Water Pollution - Characteristics of Natural and Contaminated Water PDF
Document Details
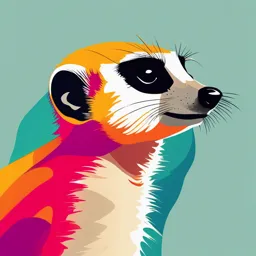
Uploaded by UseableFermat9903
UPNA
Ruben Garayoa
Tags
Related
- Water Pollution Prevention & Control PDF
- Water Pollution: Causes, Effects & Control PDF
- Chapter 7 Water How Do We Use It and Affect Its Quality PDF
- Water Pollution - UEN008: Energy and Environment - PDF
- Water Pollution Assessment and Control (ENVS 525-241) PDF
- Water Quality, Environment, Management, and Technologies PDF
Summary
This document provides a detailed overview of water pollution, covering the characteristics of natural water and the various factors that contribute to water contamination. It explores the hydrological cycle, different types of contaminants, and the impact of human activities on water quality.
Full Transcript
Unit 1: Water pollution Lecture 1. Characterization of natural and contaminated water. 1.1. Characteristics of natural water. 1.2. Characteristics and parameters of contaminated waters. 1.2.1. Physical contaminants. 1.2.2. Chemical contaminants. 1.2.3. Biological contaminants....
Unit 1: Water pollution Lecture 1. Characterization of natural and contaminated water. 1.1. Characteristics of natural water. 1.2. Characteristics and parameters of contaminated waters. 1.2.1. Physical contaminants. 1.2.2. Chemical contaminants. 1.2.3. Biological contaminants. 1.1. Characteristics of natural water More than 97.2 % of the water is on the oceans and is not usable. From the 2.8 % usable, almost 2 % is ice or water vapor. As a result, only 0.6 % of the water can be used by humans (8.4 km3). The hydrological cycle The cycle starts with the water evaporation and later precipitation (ca. 5.2 *105 km3/year). Only 20 % of this water falls into the continents. Of this, 63 % is again evaporated and 37 % is transferred to runoff. Over the ocean, evaporation is higher than precipitations (9 %). This percentage is balanced over the continents where precipitations are higher than evaporation (9 %). Human actions have an impact on the water cycle: ‐ Contamination and diseases can be extended to different areas. ‐ Atmosphere and soil may have an impact on the quality of water. H2O: covalent bonds (intramolecular): between H and O atoms (~100 kcal/mol). Hydrogen bonds (intermolecular): between H and O atoms from different molecules (between 1 and 40 kcal/mol). Characteristics of the hydrogen bonds: They are formed because of the high electronegativity of oxygen, which attracts the electronic density. They are very important because they give water its important characteristics, which are fundamental for life. They give water unexpectedly high melting and evaporation points. They are the cause why ice has smaller density than liquid water and the reason why frozen water is only solid over the surfaces and upper layers of lakes during wintertime. Life can continue below ice. When temperature is increased, hydrogen bonds start to break but several bonds remain on liquid water. Density decreases with temperature because more hydrogen bonds are broken. This causes specific heat of water to be high. High melting, vaporization heats and specific heats are the causes why water works as a perfect thermic regulator and controls Earth’s temperature between precise limits. Specific heat: the amount of heat required for causing 1 g of water to increase its temperature by 1 °C Raining water also has dissolved gases (nitrogen, oxygen, CO2), its pH is slightly acid (5.7) and when flowing in rivers it dissolves salts (low mineralization). Differences between runoff and underground water Some of the most typical characteristics of groundwater are: weak turbidity constant temperature and chemical composition almost complete absence of oxygen. Circulating groundwaters can have significant variations in the composition with the appearance of pollutants and various contaminants. Groundwater is often very pure microbiologically. The geological nature of the soil determines the chemical composition of the groundwater. Natural water contains different substances because of its dissolving and erosive potential. These substances are in suspension, in dissolution, or in colloidal form. ‐ Gases: atmosphere (CO2, N2, O2) and metabolic products of living organisms: aerobic (CO2, O2) and anaerobic (CO2, CH4, H2S, N2) decomposition. ‐ Ions: material dissolution (Ca2+, Na+, Mg2+, etc.), anions (HCO3‐, SO42‐, NO3‐, etc.), material decomposition (PO43‐, NO3‐, NH4+, etc.). ‐ Inorganic substances: sand, clay. ‐ Organic substances: amino acids, fatty acids, natural pigments, etc. 1.2. Characteristics and parameters of contaminated waters Water pollution: Water