored grain Respiration of Grains Food grains get oxygen from the air and burn food from its endosperm. This process liberates heat, water vapours and carbon dioxide. This process in grains is called respiration. The respiration in grains, during the storage period, causes a dry matter loss of 1% or more. Respiration is a process of slow combustion of carbohydrates in presence of oxygen in living system to produce the energy. C6H12O6 + 6O2 → 6CO2 + 6H2O + Energy This process is affected by temperature and concentration of O2 and CO2. Decreasing the concentration of O2 and increasing concentration of CO2 reduces the rate of respiration. A decrease in temperature slows down the respiration rates in grains like many other foods. Respiration has long been used to measure metabolic activity in stored produce. Its rate is governed by water availability, temperature, oxygen concentration, microbial contamination, mechanical damage, the conditions and period of previous storage and by mites and insect infestation. Microorganisms, especially fungi, are important causes of deterioration and grain also respires but the relative contributions of these two components to total respiration remain controversial. Some studies. using maize with 22-27% water content, have suggested that grain respiration greatly exceeds microbial respiration. While others have found relatively low and constant levels of respiration in mould-free wheat grain at 12-35% water content. Respiration rates may differ with the cultivar, age and quality of grain tested, between different methods of determining respiration, the quantity of grain used and the period of the experiment. Dry matter loss results from the utilization of carbohydrate during respiration. Consequently, respiration data can also be used to measure dry matter loss. Estimates differ as to how much dry matter loss is allowable before grain is rejected. A loss of 0.5% dry matter, sometimes with no visible moulding, was sufficient to render maize grain unfit for use. Considered grain still to be fit for animal feed with dry matter losses up to 2% and decreased germinability, while for seed considered 0.5 % an acceptable or even a small dry matter loss. Dry matter loss up to 1 % over a period of 12 months is acceptable in grain for food use. However, measurement of such small losses directly by weighing is subject to errors because the weight of fungal mycelium on the grain needs to be subtracted from the total dry matter. Moisture and temperature changes in stored grains air movement inside the storage. The migration of moisture in stored grain takes place due to change in temperature as per season i.e. winter and summer. One of the most important factors influencing storage life of the grain is moisture content. High moisture content and a warm climate promote mold growth, insect growth and increase rate of respiration of grains and due to this deterioration and losses of the stored grains takes place. Moisture migration takes place in stored bin even though the grains are stored at a desired moisture level i.e. safe for storage. Air movement and migration of moisture inside storage bin in winter season The grains after harvest in summer is stored in storage bin, grains become warm. When winter season comes, the atmospheric temperature is lowered down. The grain stored in summer is warmer than the atmospheric temperature. In this condition after passage of time the air at the surface of storage bin is cools because the outside temperature is lower than the temperature in the centre of bin due to the grains are warmer. The cool air at inside surface of bin moves down along the edge of the bin, across the bottom and then upward near the centre of the bin, where the air and grain are warm. The cool air moving through the centre of the bin picks up moisture from the grains and moves across the top of the sides. At this location the surface of the grain is cold and the moisture condenses on the grain at top surface and consequently the moisture content of grain at top surface is raised. Because of increased grain moisture, spoilage takes place at top of the bin. Air movement and migration of moisture inside storage bin in summer season In summer season, the atmospheric temperature is higher. As a result, air currents move top to bottom through the centre of the bin because at top of the bin the grain is warmer due to higher atmospheric temperature. The hot air at inside top surface of bin moves down at the centre to the bottom of bin and then upward near the surface edge of the bin, where the air and grain are warm. The hot air moving through the centre of the bin picks up moisture from the cool grains and moves across the bottom of the