Enzymes & Metabolism - in class notes.pdf
Document Details
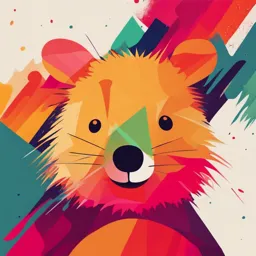
Uploaded by DeliciousCadmium
2024
Tags
Full Transcript
Canadian School of Milan Fall Semester 2024 Teacher: Mr. Valente Email: [email protected] 12TH GRADE BIOLOGY ___ Class Notes #1 Metabolism is the complex network of interdependent and interacting enzyme catalysed chemical reactions occurring in living organisms. Enzymes have a specifi...
Canadian School of Milan Fall Semester 2024 Teacher: Mr. Valente Email: [email protected] 12TH GRADE BIOLOGY ___ Class Notes #1 Metabolism is the complex network of interdependent and interacting enzyme catalysed chemical reactions occurring in living organisms. Enzymes have a specificity and only catalayse one reaction. So many different enzymes are required by living organisms for their metabolism and this enzyme specifically allows control of specific reactions in metabolism. Anabolism is the process of constructing complex molecules from simpler ones, which requires energy. This includes forming macromolecules from their smaller building blocks, or monomers, through condensation reactions. For example, proteins are made from amino acids, and starch is made from glucose units. Catabolism, on the other hand, is the breakdown of complex molecules into simpler ones, which often involves hydrolysis—breaking down macromolecules into their monomers. This includes reactions like glycolysis, where sugars are broken down, or the breakdown of fats to release energy. Catabolic processes release energy that the cell can use. 1 Anabolic reacrtions: - Protein synthesis - on ribosomes - Glycogen formation - in muscle and liver - Photosynthesis - in chloroplast → Glycogen formation - anabolic enzyme catalysed reactions of metabolism → Glucose is stored in the lier as glycogen - Specific enzymes catlalysed each step of the process Catabolic reaction: - Hydrolysis of macromolecules in digestion. - digestive enzymes like amylase and maltase ctalyse hydrolisi of large molecules - Oxidation of substrate in respiration. - The matrix of mitochondria contains high concentrations of enzymes and substrates of the Krebs cycle. Enzymes: - Globura proteins - With an active site - A few amino acids interact with the substrate in the active site - Catalysis happens atthe active site of an enzyme Example of a reaction Is it anabolic or catabolic Oxidation of pyruvate in aerobic respiration in Catabolic mitochondria Synthesis of blood plasma proteins in the liver Anabolic Digestion of starch by amylase enzymes in the Catabolic mouth 2 Photosynthesis in the palisade mesophyll of a leaf Anabolic Formation of a glycogen from glucose by the liver Anabolic Describe how several enzymes can link together to form a metabolic pathway - Several enzymes can link together to form a metabolic pathway by catalyzing a series of sequential reactions, where the product of one enzyme's reaction becomes the substrate for the next. Each enzyme facilitates a specific step in the pathway, allowing for efficient conversion of substrates into products while also enabling regulation and integration with other metabolic processes. Suggest a specific example of the interdependence of enzymes in the enzyme controlled reaction pathways of cellular metabolism - A specific example of enzyme interdependence in cellular metabolism is the glycolytic pathway, where enzymes work sequentially to convert glucose into pyruvate. Each enzyme relies on the product of the previous step, demonstrating how they collaborate to efficiently generate energy in the form of ATP. - The digestive enzyme maltase needs its substrate, maltso, which is the product of the enzyme amylase Most chemical reactions did not occur spontaneously in a cell instead cells rely on proteins called enzymes to kick-start chemical reactions and speed them up enabling cells to get the most of the energy sources available to them enzymes have a unique way of kick-starting reactions they work by binding to one or more specific molecules called reactants or substrates binding occurs at a special region on the enzyme called the active site once the substrates bind to the active site they form what's called an enzyme- substrate complex An enzyme substrate complex as the enzyme and substrates begin to react some of the chemical bonds and the substrates begin to weaken causing them to link together eventually the chemical reactions at the active site lead to the formation of a different molecule this is referred to as the product once the reaction has occurred the product is released from the active site the enzyme returns to its original state and is free to react again with another set of substrates 3 Summary - Cells use enzymes to accelerate chemical reactions, facilitating energy utilization by forming enzyme-substrate complexes that yield products. Highlights - Enzymes are proteins that speed up chemical reactions in cells. - They bind to specific molecules called substrates at the active site. - The binding forms an enzyme-substrate