ENZYMES.pdf
Document Details
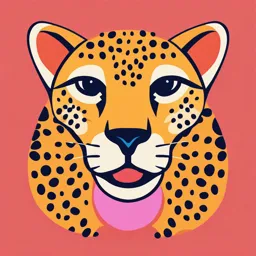
Uploaded by RefreshedJudgment
Tags
Full Transcript
Nafith Abu Tarboush DDS, MSc, PhD [email protected] www.facebook.com/natarboush The function of nearly all proteins depends on their ability to bind other molecules (ligands) Two properties of a protein characterize its interaction with ligands: Affinity: the strength of binding between...
Nafith Abu Tarboush DDS, MSc, PhD [email protected] www.facebook.com/natarboush The function of nearly all proteins depends on their ability to bind other molecules (ligands) Two properties of a protein characterize its interaction with ligands: Affinity: the strength of binding between a protein and other molecule Specificity: the ability of a protein to bind one molecule in preference to other molecules In the human body, almost every metabolic process involve the use of enzymes crushed leaves are exposed to the oxygen in air, a polyphenoloxidase breaks up polyphenols into tannins which impart the darker color and characteristic flavors Sucrose (table sugar), yeast enzyme breaks sucrose into its two smaller sugar What are enzymes? (specialized proteins, small amounts, acceleration, no change). Ribozymes are the exception Enzymes are the most efficient catalysts known Usually in the range of 106 to 1014 Non-enzymatic catalysts (102 to 104) The actions of enzymes are fine-tuned by regulatory processes Examples: catalase (108) & carbonic anhydrase (107) In enzymatic reactions, reactants are known as substrates We can simply express an enzymatic reaction using this formula E + S ES EP E + P Or E + S ES E + P where E is the free enzyme; S is the free substrate, ES is the enzyme-substrate complex; P is the product of the reaction; and EP is the enzyme-product complex before the product is released A specific three-dimensional shape which includes a region where the biochemical reaction takes place Contains a specialized amino acid sequence that facilitates the reaction Within the active site are two sub-sites, the binding site and the catalytic site, The binding & catalytic site may be the same Binding site: binds substrate through ionic, H-bonding or other electrostatic forces, or hydrophobic interactions Catalytic site: contains the catalytic groups Active sites; structures that look like canals, clefts or crevices Water is usually excluded after binding unless it participates in the reaction Substrates are bound to enzymes by multiple weak attractions (electrostatic, hydrogen, van der Waals, & hydrophobic) Binding occurs at least at three points (chirality) Forms by groups from different parts of the amino acid sequence usually forming a domain made of multiple secondary structures Takes up a relatively small part of the total volume The “extra” amino acids help create the three-dimensional active site & in many enzymes, may create regulatory sites Binding leads to formation of transition-state Usually, substrate binds by non-covalent interactions to the active site The catalyzed reaction takes place at the active site, usually in several steps Two models, lock-and-key vs. induced-fit model Glucose and hexokinase, phosphorylation Improving the binding site for ATP & excluding water (might interfere with the reaction) ΔG = ΔH - TΔS Spontaneous vs. non-spontaneous, favorable vs. non-favorable, exergonic vs. endergonic, exothermic vs. endothermic, switch of signs ΔG, ΔG° Biochemical pathways; storage (endergonic) & release (exergonic) Kinetics (rate) vs. Thermodynamics (favorability) Enzymes speed up reactions, but have no relation to equilibrium or favorability What is an activation energy (ΔG°‡) concept? Specificity varies (stereoisomers), however, there is none nonspecific Spontaneous vs. rate! What is the transition state? Transition-state complex binds more tightly to the enzyme compared to substrate Substrates of enzymatic reactions often undergo several transformations when associated with the enzyme and each form has its own free energy value Which one is the activation energy? Activation energy & final ΔG calculation Proximity effect: Bring substrate(s) and catalytic sites together Orientation effect: Hold substrate(s) at the exact distance and in the exact orientation necessary for reaction Catalytic effect: Provide acidic, basic, or other types of groups required for catalysis