bin. The moist air condenses due to cool grain and moisture accumulation at bottom. Because of increased grain moisture, spoilage takes place at bottom of the bin. Lecture No. 10 Aeration Aeration preserves the stored grain quality by maintaining uniform and cool temperature. Grain aeration systems are generally designed to carry out either a drying or cooling function — not both Aeration cooling can be achieved with airflow rates of 2–3 litres per second per tonne delivered from fans driven by a 0.37 kilowatt (0.5 horsepower) electric motor Aeration drying can be achieved with fans delivering 15–25L/s/t, typically powered by 7kW (10hp) electric motors Purpose of aeration Preventing mould Inhibiting insect development Maintaining seed viability Reducing grain moisture The advantages of aeration Extends the harvesting window of grain and seed crops reducing delays from rain at harvest and the downgrading of premium grain due to weather damage. Minimizes colour deterioration from delayed harvest of premium products that are paid on the basis of colour, such as barley or pulses. Equalises the silo temperature to minimise hot spots, which directly affect grain quality and provide favourable conditions for insect and mould contamination. Protects high moisture grain from developing moulds in storage. Cools the grain and reduces damage from insect populations in high throughput storage, typically lot feed operations, where the grain is fed to stock and there are limited opportunities for fumigation. Provides opportunities to supply wheat to millers or manufacturers throughout the year. Wheat milled for Flour retains its baking qualities longer when stored cool. Aeration passes ambient (unheated) air through bulk grain. If the air passing through has a relative humidity (RH) that is different to the air surrounding the grain, then moisture moves either from the grain or to the grain until equilibrium is reached. Long term storage of low moisture grain- Low airflow aeration Low flow aeration protects the quality of low moisture grain for many months in storage and has the added advantage of slowing the development of insect populations. To hold low moisture grain in a safe condition for long storage, a fan capacity of about two liters per second per tonne (l/s/t) is usually adequate. Exhaust air must be able to escape freely from the top of the storage. It is recommended for an opening of at least 0.1 m2 for each 500 liters per second of air delivered to the storage. (As a guide, a hole 30 cm by 30 cm is approximately 0.1 m2). Exhaust vents must be designed to prevent water entering the silo. Temperature monitoring and automatic fan control systems are recommended for long-term storage. The controllers select the best quantity and quality of air for use in the system and some controller models prevent high humidity air entering the grain bulk. Short-term storage of high moisture grain- Medium airflow aeration Harvesting grains at high moisture levels, above those suitable for delivery and storage, optimizes quality and minimizes the risk of fungal staining and sprouting and losses due to wet harvest conditions. Medium airflow aeration is needed to preserve the quality of high moisture grain before it is dried in a heated air dryer. Under aeration, the moisture content and temperature of the grain is equalized in the stack. This has the advantage of providing a consistent feedstock for the dryer and reduces the need for frequent changes to the dryer speed and heat settings. The airflow rate depends on the grain moisture content but is typically in the 4 to 10 l/s/t range. To hold the grain in a safe condition, aim for a temperature of 20oC at a maximum moisture content of 15 per cent. When harvesting in the early morning or late evenings the moisture content of each grain may be raised by moisture on or close to the grain surface. Airflow of 4 to 10 l/s/t will remove moisture from the surface region of each grain. Equipment for Monitoring, Management To properly manage stored grain the operator must be able to obtain samples from the stored grain, determine moisture content, monitor grain temperatures, and keep a simple record of both grain and ambient temperatures. A deep bin probe should be used to obtain samples at different locations to determine the moisture content, the level of fine material, and general grain conditions. A reasonably accurate moisture tester is needed. The operator must know the accuracy of the moisture tester under all conditions. Inexpensive electrical testers can give inaccurate readings under many conditions. Readings on freshly dried grain, warm or hot grain, and excessively cold grain can be inaccurate. The operator can calibrate the tester