is contaminated when its composition or physical condition is altered directly or indirectly as a consequence of the human activity. As a result, this altered water cannot be used with the same purpose than natural water. Consequences: ‐ Contamination is measured as a function of the water final use. ‐ Contamination is directly or indirectly generated by the humans. Types of water pollution: ‐ Concentrated: It is localized in a certain point (e.g. effluents from an industry) ‐ Diffuse: Typically coming from agricultural uses. Water contains pesticides and fertilizers and can be drained into aquifers and groundwater flows → pollution becomes extended in an uncontrolled way. 1.2.1. Physical contaminants. Total matter: it is composed of matter in suspension and substances that can be removed by filtration. Suspended matter is the main responsible for: ‐ Colour, reduction of the amount of sunlight that reaches water layers below the surface, sediments deposition. Substances that can be removed by filtration are responsible of: ‐ Salinity, O2 solubility, toxicity. Some quantifiable magnitudes: ‐ Matter in Suspension (MS) or Solids in Suspension (SS). ‐ Dry residue (DR) at 105 oC or total dissolved solids (TDS). ‐ Fixed residue. Odour: contaminated water has a characteristic odour due to the decomposition of the organic matter. Colour: the possible reason is the presence of organic and inorganic compounds. These compounds could be natural or anthropogenic. Urban wastewater: grey colour (recent wastewater), black colour (microorganisms have decomposed the waste). Industrial wastewater: different colours depending on the contaminant Temperature: it has a great influence on water quality Decreases gases solubility (O2). Increases microorganism activities (kinetics of the reaction). Both factors may be combined and increase the effect of each other (synergistic effect): ‐ Favours the proliferation of water organisms (plants and fungi). 1.2.2. Chemical contaminants Organic chemical contaminants: Organic Matter (O.M.). Biodegradability. The source could be natural or urban/industrial. Natural source: from plants and animals. Biodegradable. Urban/industrial source: Organic chemical products. Biodegradable and non biodegradable. Examples: Detergents: active agents (surfactants) and complementary components. Active ingredients (10‐15%): surfactants. Create foams and increase chemical oxygen demand (COD) because they are organic compounds. Co‐adjuvants: anti‐calcareous (poli‐phosphates, zeolites, carbonates, etc.). Additives: bleaching agents (perborates, NaClO), antifoam agents, etc. Pesticides: chemical products used to eliminate parasites in agriculture, cattle, pets…, etc. Formulation: active agent + other substances. Inorganic and organic (more used) pesticides: ‐ Chlorinated hydrocarbons (dichloro‐diphenyl‐trichloroethane (DDT), chlorobenzene). ‐ Organophosphates. ‐ Carbamates Polychlorinated biphenyls (PCBs): they are stable at high temperatures; not flammable; have low electric conductivity; acid, basic and oxidant resistance, etc. They are highly inert and because of this they are persistent and bio‐accumulative. They can produce or increment halogenated levels. Hydrocarbons (HC): saturated hydrocarbons (alkanes), unsaturated (olefin), cycloalkanes and aromatic compounds. General characteristics: low solubility in water, lower density than water and biodegradability. Regarding contamination: ‐ HC decrease the oxygen dissolved. Superior organisms need 2 mg O2/L in water. Usually superficial water (T=25 ºC) is oxygen saturated (9.1 mg O2/L) ‐ They may be toxic. ‐ Because of their density and solubility, HCs create one layer on the water surface that prevents light from passing and inhibits oxygen diffusion through it. There are μorganisms in water and when there is organic matter, biodegradation takes place: ‐ Aerobic process. Products are CO2, NO3‐, SO42‐, H2O. Stable and relatively inert products. But when there are high amounts of organic compounds, O2 is consumed and then: ‐ Anaerobic process: Hydrogen sulphur, CH4, NH3, amines… Unstable and undesirable. Parameters for the evaluation of the organic contamination: ‐ Biochemical oxygen demand (BOD, mgO2/L). ‐ Chemical Oxygen demand (COD, mgO2/L). ‐ Total Organic Carbon (TOC, mgC/L). ‐ Total Oxygen Demand (TOD, mgO2/L). Biochemical oxygen demand (BOD): it is the amount of oxygen consumed by microorganisms in the degradation of the organic matter under aerobic conditions. This magnitude gives the amount of oxygen necessary to biologically stabilize the organic matter (O.M.) in water. It is a biological process → it is slow. BOD5, a t = 5 days. BOD20, t = 20 days. BODT ‐> Total Biological