Energy effect: Lower the energy barrier by inducing strain in bonds in the substrate molecule Enzyme-substrate interactions orient reactive groups and bring them into proximity with one another favoring their participation in catalysis Such arrangements have been termed near-attack conformations (NACs) NACs are precursors to reaction transition states In this form of catalysis, the induced structural rearrangements produce strained substrate bonds reducing the activation energy. Example: lysozyme The substrate, on binding, is distorted from the typical 'chair' hexose ring into the 'sofa' conformation, which is similar in shape to the transition state The R groups act as donors or acceptors of protons Histidine is an excellent proton donor/acceptor at physiological pH Example: serine proteases A covalent intermediate forms between the enzyme or coenzyme and the substrate Examples of this mechanism is proteolysis by serine proteases, which include digestive enzymes (trypsin, chymotrypsin, and elastase) In general, enzymes end with the suffix (-ase) Most enzymes are named for their substrates and for the type of reactions they catalyze, with the suffix “ase” added For example; ATPase is an enzyme that breaks down ATP, whereas ATP synthase is an enzyme that synthesizes ATP Some enzymes have common names that provide little information about the reactions that they catalyze Examples include the proteolytic enzyme trypsin A numerical classification scheme for enzymes, based on the chemical reactions they catalyze Strictly speaking, EC numbers do not specify enzymes, but enzymecatalyzed reactions Numbering format: EC followed by four numbers separated by periods Major class (1-7), Minor class, subclass, further sub-classification For example: tripeptide aminopeptidases "EC 3.4.11.4" EC 3: hydrolases EC 3.4: hydrolases that act on peptide bonds EC 3.4.11: hydrolases that cleave off the amino-terminal of the amino acid polypeptide EC 3.4.11.4: cleave off the amino-terminal end from a tripeptide Simple vs. complex (conjugated) Holoenzyme vs. apoenzyme Oxidoreductases: addition or removal of O, O2, H. Require coenzymes (heme) Transferases: transfer of a group from one molecule to another Hydrolases: addition of water (carbs. & proteins) Lyases: addition of a molecule (H2O, CO2, NH3) to a double bond or reverse Isomerases: one substrate and one product Ligases: usually not favorable, so they require a simultaneous hydrolysis reaction These enzymes catalyze oxidation & reduction reactions involving the transfer of hydrogen atoms, electrons or oxygen This group can be further divided into 4 main classes: Dehydrogenases Oxidases Peroxidases Oxygenases Dehydrogenases catalyze hydrogen transfer from the substrate to a molecule known as nicotinamide adenine dinucleotide (NAD+) Lactate dehydrogenase Lactate + NAD+ Pyruvate + NADH + H+ Alcohol dehydrogenase Oxidases catalyze hydrogen transfer from the substrate to molecular oxygen producing hydrogen peroxide as a by-product Glucose oxidase -D-glucose + O2 gluconolactone + H2O2 Peroxidases catalyze oxidation of a substrate by hydrogen peroxide Oxidation of two molecules of glutathione (GSH) in the presence of hydrogen peroxide: 2 GSH + H2O2 G-S-S-G + 2 H2O Oxygenases catalyze substrate oxidation by molecular O2 The reduced product of the reaction in this case is water and not hydrogen peroxide There are two types of oxygenases: Monooxygenases; transfer one oxygen atom to the substrate, and reduce the other oxygen atom to water Dioxygenases, incorporate both atoms of molecular oxygen (O2) into the product(s) of the reaction These enzymes transfer a functional group (C, N, P or S) from one substrate to an acceptor molecule Phosphofructokinase; catalyzes transfer of phosphate from ATP to fructose-6-phosphate: Fructose 6-P + ATP F 1,6 bisphosphate + ADP A transaminase transfers an amino functional group from one amino acid to a keto acid, converting the amino acid to a keto acid and the keto acid to an amino acid This allows for the interconversion of certain amino acids These enzymes catalyze cleavage reactions while using water across the bond being broken Peptidases, esterases, lipases, glycosidases, phosphatases are all examples of hydrolases named depending on the type of bond cleaved These enzymes catalyze proteolysis, the hydrolysis of a peptide bond within