under these conditions by checking readings with the local elevator or other more accurate testers. Thermocouple cables installed in larger bins are valuable in monitoring temperatures in storage to determine the progress of aeration. In bins without cables, thermometer probes should be used to check the temperature at different locations within the bin. This helps in monitoring the progress of the aeration and in locating trouble spots. A thermometer to measure the exhaust air temperature and one to read ambient air temperature is necessary for proper fan management. Maximum-minimum thermometers are especially helpful because they provide the operator an indication of changes in temperature with time. This equipment not only helps the operator manage the stored grain but provides information on how the aeration system works and how stored grain responds to treatment. Natural Ventilation Natural ventilation is the process of supplying and removing air through an indoor space without using mechanical systems. It refers to the flow of external air to an indoor space as a result of pressure or temperature differences. There are two types of natural ventilation occurring in buildings: 1. Wind driven ventilation 2. Buoyancy-driven ventilation While wind is the main mechanism of wind driven ventilation, buoyancy-driven ventilation occurs as a result of the directional buoyancy force that results from temperature differences between the interior and exterior. The static pressure of air is the pressure in a free-flowing air stream and is depicted by isobars in weather maps. Differences in static pressure arise from global and microclimate thermal phenomena and create the air flow (wind). Dynamic pressure is the pressure exerted when the wind comes into contact with an object such as a hill or a building. The impact of wind on a building affects the ventilation and infiltration rates through it and the associated heat losses or heat gains. Wind speed increases with height and is lower towards the ground due to frictional drag. The impact of wind on the building form creates areas of positive pressure on the windward side of a building and negative pressure on the leeward and sides of the building. Thus, building shape and local wind patterns are crucial in creating the wind pressures that will drive air flow through its apertures. In practical terms wind pressure will vary considerably creating complex air flows and turbulence by its interaction with elements of the natural environment (trees, hills) and urban context (buildings, structures). Vernacular and traditional buildings in different climatic regions rely heavily on natural ventilation for maintaining thermal comfort conditions in the enclosed spaces. Building Design Guidelines for Natural Ventilation Structures The following design guidelines are normally practiced from the Whole Building Design Guide, a program of the National Institute of Building Sciences: Maximize wind-induced ventilation by sitting the ridge of a building perpendicular to the summer winds Widths of naturally ventilated zone should be narrow {max 13.7 m (45 feet)} Each room should have two separate supply and exhaust openings. Locate exhaust high above inlet to maximize stack effect. Orient windows across the room and offset from each other to maximize mixing within the room while minimizing the obstructions to airflow within the room. Window openings should be operable by the occupants Consider the use of clerestories or vented skylights. Mechanical Ventilation Mechanical ventilation is the process of supplying and removing air by using mechanical systems. Mechanical Ventilation systems can be installed in both horizontal and vertical storages. The following set of basic design parameters are found suitable for cooling of dry grains by mechanical ventilation. Air flow rate: Silos 0.8 litre/second/tonne, Sheds 1.6 litre/second/tonne Maximum duct velocity: 10 m/ s Maximum entry velocity of air into grain: 0.15 m/ s The most common air flow rates for aerating paddy range from 0.07-0.28 m3/minute/tonne of paddy. From said parameters, fan output, duct size and duct surface area can be determined. Air can be pulled or pushed through the grain in storage. The air can be moved from the top to bottom or from the bottom to the top of the bin. In the tropical climates, aeration should be done from bottom to top because any air trapped under the roof of a storage structure is usually at high temperature. This high temperature air can be forced out easily from the top. The cooler air from bottom also cools the warmer grain stored in bin. The principal parts of an aeration system are, (1) fans to move the air and (2) ducts for carrying air from the