Oxygen Demand BOD5 represents around 60‐70 % of the BODT Standard values: Highly pure waters, BOD5 < 3 mgO2/L. Contaminated waters. BOD5 > 8 mgO2/L. Urban wastewaters, BOD5 100 – 400 mgO2/L. Agrifood industry wastewaters, BOD5 10.000 mgO2/L. Limitations: ‐ Very slow analysis (at least 5 days). ‐ It only determines organic biodegradable matter ‐ It is necessary to carry out pre‐treatments to measure it. The micro‐organisms in the sample consume the oxygen and generate CO2 in the process. This is absorbed with NaOH. This will cause a vacuum pressure, which can be determined as a measured value in mg BOD/L. The sample volume used regulates how much oxygen is available in order for full BOD to run. With different volumes, measuring ranges up to 4,000 mg BOD/L can be measured. Chemical Oxygen Demand (COD): It is the amount of oxygen consumed by chemical reactions in the organic matter degradation. With this magnitude, all organic matter (biodegradable and not) and inorganic matter that can be degraded by oxidation are measured. COD > BOD Limitations: ‐ It is not specific, and measures b.O.M., n.b.O.M. and I.M. ‐ It is not precise. Advantages: in 2h it is possible to get information about the O.M. Information from the BOD5/COD ratio: ‐ Biodegradability values close to 1 → wastewaters with high biodegradability values close to 0 → wastewaters with low biodegradability ‐ Types of water: BOD5/COD = 0.4 – 0.8 → wastewaters from urban sources BOD5/COD < 0.2 → wastewater from industrial sources General parameters serving as indicators of the contamination: Turbidity: Interference to the light passing through the water. Causes for turbidity are mainly insoluble matter, matter in suspension and dissolved matter in water that causes light dispersion (scattering). It is measured in Nephelometric Turbidity Units (NTU’s). Conductivity: it is the capacity to conduct electricity. It depends on the concentration of ions in water. Hardness: It is due to the presence of certain compounds that contain Ca2+ and Mg2+, but also Fe2+ and Mn2+ cations. Anions usually are (HCO3)‐ and (SO4)2‐ Temporary hardness: sum of Ca2+ and Mg2+ bicarbonates. They can be removed by boiling the water or by adding lime (water softening process), Ca(OH)2. Permanent hardness: It is caused by Ca and Mg salts (different from bicarbonates, typically sulphates and chlorides) It is not affected by heating → permanent hardness. Total hardness: total concentration of Ca2+ and Mg2+, expressed as mg CaCO3/L (ppm of CaCO3) The reaction: Is affected by the concentrations of the species in the water. CO2 solubility is dependent on the temperature. CO2 solubility in water at 20 ºC: ~1600 mg/L CO2 solubility in water at 60 ºC: ~ 550 mg/L (around 1/3) This is what happens inside kettles, boilers, dishwashers and washing machines and results in the formation of calcium carbonate deposits on their heating elements. This is usually called limescale in everyday language. Limescale reduces heat transfer from the element to the water and makes the appliance less efficient. pH: magnitude used to express the acidity and alkalinity pH = ‐log[H+], in aqueous solutions it ranges between 0 and 14. Acidity: related to the capacity of water to absorb hydroxyls (OH‐). Alkalinity: related to the capacity of water to absorb protons (H+). It is important to know the tendency of the water to form calcareous incrustations. Alkalinity: It is defined as the amount of ions present in water that react with H+ to neutralize them. It is mainly caused by (CO3)2‐, (HCO3)‐ and OH‐. The main problem related with alkalinity is that these anions can react with cations, precipitate and obstruct tubes. It is determined by the number of equivalents used for its neutralization. Three reactions and a valorization curve are obtained: pH=8.3, OH‐ neutralization and CO32‐ to HCO3‐ pH=4.5, HCO3 ‐ to CO32‐ Half of the H+ needed for the CO32‐ neutralization are added at pH=8.3 Nitrogen and phosphorus (N,P): They are nutrients of the biologic processes. They can be considered as contaminants when their concentration allows algae growing (eutrophication). Algae growth is limited by N in ocean waters and by P in pond waters. Human activity removes this constraint. N comes from nitrates (agriculture and cattle) and P comes from detergents. Algae consume oxygen when they die and decompose. Nitrogen can be found in water as: organic nitrogen, NH3/NH4+, NO3‐ and NO2‐. Phosphorous as organic‐P, PO43‐ and polyphosphate. Bacterial processes involving N dissolved in water: Organic Nitrogen to ammonia by ammonification or mineralization. Nitrification (oxidation): Nitrification is the biological oxidation of ammonia or ammonium to nitrite followed by the oxidation of the nitrite to nitrate: Heavy metals: All known metals except alkaline and alkaline earth metals. Some are present in high concentrations (Fe3+, Al3+) and others in trace levels (Hg2+) in contaminated waters. They are persistent and enter into the food chain. Pb (< 0.5 mg/dm3): batteries, gasoline, pipes. Nervous system and kidney damages. Anaemia. Hg (< 0.01 mg/dm3): electric devices, paints. Nervous system and kidney damages. Cd (< 0.2 mg/dm3) Summary: Water Quality Index (WQI) is a technique for rating water quality. It is an effective tool to assess quality and ensure sustainable safe use of water for drinking. 