proteins Proteolytic enzymes differ in their degree of substrate specificity Trypsin, is quite specific; catalyzes the splitting of peptide bonds only on the carboxyl side of lysine and arginine Thrombin, catalyzes the hydrolysis of Arg-Gly bonds in particular peptide sequences only Catalyze the addition or removal of functional groups from their substrates with the associated formation or removal of double bonds between C-C, C-O and C-N Aldolase; breaks down fructose-1,6-bisphosphate into dihydroxyacetone phosphate and glyceraldehydes-3-phosphate F 1,6 bisphosphate DHAP + GAP Enolase; interconverts phosphoenolpyruvate and 2phosphoglycerate by formation and removal of double bonds Catalyze intramolecular rearrangements Glucose-6-phosphate isomerase; isomerizes glucose6-phosphate to fructose-6phosphate Phosphoglycerate mutase; transfers a phosphate group from carbon number 3 to carbon number 2 of phosphorylated glycerate (BPG intermediate) 3-P glycerate 2 P glycerate Ligases join C-C, C-O, C-N, C-S and C-halogen bonds The reaction is usually accompanied by the consumption of a high energy compound such as ATP Pyruvate carboxylase Pyruvate + HCO3- + ATP Oxaloacetate + ADP + Pi A. Not all enzymes rely on their active site for catalysis (chymotrypsin vs. conjugated enzymes) Cojugated: coenzymes, metal ions, & metallocoenzymes Functional Groups on Amino Acid Side Chains: Almost all polar amino acids (nucleophilic catalysis) Ser, Cys, Lys, & His can participate in covalent catalysis Histidine: pKa, physiological pH & acid–base catalysis B. Coenzymes in Catalysis Usually (but not always) synthesized from vitamins Each coenzyme is specific for a type of reaction They are either: * Activation-transfer coenzymes * Oxidation–reduction coenzymes Metal Organic Apoenzyme vs. holoenzyme Organic (coenzymes) Prosthetic groups (tightly bound) vs. co-substrates (loosely bound) Tryptophantryptophyl quinone Organometallic Cross-linked amino acid TPQ Stable free radicals LTQ CTQ Usually participate directly in catalysis by forming a covalent bond Characteristics: Two groups in the coenzyme: ▪ Forms a covalent bond (functional group) ▪ Binds tightly to the enzyme (binding group) Dependence on the enzyme for additional specificity of substrate & additional catalytic power Thiamine pyrophosphate Source: thiamine (B1) Decarboxylation reactions Pyrophosphate: Provides negatively charged oxygen atoms Chelate Mg2+ (tight binding) Functional group (reactive carbon atom) Reactive thiamine carbon forms a covalent bond with a substrate keto group while cleaving the adjacent carbon– carbon bond Thiamin (vitamin B1) is rapidly converted to its active form, thiamin pyrophosphate, TPP, in the brain & liver Required by pyruvate dehydrogenase & α-ketoglutarate dehydrogenase Source: pantothenate (B5) Binding group: adenosine 3’,5’-bisphosphate (tight & reversible) Functional group: sulfhydryl group (nucleophile) Attacks carbonyl groups & forms acyl thioesters (the “A”) How it is different from usual? (regeneration & acyl-CoA derivative) Like some others (NAD+), why do they call them coenzymes? ▪ Common to so many reactions ▪ The original form is regenerated by subsequent reactions ▪ Synthesized from vitamins ▪ The amount in the cell is nearly constant Biotin is required for carboxylation reactions (covalently bound to Lys) Source: food & intestinal bacteria Deficiencies are generally seen Long antibiotic therapies Excessive consumption of raw eggs (egg white protein, avidin, high affinity for biotin) Pyruvate carboxylase Acetyl CoA carboxylase (fatty acid synthesis) Synthesis: Pyridoxine (B6) Functions in the metabolism of amino acids (transaminases) Reversible reactions Mechanism: Reactive aldehyde forms a covalent bond with the amino groups Ring nitrogen withdraws electrons from bound amino acid (cleavage of bond) A large number of coenzymes Do not form covalent bonds with the substrate Most common: NAD+ (niacin, B3) & FAD (riboflavin, B2) Others: work with metals to transfer single electrons to O2 (Vitamins E & C) Again: Dependence on the enzyme for additional specificity of substrate & additional catalytic power Functional group (C opposite to N) Accepts a hydride ion The H+ from substrate dissociates, & a keto group (CO) is formed (ADP) portion of the molecule binds tightly The role of enzymes’ Histidine Source: Riboflavin (B2) FMNH2 