complex. - Chemical bonds in substrates weaken during the reaction. - This leads to the creation of a new molecule, the product. - Once the product is released, the enzyme can react again. - This process maximizes energy efficiency in cells. Key Insights - Role of Enzymes: Enzymes are crucial for cellular efficiency, as most reactions in cells do not occur spontaneously. They serve as catalysts, lowering the activation energy needed for reactions. - Active Site Function: The active site is a specific region on the enzyme where substrates bind. This specificity is vital for ensuring that enzymes only catalyze certain reactions, maintaining cellular order. - Enzyme-Substrate Complex: The formation of the enzyme-substrate complex is a key step in the reaction process. This complex stabilizes the transition state, allowing the reaction to proceed more easily. - Bond Weakeness: As substrates interact with the enzyme, their chemical bonds weaken. This is a critical step in facilitating the transformation into products, highlighting the dynamic nature of enzyme activity. - Regeneration of Enzymes: After a reaction, enzymes return to their original state, ready to catalyze new reactions. This regeneration is essential for cellular metabolism, as it allows enzymes to work repeatedly. - Energy Utilization: By speeding up reactions, enzymes enable cells to maximize their use of available energy sources, supporting growth and metabolism in organisms. - Biological Importance: Understanding how enzymes function has implications for biotechnology and medicine, as manipulating enzyme activity can lead to new treatments and industrial applications. 4 Every single second inside every living cell, thousands of chemical reactions are taking place. These reactions are performed by enzymes. An enzyme is a protein that catalyzes a chemical reaction. It initiates the reaction, speeds up the reaction's progress, and makes sure the outcome is always the same. These enzymes often work together to form longer pathways, such as the Citric Acid Cycle, which is a series of chemical reactions used by cells to generate energy from carbohydrates. The essential tasks of life such as metabolism, Protein synthesis, and cell renewal and growth are all regulated by enzymes. The life-sustaining power of enzymes lies in the fact that they catalyze reactions in mild conditions of pH, temperature, and atmospheric pressure. The rates of catalyzed reactions are millions to trillions times faster than those of the same reactions uncatalyzed. To speed up a reaction in the absence of enzymes, additional energy would need to be provided as heat, which is jostles the substrates and occasionally provides Enough energy to trigger a reaction. In the course of most reactions, an unstable and highly energetic transition state is formed as the substrates are transformed into products. An enzyme acts as a template for the reaction, binding to its substrate and holding it in the proper position to form the product. An enzyme also surrounds the substrate with reactive groups that stabilize the transition state, making it easier for the reaction to occur. To understand how enzymes work, let's take a closer look at a reaction in the Citric Acid Cycle that is catalyzed by the enzyme aconitase. Aconitase binds to its substrate citrate and removes a hydroxyl group and a hydrogen atom to form intermediate cis-aconitate. It then adds the hydrogen in the hydroxyl back in slightly different positions to form the product isocitrate. In the active site, some amino acids are perfectly positioned to recognize the substrate and hold it in the optimal position for the reaction to begin. Some amino acids are involved in recognizing and holding the substrate. Other amino acids are directly involved in catalysis. Histidine 101 acts as an acid by donating its proton. Thanks to the chemical environment of Serine 642, it can act as a base by accepting the proton from the substrate. The active site of aconitase also contains an iron-sulfur cluster that stabilizes the substrate electrostatically and helps to position it relative to the catalytic residues. The first step in the reaction is dehydration. In this step, histidine acts as an acid and protonated the hydroxyl 5 On the substrate allowing it to leave as a water molecule. Serine then acts as a base by extracting a hydrogen atom from the opposite side of the substrate forming the intermediate cis-aconitate. Cis-aconitate then flips upside down, and the complementary hydration reaction is performed. In this step, histidine grabs a hydrogen atom from a passing water molecule, placing the resulting hydroxyl group back onto the substrate. Serine then returns its hydrogen atom and the final product isocitrate is released. Notice that the enzyme itself was not changed by the reaction. It extracted the hydroxyl group and a hydrogen atom and then put them back, starting and ending in the same state. This is the hallmark of a catalyst, when it finishes a reaction, it is ready for the