fans to storage system. Axial fans are normally used for shed aeration. Non-overloading centrifugal fans should be used for vertical storages because of the higher-pressure requirements. With overloading fan, it is recommended that a manually adjustable throttle valve should be used in the inlet duct to cut back the airflow while aerating partly filled silos. Various configurations of ducting can be used for air distribution inside the grain mass. Ventilation systems for silos are, usually; (1) central vertical system (2) floor ducts and (3) perforated floors Vertical duct system: Top part of the central duct is solid and the lower half section is perforated. A fan at the top of the duct pulls the air through the grain and perforations. The sucked air is blown out of the bin by the fan. On-floor ducting is used, it is often important to allow for its removal to facilitate floor sweeping. For this reason, full round ducting is usually used in sheds where grain loads are moderate. Both longitudinal and cross-floor ducting can be used in sheds. Generally ducting is made from galvanized steel sheet with 2.5 mm perforations at 6 mm centers. Ducting in silos is normally exposed to considerably higher grain pressures. In-floor ducting requires the use of flat perforated sheet. Cost of such system is significantly higher because of requirement of a structural frame below the perforated sheet for its support. An alternative is perimeter ducting formed from quarter round corrugated ducting. Perforated ducts may be circular, semicircular, rectangular or inverted V-shaped. Reference: K.M Sahay and K.K.Singh, 2015, A Text Book Unit Operations of Agricultural Processing, Vikas Publishing House, Noida. Lecture No 11 Pressure Theories in design of Silos The agricultural granular product like cereals which are mostly stored in silo, For the design of silos the knowledge of characteristics of grain like bulk density, internal angle of friction, coefficient of friction on the walls is needed. The walls of the silos have to design by considering self-weight and loads of the grain during construction. The silos walls are subjected to two main forces or thrusts, (1) the horizontal thrust due to loading of material which tries to open up the silo and (2) the vertical pressure which is generated due to loading of material on the walls. The speed of filling of granular material affects the stress development on silo. When the material is filled very rapidly without any compaction other than the compaction produced in the operation, the greatest forces are obtained for the lateral thrust while the apparent density and the angle of internal friction of the material are at minimum values. On the contrary, when the grain is filled at such a slow rate which provides every possibility of compaction, the greatest forces are obtained for the vertical pressure. In this case the bulk density and the angle of internal friction of material are at maximum values. The characteristics values of the granular material which cause greatest stresses should be taken into account while determining the lateral and vertical pressures of storage silo. These values are function of the silo wall nature. For smooth walled silo the greatest vertical and lateral pressure, be estimated with maximum values of bulk density and angle of internal friction while the minimum value of angle of friction of grains on the walls be taken. Whereas for silos with rough walls, the lateral pressure should be calculated with values of minimum bulk density, minimum angle of internal friction and the minimum angle of friction of the grains on the walls. The greatest vertical pressure should be estimated with maximum bulk density, maximum angle of internal friction, and the minimum angle of friction of the grains on the walls. Grain Pressure Theories The grain pressure in bins were first calculated as being a semi-liquid of same density as the grains. The lateral pressure was first calculated using the hydrostatic formula. Pl = wh Where, Pl = lateral pressure exerted by the grain on the bin wall, kg/m2 w = density of grain, kg/m3 h = depth of grain from the top of the bin surface, m This formula has serious deficiencies because many structures buckled under the vertical load arising from the friction of the grain on the walls. The formula (1) was modified to incorporate a factor 'K ' known as Rankine's earth pressure coefficient. The Rankine's formula is based on the following principle, the resistance to displacement by sliding along a given plane in a loose granular mass is equal to the normal pressure exerted between the parts of the mass on either side of the plane, multiplied by the specific constant. The formula (1) when