and FADH2 Flavoproteins FAD and FMN are prosthetic groups (tightly bound) Succinate dehydrogenase Pyruvate dehydrogenase complex Metals can be tightly bound (metalloenzymes) or loosely bound (metal-activated enzymes) Acting as electrophiles Metal-activated enzymes; the metal either required or enhances activity (Mg2+, Mn2+, Ca2+, & K+) Phosphofructokinase & TPP; (Mg2+) is required to coordinate the phosphate groups on the ATP for a successful reaction (chelation) Metal Enzyme Zn2+ Carbonic anhydrase Zn2+ Carboxypeptidase Mg2+ Hexokinase Se Glutathione peroxidase Mn2+ Superoxide dismutase Fructose-6-phosphate + ATP → fructose-1,6-bisphosphate + ADP Alcohol dehydrogenase (ADH) Activated serine (pulls a proton off –OH) Oxyanion is stabilized by zinc Transfer of a hydride ion to NAD+ Zinc in ADH as His in lactate dehydrogenase Metal ions are usually incorporated during synthesis & removal of the metal causes denaturation These metal ions may contribute either to the structure or the catalytic mechanism Liver alcohol dehydrogenase (dimer); 2 Zn+2 in each monomer; one for structural maintenance (joins the two subunits), the other is catalytic Carbonic anhydrase; A zinc atom is essentially always bound to four or more groups Biochemical Kinetics: the science that studies rates of chemical reactions An example is the reaction (A P), The velocity, v, or rate, of the reaction A P is the amount of P formed or the amount of A consumed per unit time, t. That is, or The rate is a term of change over time The rate will be proportional to the conc. of the reactants It is the mathematical relationship between reaction rate and concentration of reactant(s) For the reaction (A + B P), the rate law is From this expression, the rate is proportional to the concentration of A, and k is the rate constant A multistep reaction can go no faster than the slowest step v = k(A)n1(B)n2(C)n3 k is the rate constant: the higher the activation energy (energy barrier), the smaller the value of k (n1+n2+n3) is the overall order of the reaction Dimensions of k Overall order V= Dimentions of k Zero k (conc.)(time)-1 First k(A) (time)-1 Enzymatic reactions may either have a simple behavior or complex (allosteric) behavior Simple behavior of enzymes: as the concentration of the substrate rises, the velocity rises until it reaches a limit Thus; enzyme-catalyzed reactions have hyperbolic (saturation) plots The maximal rate, Vmax, is achieved when the catalytic sites on the enzyme are saturated with substrate Vmax reveals the turnover number of an enzyme The number of substrate molecules converted into product by an enzyme molecule in a unit of time when the enzyme is fully saturated with substrate At Vmax, the reaction is in zero-order rate since the substrate has no influence on the rate of the reaction For a reaction: Km, called the Michaelis constant is In other words, Km is related to the rate of dissociation of substrate from the enzyme to the enzyme-substrate complex Km describes the affinity of enzyme for the substrate A quantitative description of the relationship between the rate of an enzyme catalyzed reaction (V0) & substrate concentration [S] The rate constant (Km) and maximal velocity (Vmax) When [S] << Km Linear relation V = X [S] The substrate concentration at which V0 is half maximal is Km When [S] >> Km Independent relation The lower the Km of an enzyme towards its substrate, the higher the affinity When more than one substrate is involved? Each will have a unique Km & Vmax Km values have a wide range. Mostly between (10-1 & 10-7 M) KD: dissociation constant, The actual measure of the affinity KD = (k-1/k1) When you increase the enzyme concentration, what will happen to Vmax & Km? For the enzymatic reaction k1 k2 k-1 The maximal rate, Vmax, is equal to the product of k2, also known as kcat, and the total concentration of enzyme Vmax = k2 [E]T kcat, the turnover number, is the concentration (or moles) of substrate molecules converted into product per unit time per concentration (or moles) of enzyme, or when fully saturated kcat = Vmax/ [E]T In other words, the maximal rate, Vmax, reveals the turnover number of an enzyme if the total concentration of active sites [E]T is known a 10-6 M solution of carbonic anhydrase catalyzes the formation of 0.6 M H2CO3 per second when it is fully saturated with substrate Hence, kcat