next, so it can perform thousands of reactions in a row. Notice also that the shape of an active site is often flexible. Many enzymes surround their substrates, closing around them to form the perfect environment for a reaction. Enzymes are fundamental to life on Earth, 6 Class Notes # 2 Enzymes and activation energy An enzyme serves as a biological catalyst - Enzymes are typically proteins with a specific three-dimensional shape, which includes an active site for substrate binding. - Activation energy (Ea) is the minimum energy required for a reaction to occur. Enzymes lower this energy barrier. Mechanism of Action: ○ Orientation: Enzymes align substrates correctly for the reaction. ○ Straining Bonds: They can put stress on chemical bonds, facilitating their breaking. ○ Microenvironment: The active site may provide an optimal environment for the reaction (e.g., different pH). ○ Stabilizing Transition States: Enzymes may stabilize high-energy transitional forms of the substrate. Impact of Conditions: ○ Temperature: Enzyme activity usually increases with temperature up to an optimal point; high temperatures can denature enzymes. ○ pH: Each enzyme has an optimal pH range; deviations can affect enzyme shape and activity. Biological Importance: Enzymes are essential for various processes, including metabolism, digestion, and DNA replication. 7 Q. 1 Name a specific biological molecule example of a reaction, which begins with one large molecule and ends with two smaller ones? - Glycogen is broken down by glycogenolyisi forming glucose Describe what heppens to the large molecule at the preak of the graph - The peak of the graph indicates the maximum concentration of the point at which the reaction is at the transition state. Explain what is meant by the term ‘activation energy’ - The minimum amount of energy required for a chemical reaction to occur. Compare and contrast the activation energy in the enzyme catalysed reaction and the reaction without enzymes - Enzyme-catalyzed reactions have lower activation energy and proceed more rapidly and specifically, while non-enzyme catalyzed reactions have higher activation energy and are generally slower and less specific. Describe how lowering the activation energy wougld change the rate of the reaction. - Lowering activation energy accelerates the reaction rate by enabling more molecules to reach the transition state and react, often without the need for high energy inputs. 8 Class Notes # 3 Intracellular & extracellular Extracellular : - Digestion of protein begins in the stomach, where hydrochloric acid denatures pritein, and the extracellular enxyme pepsin to break polyppeptide chains - Little groups of cells in the pancreas secrete more digesrive enzymes which hydrolyse proteins into pieces of a new amino acid. These exaple of extracullae enzymes are active outsidde the cell. Intercellular : - There are many enzymes which catalasye reactions inside cells. - Glycolisis (a lineer pathway) in cytolasm - Krebs cycle 9a clinical pathway ) in mitochondria Heat is generated because the reactions are not 100% efficient in energy transfer. In each reaction the changes in energy in the molecules must be negative (exothrmis or near zero to proceed - Reactioond csn br catalysed by intracellular enzymes - Reactions can also be catalsysed by extracellular enzymes - Reactions of the metabolism generate heat 9 Q. 2 Give two examples of extracellular enzymes - Pepsin - Trypsin Suggest two places where extracellular enzymes are synthesised - Pancreas - Fungi How are extracellular enzymes released from the cells which make them? - Exocytosis Intracellar enzymes are found inside the cytolasm and in organelles of wurokaryote cells. Give two examples of metabolic pathways which use intracellulra enzymes? - Glycolisis - Krebs cycle Describe the essential feature which makes a pathway cyclical - Regeneration of strating a molecule Distinguish between a linear and a cyclic matabolic pathway - the process consists of a series of reactions that proceed in a straight line from a starting substrate to an end product, with each enzyme catalyzing a specific reaction in sequence. (final product ) - a series of reactions that regenerate the starting molecule at the end of the cycle, allowing the process to repeat indefinitely. (in a loop) Q. 3 What is an Enzyme? - An enzyme is a biological catalyst that speeds up chemical reactions in living organisms. It is typically a protein (or in some cases, RNA) that lowers the activation energy required for a reaction, allowing it to occur more quickly and efficiently. Define the active site - The active site is the specific region on an enzyme where substrate molecules bind and undergo a chemical reaction. It has a unique shape and chemical environment that allows it to recognize and interact specifically with its substrates, facilitating the catalysis of the biochemical reaction. Explain how an enzyme attaches to a substrate using the lock snd key hypothesis - The lock and key hypothesis explains how an