multiplied by the factor 'K ' is known as Rankine's earth pressure coefficient. Pl = Kwh Where, K = Rankine's coefficient Φ= angle of internal friction of the grain = natural angle of repose The internal angle of friction of the grain was assumed by theoreticians and engineers, as equal to the natural angle of repose. Therefore, Therefore, The whole weight of the grain was assumed to be transferred to the bottom of the beam. The Rankine theory with horizontal soil surface behind a vertical wall is shown in Figure. Consider an element of soil at depth 'h'. The vertical pressure acting on this element Pv and the horizontal pressure Pl is active pressure at failure. The Rankine theory Mohr Circle may be constructed as shown in fig. The Φ is the angle of internal friction of soil. Pv and Pl are the maximum and minimum principle stresses for which the Mohr Circle is constructed Pl = K Pv K = Rankine coefficient = Coefficient of earth pressure The above Rankine formula is used for determination of pressure induced by granular materials against retaining wall in shallow bins. Airy developed a theory for pressure induced by granular materials against retaining wall or in shallow bins. The lateral pressure exerted by grains in a shallow bin can be given by following Airy's equation Where, w = grain bulk density h = depth of grain to point under consideration µ = coefficient of friction of grain on grain = tan Φ, Φ is angle of internal friction µ' = tan Φ', Φ' is the angle of wall friction. The scientist Janssen took into account the friction between the grain and bin wall and, proposed the following formula for 'deep bins'. Where, Pl = lateral pressure R = hydraulic radius w = grain bulk density (unit weight of grain) µ = coefficient of friction of the grain on the wall = tan Φ h = depth of grain Problems of Silo Design 1. Wheat weighing 900 kg/m3 is loaded in a circular concrete silo of 3 m internal diameter and a clear height of 8 m. The angle of internal friction for wheat is 25° and that for wheat and concrete is 24°. Applying Airy theory, calculate the maximum lateral pressure at the bottom of bin section. Solution Lateral pressure against a shallow bin wall is Where, Pl = lateral pressure w = bulk density of grain = 900 kg/m3 h = depth of bin (point) under consideration = 8 m µ = coefficient of friction of grain to grain = tan Φ, Φ is the angle of internal friction = 250 = tan 250 = 0.466 µ' = coefficient of friction of grain to wall = tan Φ' , Φ' is the angle of external (wall) friction = 240 = tan 240 = 0.445 So, Pl= 2338.5 kg/m2 Practice Question: 1. A RC.C cylindrical grain storage bin has internal diameter of 5 m and is 20 m deep. It is completely filled with paddy weighing 600 kg/m3. The angle of internal friction for paddy can be taken as 350, while the angle of friction between paddy and bin wall is 30°. The ratio of horizontal and vertical pressure intensity k, is 0.4. Calculate the lateral pressure intensity at 4.0 m and 18 m depth using Janssen theory. Reference: K.M Sahay and K.K.Singh, 2015, A Text Book Unit Operations of Agricultural Processing, Vikas Publishing House, Noida. Lecture Note: 12 Structural, functional and thermal design of the Storage Room Size and location of the storage area are important - The availability of proper utilities must be considered when choosing the site. Three phase power and an adequate water supply must be present. Possible expansion must also be taken into account, including areas for parking, shipping and receiving, cold storage, and packing facilities. Room should be sized to handle the peak amounts of produce Square room construction - lower heat loss and lower construction costs, which are due to higher volume to surface area ratio. Economical roof span for framing systems is 12 to 19 m; therefore, buildings often have this range for the width and then are simply extended in length The height of the refrigerated spaces is often in the range of 6 to 8 m, and pallets may be stacked to a height of 4.5 to 5.5 m. The type and amount of insulation to be used for the storage rooms are dependent on the time of year when the storage room is to be used. Rooms designed to operate throughout the year require more insulation than rooms that are to be used only in the fall or summer. Ceilings require the most insulation, followed by walls and then floors. The minimal insulation requirements are 4.6 m2 ⋅°C /W in the ceiling, 4.2 m2 ⋅°C /W in the walls, and 2.3 m2 ⋅°C /W in the floors. Vapor barriers are needed to prevent moisture from forming within the insulated spaces in the walls and ceilings. Rooms that are to be used for CA or MA storage should be well sealed, because infiltration rates fluctuate with external atmospheric