is 6 × 105 s-1 3.6 X 107 min-1 Each catalyzed reaction takes place in a time equal to 1/k2, which is 1.7 μs for carbonic anhydrase The turnover numbers of most enzymes with their physiological substrates fall in the range from 1 to 104 per second Specificity constant (kcat/KM): determines the relative rate of the reaction at low [S] kcat/KM (M-1 min-1) is indicative of: Enzyme’s substrate specificity: the higher the ratio, the higher the specificity Enzyme’s catalytic efficiency: the higher the ratio, the more efficient the enzyme kcat values vary over a wide range KM values also vary over a wide range Kcat/KM, the range is only 4 Reaction rate; measures the concentration of substrate consumed (or product produced) per unit time (mol/{L.s} or M/s) Enzyme activity; measures the number of moles of substrate consumed (or product produced) per unit time (mol/s) Enzyme activity = rate of reaction × reaction volume Specific activity; measures moles of substrate converted per unit time per unit mass of enzyme (mol/{s.g}) Specific activity = enzyme activity / actual mass of enzyme This is useful in determining enzyme purity after purification Turnover number; measures moles of substrate converted per unit time per moles of enzyme (min-1 or s-1) Turnover number = specific activity × molecular weight of enzyme A solution contains initially 25X10-4 mol L-1 of peptide substrate and 1.5 μg chymotrypsin in 2.5 ml volume. After 10 minutes, 18.6X10-4 mol L-1 of peptide substrate remain. Molar mass of chymotrypsin is 25,000 g mol-1. How much is the rate of the reaction? ▪ (conc./time) How much is the enzyme activity? ▪ (mol./time) How much is the specific activity? ▪ (enz. Act. / enz. Mass) How much is the turn over number? ▪ (sp. Act. X enz. molar mass) Determining the Km from hyperbolic plots is not accurate since a large amount of substrate is required in order to reach Vmax This prevents the calculation of both Vmax & Km Lineweaver-Burk plot: A plot of 1/v0 versus 1/[S] (doublereciprocal plot), yields a straight line with an y-intercept of 1/Vmax and a slope of KM/Vmax The intercept on the x-axis is -1/KM ب+ص=أس A biochemist obtains the following set of data for an enzyme that is known to follow Michaelis-Menten kinetics. Approximately, Vmax of this enzyme is … & Km is …? A. 5000 & 699 B. 699 & 5000 C. 621 & 50 D. 94 & 1 E. 700 & 8 You are working on the enzyme “Medicine” which has a molecular weight of 50,000 g/mol. You have used 10 μg of the enzyme in an experiment and the results show that the enzyme converts 9.6 μmol per min at 25˚C. the turn-over number (kcat) for the enzyme is: A. 9.6 s-1 B. 48 s-1 C. 800 s-1 D. 960 s-1 E. 1920 s-1 Isozymes Inhibition Conformation Amount None-specifically What are isozymes? Same substrate & product, different gene, different localization, different parameters (Km, Vmax, kcat) Hexokinase found in RBCs & in liver Catalyzes the first step in glucose metabolism Hexokinase I (RBCs): KM (glucose) ≈ 0.1 mM Hexokinase I V (glucokinase, liver, pancreas) ≈ 10 mM RBCs: when blood glucose falls below its normal fasting level (≈ 5 mM), RBCs could still phosphorylate glucose at rates near Vmax Liver: rate of phosphorylation increases above fasting levels (after a highcarbohydrate meal) High KM of hepatic glucokinase promotes storage of glucose Pancreas: works as a sensor Oxidation of acetaldehyde to acetate. Four tetrameric isozymes (I-IV) ALDH I (low Km; mitochondrial) and ALDH II (higher Km; cytosolic) ~50% of Japanese & Chinese are unable to produce ALDH I (not observed in Caucasian & Negroid populations) Flushing response Tachycardia Mechanism-based inhibitors mimic or participate in an intermediate step of the catalytic reaction The term includes: A. Covalent inhibitors B. Transition state analogs C. Heavy metals The kinetic effect of irreversible inhibitors is to decrease the concentration of active enzyme Covalent or extremely tight bonds with active site amino acids Amino acids are targeted by drugs & toxins The lethal compound [DFP] is an organophosphorus compound that served as a prototype for: The nerve gas sarin The insecticides malathion & parathion DFP also inhibits other enzymes that use serine (ex. serine proteases), but the inhibition is not as lethal Aspirin (acetylsalicylic acid): covalent acetylation of an active site serine in the enzyme prostaglandin