enzyme attaches to a substrate by suggesting that the active site of the enzyme (the "lock") has a specific shape that perfectly fits the substrate (the "key"). When the substrate molecule approaches the enzyme, it binds to the active site due to this 10 complementary shape, forming an enzyme-substrate complex. This precise fit allows the enzyme to catalyze the reaction, converting the substrate into products, after which the products are released, and the enzyme remains unchanged for further reactions. Research how temperature, pH and the concentration of substrate can speed up an enzyme catalysed reaction. What changes when an enzyme becomes denatured? - When an enzyme becomes denatured, its three-dimensional structure changes, leading to the loss of its specific shape, particularly at the active site. This alteration prevents the enzyme from binding to its substrate, resulting in a significant decrease or complete loss of enzymatic activity. Denaturation is often irreversible, meaning the enzyme cannot regain its original structure and function. For each factor complete the table below Factor How can this factor speed up an enzyme reaction? Concentration of substrate Increasing substrate concentration speeds up an enzyme reaction by providing more substrate molecules for the enzyme's active site to bind to, resulting in more frequent formation of enzyme-substrate complexes and a higher reaction rate. This increase continues until the enzyme becomes saturated; beyond a certain concentration, all active sites are occupied, and the reaction rate levels off. pH pH influences enzyme activity by affecting the enzyme's structure, substrate binding, and overall catalytic efficiency. Temperature Temperature increases enzyme activity up to a point, after which high temperatures can cause denaturation and a significant loss of enzymatic activity 11 Measuring reaction rate : - Enzymes are biological catalysts essential for cellular processes. - Self-incompatibility prevents self-fertilization in plants, aiding evolution. - Measuring enzyme rates involves using a color-changing substrate solution. - A spectrophotometer helps track absorbance to calculate reaction rates. - Repeating experiments ensures reliable results for enzyme activity. - Understanding self-incompatibility can enhance plant breeding technologies. - - Enzymes as Catalysts: Enzymes act as biological catalysts, reducing the energy needed for reactions and ensuring they occur efficiently in cellular environments. - Specificity of Active Sites: The unique shape of an enzyme’s active site allows it to bind only to specific substrates, ensuring precise biochemical reactions. - Reaction Acceleration: By lowering activation energy, enzymes drastically increase reaction rates, crucial for maintaining life processes in cells. - Formation of Enzyme-Substrate Complex: The temporary formation of this complex is essential for facilitating the chemical changes, highlighting the dynamic nature of enzyme function. - Reusability of Enzymes: After a reaction, enzymes can be reused multiple times, making them efficient and sustainable in cellular operations. - Role in Metabolism: Enzymes are central to metabolism, influencing energy production and utilization, which are vital for cellular activities. - Implications for Biotechnology: Understanding enzyme mechanisms opens pathways for biotechnological innovations, including drug development and industrial applications. 12 - Lowering temperature results in sufficient thermal energy for the activation of a given enzyme-catalysed reaction to be achieved - Increasing the temperature wil increase the speed and motion of both enzyme and substrate resultingg in higher enzyme activity The effect of pH on the enzyme activity - Changing the shape or charge of the active site will diminish its bailey to bind to the substrate, haltijng enzyme function - Changing the pH will alter the charge of an enzyme 13 Class Notes # 4 Mitochondria; the cell’s powerhouse Summary Mitochondria, the powerhouses of eukaryotic cells, produce ATP through complex protein transport and electron transport systems. Highlights - Mitochondria are known as the cell's powerhouse, producing ATP. - Proteins are transported into mitochondria via specialized translocator complexes. - The inner membrane has cristae housing four protein complexes for electron harvesting. - Coenzyme Q transfers electrons between complexes II and III during respiration. - Proton motive force is created by pumping protons across the inner membrane. - ATP synthase generates ATP as protons flow back into the matrix. - Mitochondria are dynamic, changing shape and location based on cellular energy needs. Key Insights - Powerhouse Functionality: Mitochondria generate ATP, essential for cellular functions, highlighting their role in energy metabolism. - Protein Transport Mechanisms: The intricate transport of proteins into mitochondria emphasizes the complexity of cellular machinery and the importance of protein localization. - Cristae