pressure changes and wind speed. Such changes make it difficult to maintain steady-state conditions when the passive methods of MA storage are chosen. Although conditions can be maintained in a CA storage (active control of air composition), high infiltration rates result in higher operating costs. The seals of controlled and MA storage should be checked for air leaks. A standard test is to pressurize the storage room to 25 mm (1 in) of water and to determine how long the pressure takes to decrease to 12 mm (1/2 in) of water. o A simple method to control the pressure range is a water trap, which may be constructed by building a trough in the wall and filling it with water. A thin wall is built from the main wall and extends down into the trough. The trough is filled with water until it extends up above the lower limit of the thin wall. When the pressure in the room changes, the water level in the inside rises or drops depending on the direction of the change. When the pressure reaches a certain degree, the water level will have dropped low enough on each side to allow air to enter or exit the room and relieve the pressure. o The system should be designed so that the water height differential is only 2.54 cm, resulting in a maximal pressure difference of approximately 250 Pa. Glycol is often used instead of water since it does not evaporate readily at storage room temperatures. o Another method for controlling the pressure limits in a room is to use spring- loaded or weight-loaded check valves. These valves are more expensive than the water traps. o For small pressure changes a breather bag may also be used. Breather bags are installed by cutting a hole in the wall of the storage room and sealing the open end of a larger bag around the perimeter of the hole. The increase or decrease of pressure in the room allows the bag to fill with or empty of gas. Essentially, it allows the effective volume of the room to increase or decrease, minimizing the change in pressure. Bags should have 0.35 to 0.4 m3 of capacity for every 100 m3 of room volume The standard free volume (SFV) is the ratio of the volume of air to the volume of commodity. The SFV in typical warehouses ranges from 1.5 to 3.0 and is a function of the stacking arrangement, room geometrical characteristics, commodity shape and density, and method of packing, either in bulk or in crates. CA rooms with a higher SFV have more stable gas composition with time but also require more intervention to modify the gas composition. For example, a storage room with an SFV of 3.0 requires approximately twice as much time to reach its equilibrium gas composition as a room with an SFV of 1.5, since twice as much O2 must be removed by the respiration of the produce or by the control system. Lecture Note: 13 Cold Storage Low temperature preservation is promising technology in extension of storage-life. Cold storage Based on their thermal incompatibility the produce is classified into Produce not sensitive to cold (0-4°C) E.g. Animal products, apple, grape, carrot and onion etc Produce moderately sensitive to cold (4-8°C) E.g. Mango, orange, potato and tomato (ripened) etc Produce sensitive to cold (>8°C) E.g. Pineapple, banana, pumpkin and lady’s finger etc Based on the purpose cold stores are classified: Bulk cold stores Multi-purpose cold stores Small cold stores Frozen food stores Mini units /walk in cold stores Controlled atmosphere stores Cold Load Calculations It is basic requirement to know the types of loads in the specific cold storage in order to find the capacity of the refrigeration system for the cold storage. It is necessary not only to cool the product to the storage temperature but also to meet the cooling load due to various heat infiltrations taking place in the cold storage. It is also necessary to reduce cold storage load in order to reduce the energy cost for the operation of refrigeration plant. The various factors contributing the total load of the cold storage are discussed below. Sensible heat load 1. Heat flow through walls, ceilings, floor, doors (structural heat gain). 2. Heat gain from infiltration of air due to door openings and movement of products through opening provided in the walls. For example, crates of milk enter in the milk cold storage through a gap provided in the wall using conveyer. This load is kept minimum by using appropriate strips of flexible plastic sheets to reduce the exchange of air. 3. Heat received by workers working in cold storage. Though, it is very small as number of persons working in the cold storage is very few. This load is very important in air conditioning system as it is for providing comfort to large number of occupants. 