endoperoxide synthase (cyclooxygenase) Aspirin resembles a portion of the prostaglandin precursor that is a physiologic substrate for the enzyme Transition-state analogs: extremely potent inhibitors (bind more tightly) Drugs cannot be designed that precisely mimic the transition state! (highly unstable structure) Substrate analogs: bind more tightly than substrates Known as suicide inhibitors Synthetic inhibitor Anticancerous Analog of tetrahydrofolate Binds to enzyme a 1000-fold more tightly Inhibits nucleotide base synthesis A transition-state analog to glycopeptidyl transferase or transpeptidase Required by bacteria for synthesis of the cell wall The reaction is favored by the strong resemblance between the peptide bond in the β-lactam ring of penicillin & the transition-state complex of the natural transpeptidation reaction Inhibitors that undergo partial reaction to form irreversible inhibitors in the active site are sometimes termed suicide inhibitors A drug used to treat gout Decreases urate production by inhibiting xanthine oxidase The enzyme commits suicide by converting the drug to a transitionstate analog The enzyme contains a molybdenum–sulfide (Mo-S) complex that binds the substrates and transfers the electrons required for the oxidation reactions Xanthine oxidase oxidizes the drug allopurinol to oxypurinol, a compound that binds very tightly to a molybdenum–sulfide complex in the active site Tight binding of a metal to a functional group in an enzyme Mercury (Hg), lead (Pb), aluminum (Al), or iron (Fe) Relatively nonspecific for the enzymes they inhibit, particularly if the metal is associated with high-dose toxicity Mercury: binds to so many enzymes, often at reactive sulfhydryl groups in the active site It has been difficult to determine which of the inhibited enzymes is responsible for mercury toxicity Lead provides an example of a metal that inhibits through replacing the normal functional metal in an enzyme, such as calcium, iron, or zinc Its developmental & neurologic toxicity may be caused by its ability to replace Ca+2 in several regulatory proteins that are important in the central nervous system and other tissues Characterized by a rapid dissociation of the enzyme-inhibitor complex Usually these inhibitors bind through non-covalent interactions & inhibitor maintains a reversible equilibrium with the enzyme Reversible inhibitors can be divided into two classes: competitive & noncompetitive The double-reciprocal plots are highly useful for distinguishing among these inhibitors The inhibitor competes with substrate Increasing [S] can overcome the inhibition (Vmax) Does KM change? Significance (ex. Hexokinase) The inhibitor binds at a site other than the active site The complex does not proceed to form product or has a lower efficiency Vmax vs. KM Can we reach Vmax? These regulatory mechanisms include A. Allosteric activation and inhibition; B. Phosphorylation or other covalent modification; C. Protein-protein interactions between regulatory & catalytic subunits or between two proteins; D. Proteolytic cleavage These types of regulation can rapidly change an enzyme from an inactive form to a fully active conformation Chymotrypsin: Specificity for aromatic residues mainly. Also, hydrolysis of ester bonds Aspartate transcarbamoylase (ATCase): synthesis of CTP & UTP for RNA and DNA synthesis What are allosteric enzymes? A multisubunit enzyme with catalytic subunit(s) and regulatory subunit(s) Binding triggers a conformational change in the active site The Michaelis-Menten model can’t explain the kinetic properties The effect of the modulators (allosteric modifiers) Homotropic vs. heterotropic The substrate concentration at half of the Vmax is called (K0.5) Allosteric inhibitors have a much stronger effect on enzyme velocity activator inhibitor The effect of modifiers on Vmax & K0.5 Two conformations: more active (R) & less active or inactive (T), The equilibrium ratio (T/R) is called L and assumed to be high As L (T/R) increases, the shape becomes more sigmoidal Either substrate or activator must be increased to overcome the effects of the allosteric inhibitor Conformational change ATCase and Hb are allosteric proteins (cooperative behavior) Catalytic can be separated from regulatory (hyperbolic) Cooperativity in relation to substrate CTP is an inhibitor of ATCase (feedback