Structure: The invaginated structure of the inner membrane maximizes surface area for electron transport, showcasing evolutionary adaptations for efficiency. - Role of Coenzyme Q: This lipid-soluble molecule is crucial for electron transfer, linking different complexes and demonstrating the interconnectedness of cellular processes. - Proton Motive Force: The electrochemical gradient is vital for ATP synthesis, underscoring the coupling of electron transport and energy production. - ATP Synthase Mechanics: The physical movement and interaction of components in ATP synthase illustrate the intricate nature of biochemical machinery. - Mitochondrial Dynamics: Their ability to change shape and position reflects a responsive system that meets cellular energy demands, with implications for cell health and death. 14 Class Notes # 5 Cellular respiration Summary: - Mitochondria are essential for cellular respiration, converting food and oxygen into ATP, the energy currency for life. Highlights: - Mitochondria are the "powerhouse of the cell," crucial for energy production. - Cellular respiration transforms glucose and oxygen into ATP, vital for bodily functions. - ATP acts like a rechargeable battery, powering processes like maintaining homeostasis. - Aerobic organisms need oxygen for energy; anaerobic organisms can survive without it. - Cellular respiration involves glycolysis, the citric acid cycle, and oxidative phosphorylation. - The electron transport chain creates a proton gradient, fueling ATP production. - Water is a byproduct of cellular respiration, formed when electrons bond with oxygen. Key Insights: - Mitochondria's role in ATP production emphasizes their importance in energy metabolism and overall cellular function. - Glycolysis serves as the initial step in energy conversion, illustrating how cells manage energy sources efficiently. - The distinction between aerobic and anaerobic respiration highlights the diversity of life and metabolic pathways. - The citric acid cycle showcases the intricate biochemical processes that sustain life through energy extraction. - The electron transport chain's mechanism reveals the sophistication of cellular energy conversion systems. - The rapid ATP production rate demonstrates the efficiency of cellular respiration, essential for sustaining life. - The formation of water as a byproduct underscores the interconnectedness of metabolic processes and environmental cycles. 15 - Energy is converted to a usable form in cell respiration - ATP is made most commonly when oxidative phosopholylation is used. The key component in this process is ATP synthase. - This enzyme sits embedded within the inner membrane (cristae) of the mitochondria. Reduction = electron are gained - Oxygen is removed - Hydrogen is gained Oxidation = Electrons are lost - Oxygen is added - Hydrogen is lost Magnification formula: (all the units need to be the same size) - Image size / actual size - Image size/ magnification - Magnification x actual size Electron tomography is a technique for obtaining 3D structures of sub-cellular structures using. 3− Phphorylation is a reaction where a phosphate group (𝑃𝑂4 ) is aded to an organic molecule. The Phosophyrlated molecule is less stable and therefore reacts more easily in the metabolic pathway Glycolisysis occurs in the cytoplasm. A hexose sugar is phosphatelyated using ATP. The hexose phosphante is then split into two triose phosphate. Oxidation occuts removes hydrogen - Pyruvate enters the mitochondrion matrix - Enzymes remove one carbon dioxide and hydrogen from the pyruvate. + + 𝑁𝐴𝐷 ⇔ 𝑁𝐴𝐷𝐻 + 𝐻 𝐶6 𝐻12𝑂6 + 𝑂2 = 𝐶𝑂2 + 𝐻2𝑂 - Fatty acids have a long chain of carbon atoms - CoA can oxidise this chain - Break it down 16 - Acetyl CoA enters the krebs cycle - Acetyl group (2C) joins 4C sugar to from a 6C sugar ]oxidative decarbonisation of the 6C sugar to a 5C compound produces CO2 17 Class Notes # 6 HL Glyxoliss link reaction & Krebs Cycle What is Posphoryation? - Posphorylation is adding phosphate to a molecule. ADP is phosophorylated to make ATP + Reduction of 𝑁𝐴𝐷 - NAD picks up a hydrogen ion and becomes reduced NAD + − 𝑁𝐴𝐷 removes two hydrogen atoms from the molecule being oxidized. It picks up a 𝐻 ion and becomes + reduced and while another H atom becomes a 𝐻 ion which is released into the solution Glycoliss → link reaction → Krebs Cycle → Electron transport chain → Oxidative phosphorylation Reaction Description Glycolysis example Oxidation Hydrogen is removed Oxidation of triose phosphate Reduction Hydrogen is added + Reduction of 𝑁𝐴𝐷 to make NADH Phosphorylation Phosphate is added Phos of glucose to make glusose - 1, 6- bisphosphate Dephosphorylation Phosphate is removed Depho of triose phosphate What lysis? - Lysis is the splitting of a large molecule into two smaller ones This reaction is one sttep of glycolisis - this reaction is caralysed by a specific enzyme Glycolisis takes place in the cytoplasm Glucose is broken down into pyruvate Glucose is phosphorylated making it unstable Decarboxylation is the removal