4. Heat load due to lighting and other motors used in the cold storage. Latent heat load 1. Latent heat load from infiltration of air. 2. Latent heat load from occupancy. 3. Latent heat generated from the stored products. Based on the above heat load, the actual amount of heat flow rate is calculated in order to find total load to decide the capacity of evaporator of the refrigeration plant. Wall Gain Load The heat flow rate by conduction through the walls ceiling & floor of the cold storage from outside to inside is called wall gain load. Air Change Load This is the amount of heat carried by the air when cold storage door is opened and part of cold air is replaced by outside warmer air. Product Load It is necessary to cool the product from initial temperature to the storage temperature. The amount of heat to be removed from the product to lower the temperature of the product from initial temperature to storage temperature is called product load. Miscellaneous Load The miscellaneous load consists of primarily of heat given off by light and electric motors present in the cold storage. Cooling load for electric appliances in terms of kJ is given by Qc = kW × 3600 kJ/h Total heat load = Sum of Wall Gain load, air change load, sensible heat, latent heat, miscellaneous load Lecture No 14: Controlled and Modified atmospheric Storage Controlled Atmosphere Storage CA storage implies precise control of the gas concentrations inside the storage room. Modification of atmospheric gas levels may reduce the respiration rate of fresh produce, as well as control the level of ethylene (C2H4) and thus retard ripening. The gas concentrations of ambient air are 78.08% N2, 20.95% O2, and 0.03% CO2. In most CA storage systems, the O2 level is decreased and/or the CO2 level is increased. Either generally causes a decrease in product respiration rate. Different types of produce respond differently to these two gases, and thus the proper atmosphere for a given commodity should be predetermined experimentally. In some cases, ideal concentrations of these gases for long-term storage of one commodity may prove harmful to another. For example, cauliflower stored in 10% CO2 at 5°C is injured after a week in storage, whereas broccoli in the very same environment remains in excellent condition. The choice of CA system to use depends primarily on the gas composition that is desired and the rate at which it is to be achieved. CA and MA are for long-term storage of apples, but the shelf life of certain other fruits (pears, sweet cherries) and vegetables (cabbage) can also be extended by these methods. In addition, there is considerable evidence that MA can extend the shelf life of meat, fish, poultry, fresh pasta, sandwiches, eggs, and bakery products. Because grains and oilseeds are more stable than high moisture foods (e.g., fruits, vegetables, meats), CA and MA are used primarily for disinfestation rather than for increasing the shelf life Principle behind controlled and modified atmosphere technologies is to reduce the rate of respiration, reduce microbial growth, and retard enzymatic spoilage by changing the gaseous environment surrounding the food product. This is achieved by reducing the concentration of oxygen (O2), which is required in respiration, or by adding an inhibitory gas such as carbon dioxide (CO2) or carbon monoxide (CO). The balance between O2 and CO2 is critical, and an optimal ratio is required for each specific product. A major difference between CA and MA storage is in the degree of control of the gaseous composition of the storage atmosphere. The CA implies a higher degree of control than MA in maintaining specific levels of O2, CO2, and other gases. Also, in MA storage the composition of the atmosphere surrounding the product is generally created and maintained by the interaction of the commodity’s respiration with the permeation of respiratory gases through the packaging material. Modified atmosphere conditions can also be established and adjusted by pulling a slight vacuum and replacing the package atmosphere with a desirable gas mixture, which can be further, adjusted through the use of O2, CO2, or ethene (C2H4) absorbers. In CA storage facilities, both temperature and gas composition of the storage atmosphere are regulated or controlled. The gas concentration ranges encountered in CA storages are 1 to 10% O2, 0 to 30% CO2, and the balance is nitrogen (N2). Air consists of approximately 78% N2, 21% O2, 0.03% CO2, and traces of several other gases that have no physiological significance. Practical quality advantages include: 1. Reduction in chlorophyll breakdown, with resulting higher colour stability. 2. Reduction in enzymatic browning in cut produce, whenever low levels of O2 are used. 3. Improvement in texture caused by the action of CO2 on enzymes acting on cellular membranes. 