inhibition), ATP is an activator Aspartate Why is it effective? Adds two negative charges: new electrostatic interactions and accordingly conformation Can form three or more hydrogen bonds: specific interactions with hydrogen-bond donors Can take place in less than a second or over a span of hours Often causes highly amplified effects Rapid and transient regulation of enzyme activity - REVERSIBLE Phosphorylation: (Ser, Thr, & Tyr) Mostly, ATP is the donor Kinases vs. phosphatases Phosphorylation does not lead always to activation of enzymes Glycogen phosphorylasereaction (two forms; a & b). Ser is away from the active site Both phosphorylase b and phosphorylase a exist as equilibria between an active R state and a less-active T state Phosphorylase b is usually inactive because the equilibrium favors the T state Phosphorylase a is usually active because the equilibrium favors the R state The transition of phosphorylase b between the T and the R state is controlled by the energy charge of the muscle cell. Protein kinase A (PKA): refers to a family of enzymes whose activity is dependent on cellular levels of cyclic AMP (cAMP) cAMP: referred to as a hormonal 2nd messenger Either dedicated or not Has several functions in the cell, including regulation of glycogen, sugar, & lipid metabolism Adrenaline (epinephrine) → ↑cAMP → activates protein kinase A → phosphorylates & activates glycogen phosphorylase kinase → phosphorylates & activates glycogen phosphorylase Phosphorylation cascade Adenylylation (addition of adenylyl group). AMP (from ATP) is transferred to a Tyr hydroxyl by a phosphodiester linkage. The addition of bulky AMP inhibits certain cytosolic enzymes. Uridylylation ( addition of uridylyl group). ADP-ribosylation: inactivates key cellular enzymes Methylation: masks a negative charge & add hydrophobicity on carboxylate side chains Acetylation: masks positive charges when added to lysine residues G protein: a family of trans-membrane proteins causing changes inside the cell. They communicate signals from hormones, neurotransmitters, and other signaling factors When they bind guanosine triphosphate (GTP), they are 'on', and, when they bind guanosine diphosphate (GDP), they are 'off‘ α-Subunit can be stimulatory or inhibitory Same as Gα Hydrolysis vs. exchange Activation or inhibition Example: RAS Irreversible cut usually at the Nterminus Trypsin, chymotrypsin, pepsin (trypsinogen, pepsinogen chymotrypsinogen) Chymotrypsinogen: single polypeptide chain (245 residues), 5 (S—S) bonds Blood clotting The soluble protein fibrinogen is converted to the insoluble protein fibrin Regulated Enzyme Synthesis Regulated by increasing or decreasing the rate of gene transcription (induction & repression) Usually slow in humans (hours to days) Sometimes through stabilization of the messenger RNA A. B. Regulated Protein Degradation Can be degraded with a characteristic half-life within lysosomes During fasting or infective stress: gluconeogenesis increase & synthesis of antibodies (protein degradation increases) Increased synthesis of ubiquitin Increase in T° increases the rate until reaches a max (≈ 50°): the optimal temperature of each enzyme is its’ denaturation Autoclave steam heating Hypothermia, metabolic reactions, cardiac surgery Usually a well defined optimum point Most enzymes have their max. activity between (5-9) Extremes of pH denatures protein pH can alter binding of substrate to enzyme (KM) by altering the protonation state of the substrate, or altering the conformation of the enzyme The effect of pH is enzyme-dependent Thermophiles (heat lovers) Taq polymerase and PCR Psychrophiles (cold lovers) Biobleaching of paper pulp using heat-stable xylanases lipases and proteases An antibody that is produced against a transition-state analog (active) An abzyme is created in animals RNA molecules Examples: telomerase & RNase P Catalyze splicing reactions and are involved in protein synthesis The catalytic efficiency of catalytic RNAs is less than that of protein enzymes, but can greatly be enhanced by the presence of protein subunits 1. COUNTERREGULATION OF OPPOSING PATHWAYS Synthesis vs. degradation (a different regulatory enzyme) 2. TISSUE ISOZYMES OF REGULATORY PROTEINS 3. REGULATION AT THE RATE-LIMITING STEP Pathways are principally regulated at their rate-limiting step The slowest step & is usually