of carbon dioxide from a molecule. Many stps in the breakdown of glucos involve decarboxylation. E.x The conversion of pyruvate to acetyl in the lik reaction Decarboxylation removes a “carboxyl group’ replacing it with a hydrogen atom 18 In aerobic respiration the link reaction follows glycolisis. Pyruvate is converted into acetyl-coA in the link reaction. Acetyl-CoA enters the krebs cycle in the mitochondria. + Pyrivate is decarboxylation and oxidised 𝑁𝐴𝐷 is reduced forming NADH one molecule of CO2 in produced the actyl compound is attached to coenzyme A to form acetyl coenzyme A (acetyl- CoA) Class Notes # 7 HL Electron transport chain Summary - Mitochondria generate energy through the electron transport chain, utilizing a proton gradient to synthesize ATP, crucial for cellular functions. Highlights - Mitochondria are double-membraned organelles vital for energy production. - The inner mitochondrial membrane maintains a proton gradient essential for ATP synthesis. - ATP synthase converts proton flow into ATP, powering cellular reactions. - The electron transport chain consists of four protein complexes that transfer electrons. - Complexes I and II channel electrons from NADH and FADH2 to coenzyme Q. - Complex IV reduces oxygen to water, completing the electron transport process. - Oxygen is crucial as the final electron acceptor, enabling ATP production. Key Insights - Mitochondrial Functionality: Mitochondria are essential for energy production in eukaryotic cells, underscoring their role as the “powerhouse” of the cell. They perform this through a series of chemical reactions that produce ATP from nutrients. - Proton Gradient Maintenance: The inner mitochondrial membrane’s ability to maintain a proton gradient is crucial for ATP synthesis. This gradient drives the ATP synthase, highlighting the importance of membrane structure in energy production. - Role of ATP Synthase: ATP synthase operates similarly to a turbine, converting the kinetic energy from protons flowing through it into chemical energy stored in ATP. This analogy emphasizes the efficiency of biological energy conversion. 19 - Electron Transport Chain Dynamics: The four protein complexes in the electron transport chain work in tandem to transfer electrons and pump protons, which illustrates a highly coordinated system that fuels ATP production. - Complex I and II Contributions: Both complexes I and II play significant roles in the electron transport chain by channeling high-energy electrons from metabolic byproducts, showcasing the interconnectivity of metabolic pathways. - Final Electron Acceptance: Complex IV’s conversion of oxygen to water illustrates the critical role of oxygen in cellular respiration, emphasizing why aerobic organisms rely on it for survival. - Significance of Oxygen: The necessity of oxygen as the final electron acceptor in the electron transport chain highlights the interconnectedness of respiration and energy production, emphasizing its importance in cellular metabolism. 20 Class Notes # 8 Photosynthesis - The calvin cycle Summary The Calvin cycle transforms carbon dioxide into glucose in plants, creating sustainable energy through a series of efficient biochemical processes. Highlights Carbon dioxide (CO2) from the air is essential for plant energy production. The Calvin cycle is a sustainable production line for glucose. Rubisco enzyme initiates the carbon fixation process. ATP and NADPH provide the energy and hydrogen needed for sugar production. The cycle efficiently recycled materials to continuously produce RuBP and glucose. Nature’s cycles enhance efficiency in energy use and resource management. Key Insights Carbon Capture: The Calvin cycle is crucial for converting CO2 into usable sugars, showcasing how plants harness atmospheric carbon for energy. This process is vital for the Earth’s carbon cycle. Energy Transformation: Photosynthesis involves two stages, with the Calvin cycle representing the second phase that captures sunlight energy in chemical form, emphasizing the importance of ATP and NADPH. Sustainable Production: This cycle acts like a factory, efficiently converting raw materials into energy-rich molecules, illustrating nature’s ability to sustain life through biochemical processes. 21 Molecular Collaboration: The simultaneous operation of multiple cycles ensures a steady supply of G3Ps, highlighting the interconnectedness and efficiency of biological systems in producing essential compounds. Resource Recycling: The Calvin cycle’s design emphasizes the importance of recycling, enabling plants to reuse components and minimize waste, which is vital for ecological balance. Solar Energy Utilization: The process underscores how plants use solar energy to drive chemical reactions, providing a foundation for life on Earth by transforming sunlight into food. Biodiversity and Efficiency: The presence of numerous cycles in nature enhances efficiency and adaptability, allowing ecosystems to thrive and optimize resource use across various environments. 22 23