4. Reduction in some physiological disorders induced by C2H4, such as scald of apples and pears and chilling injury of citrus fruits, avocado, chili pepper, and okra. 5. Reduction in microbial activity especially molds. The marketing and distribution advantages of CA or MA technologies include: 1. Reduction in fresh food spoilage and quality loss through the distribution at the retail level. 2. Expanded radius of distribution systems and market area. 3. Improved branding options and product differentiation. 4. Potential for increased profitability in all fresh or chilled food operations. Other concerns associated with CA or MA technology include: 1. Development of off-flavors due to accumulation of ethanol, acetaldehyde, and other volatiles. 2. Increased softening in products such as cucumbers, cauliflower, celery, and onion. 3. Development of physiological disorders, such as brown stain on lettuce, internal browning and surface pitting of some fruits, and blackheart of potatoes induced by inappropriate modified atmospheres. 4. Increased susceptibility of some products (celeriac, carrot, pepper, Chinese cabbage, and citrus fruits) to post-harvest pathogens. The three main control systems that may be used to obtain the desired gas concentrations: 1. O2 control systems (a) external burners The combustion of propane or natural gas to remove O2 from air that enters the storage room. Open flame or catalytic burners may be used. Precautions: (a) Requires a CO2 scrubber to prevent high accumulations of the gas. (Combustion process produces a mixture of CO2 and water vapor) (b) The air should be cooled after combustion and before it is injected into the room. (c) Risk of extinguishing the flame by the O2-depleted recirculating airstream. liquid or gaseous nitrogen (N2) (b) gas separator systems i. pressure swing absorption (PSA) ii. hollow fiber membrane separators (HFMSs) iii. high-temperature ammonia cracking (HTAC). (c) hypobaric storage (d) liquid N2 is injected into the room through spray headers that atomize the N2 into a fine mist. 2. CO2 control systems scrubbing systems for the removal of excess CO2. These are based on (a) caustic soda (b) hydrated lime (c) water (d) activated charcoal (e) molecular sieves. 3. C2H4 control systems (a) the heated catalyst scrubber (b) C2H4absorbing beads (c) Ozone. 4. Relative humidity Relative humidity is another important factor for the long-term storage of perishable commodities. Low relative humidity allows excessive moisture loss from the produce, resulting in a loss of quality. The recommended humidity level for the storage of fresh fruits and vegetables is commodity specific; levels are generally in the range of85% to95%. Wilting and shriveling are likely to occur in most vegetable crops when stored at low humidity. However, relative humidity close to 100% can be ideal for the growth of microorganisms and can cause surface cracking in some produce. Low relative humidity is generally difficult to obtain in storage rooms. It is best to use large evaporator coils for the refrigeration system so that the coils operate at 3°C lower than the surrounding air temperature and thus reduce condensation The smaller the temperature difference between the evaporator coils and the air, the less the moisture loss. Providing good insulation and minimizing air leaks are steps that can be taken to maintain high relative humidity. Systems that have a lot of condensation on the evaporator coils need to be defrosted often, since the build-up of frost or ice on the coils results in decreased refrigeration capacity. Mechanical humidifiers, spray nozzles, or steam systems may also be used to increase the humidity in the storage room. Pressure-atomized or heat-vaporized water can be added to the room to add moisture. A rate of 4 L water/h per ton of refrigerant should be adequate to maintain a relative humidity of 95%. These systems are an added expense and require additional maintenance. 5. Temperature Molecular mobility is depressed and consequently chemical reactions and biological processes are slowed down at low temperature. Low temperature is produced by refrigeration system using refrigerants (either by vapour absorption or vapour compression), Peltier effect, thermoelectric cooling, vacuum cooling, hypobaric cooling, ice, etc. The temperature is measured continuously and monitoring throughout the store unit by real time and temperature indicators. Read More from: 1. Amalendu Chakraverty, Arun S. Mujumdar G. S. Vijaya Raghavan and Hosahalli S., (2003) Handbook of Postharvest Technology Cereals, Fruits, Vegetables, Tea, and SpicesRamaswamy., Marcel Dekker, Inc.