not readily reversible Changes in this step can influence flux through the rest of the pathway Usually the first committed step in a pathway Requirement for high amount of energy High KM values of enzyme towards its substrate 4. The committed step A committed step in a metabolic pathway is the first irreversible reaction that is unique to a pathway and that, once occurs, leads to the formation of the final substrate with no point of return Committed steps are exergonic reaction For example, the committed step for making product E is (B → C), not (A → B) A C D E X Y Z B 5. FEEDBACK REGULATION This type of regulation is much slower to respond to changing conditions than allosteric regulation Negative feedback regulation (feedback inhibition) Positive feedback regulation Feed-forward regulation Disposal of toxic compounds 6. Enzyme compartmentalization Both enzymes and their substrates are present in relatively small amount in a cell A mechanism by which rate of reactions become faster is their compartmentalization; reducing area of diffusion In this way, enzymes are sequestered inside compartments where access to their substrates is limited Lysosomes; proteins get transported to lysozymes Mitochondria; energy metabolic pathways Metabolism of fatty acids; synthesis (cytosol) vs. degradation (mitochondria) 7. Enzyme complexing (A multienzyme complex) Complexing various enzymes that share one process Product of enzyme A pass directly to enzyme B Pyruvate dehydrogenase (mitochondria) 3 enzymes: decarboxylation, oxidation, & transfer of the resultant acyl group to CoA Concept Examples: ALT, AST, LDH, CK (CPK) Liver disease: ALT & AST ALT is the most specific Ratio can also be diagnostic (ALT/AST) In liver disease or damage (not of viral origin): ▪ ratio is less than 1 With viral hepatitis: ▪ ratio will be greater than 1 LDH-1/LDH-2 ratio is diagnostic for myocardial infarction (heart attacks) Normally, this ratio is less than 1 Following an acute myocardial infarct, the LDH ratio will be more than 1 Heart, skeletal muscles, & brain Like LDH, there are tissue-specific isozymes of CPK: CPK3 (CPK-MM): the predominant isozyme in muscle CPK2 (CPK-MB): accounts for ≈35% of CPK activity in cardiac muscle, but less than 5% in skeletal muscle CPK1 (CPK-BB) is the characteristic isozyme in brain and is in significant amounts in smooth muscle Most of released CPK after MI is CPK-MB Increased ratio of CPK-MB/total CPK may diagnose acute infarction, but an increase of total CPK in itself may not The CPK-MB is also useful for diagnosis of reinfarction because it begins to fall after a day and disappears in 1 to 3 days, so subsequent elevations are indicative of another event 1. MI 2. MI (hrs post) 3. LDH proteins 4. Liver disease 5. MI (2d post) 6. MI (1d post) 7. Liver + HF 8. Normal Sample #3 represents results for a control. Sample #8 results are from a normal specimen. Sample# 1 MI patient. The specimen was collected at a time when the activity of both LDH and CK were elevated. Note the LDH flip and the high relative activity of the MB isoenzyme. Sample# 2 MI patient who experienced chest pain only several hours previously. Total CK is significantly elevated with a high relative MB isoenzyme activity. Sample# 6 MI patient (the 1st day post MI); CK activity is definitely elevated with a high relative MB isoenzyme activity and the LDH flip is evident. Sample# 5 MI patient (2 days post MI) so that CK has almost returned to normal activity and the LDH flip is definite. Sample# 7 MI patient with complications of heart failure and passive liver congestion or the patient was involved in an accident as a consequence of the MI, and suffered a crushing muscle injury. Sample# 4 a patient with liver disease. Although the LDH isoenzyme pattern is indistinguishable from muscle disease or injury, the absence of at least a trace of CKMB isoenzyme is inconsistent with the muscle CPK isoenzyme distribution as is the apparently normal total activity. Like all cardiac markers, troponins have a unique diagnostic window Troponin levels rise within four to six hours after the beginning of chest pain or heart damage, and stay elevated for at least one week. This long elevation allows detection of a myocardial infarction that occurred days earlier, but prevents detection of a second infarction if it occurred only days after the first