1.2.3. Biological contaminants. Part of the organic matter in wastewater is formed by macroscopic and microscopic organisms: animal, vegetal and protist. Regarding water treatment, protists are the most important organisms. They are unicellular or pluricellular but without differentiated tissues (a cellular organizational level intermediate between cells and a complete organism): ‐ Bacteria: food: O.M., oxygen and water. Heterotrophic (consume organic C) and Autotrophic (get C from CO2 for growing cells). Heterotrophic can be aerobic, anaerobic and facultative anaerobic. ‐ Algae: eutrophication. ‐ Virus: parasites. They do not have a principal role in the water depuration. Biological contamination indicators: Coliform bacteria. They are in animal and human intestines. Their presence in a water sample is an indicator of fecal contamination. Indicator of faecal contamination. Biotic indexes. Microorganisms determination in water. Toxicity essays. Carried out with aquatic organisms (e.g., Daphnia magna). It measures the response of these aquatic organisms to the presence of specific substances, residues, or isolated or combined environmental factors. Unit 1: Water pollution Lecture 2. Contaminated water treatments. Water line. 2.1. Contaminated water description. 2.2. Flowcharts. 2.3. Wastewater treatment plant (WWTP). 2.3.1. Pre‐treatment0 2.3.2. Primary treatment 2.3.3. Secondary treatments 2.3.4. Tertiary treatments 2.1 Contaminated water description Water composition must be accurately determined. It is key for its proper management. Depending on its origin, it is possible to differentiate two types of wastewater: - Wastewater from urban sources - Wastewater from industrial sources Wastewater from urban sources: Conventional sewers: Includes water from surface runoff, drainages, and homes wastewater (blackwaters). Separated sewers: better, but more costly Legal framework: four different levels European>National>Regional>Local Home wastewaters normally have constant flows and contamination levels. Usual Contaminants (see Lecture 1): ‐ Matter in suspension ‐ Biodegradable organic matter ‐ Nitrogen, Phosphorus ‐ Dissolved substances Home wastewaters have homogeneous compositions and can be treated systematically in Wastewater treatment plants (WWTP). Some industrial wastewater can be treated as urban wastewater. Wastewaters from industrial sources: their composition depends on the type of industry and on the production method used. Some characteristics: ‐ They may contain non‐biodegradable synthetic organic substances. ‐ There is a wide variety of very toxic chemical compounds (heavy metals, organochlorines, pesticides, cyanide, etc.) ‐ pH values may vary a lot. ‐ They do not contain bacteria and viruses. ‐ They usually have high temperatures. 2.2. Flowcharts. There is not a unique WWTP flowchart, contaminants may be treated by different depurating processes. The process selected will depend on: ‐ Wastewater characteristics and volume to be treated ‐ Contaminants’ properties ‐ Required purity ‐ Economic analysis 2.3. Wastewater treatment plant (WWTP). 2.3.1. Pre‐treatment. Removal of coarse contaminants, grease, sand and particles that can easily sediment. It is necessary to remove these contaminants because they may hinder the subsequent operations. Decreases BOD5 by 30‐40% Treatments: Spillways (overflows) Bar screening, Grit chambers (desanding) Degreasing and deoiling Spillways To evacuate into the nearest watercourse the excess flow over the calculated for the right operation of the sewage treatment plant. Excess flow can be also derived to a holding tank for their treatment in the WWTP afterwards. Rainwater collected during the first 10‐15 min. is contaminated and holding tanks are necessary. This water is later reintroduced to the WWTP. Screening: Bar screens, grids and sieves. The main objective is to retain and separate floating and suspended bulky elements that flow together with incoming wastewater. Objectives: ‐ To avoid later depositions ‐ To avoid obstructions in canals and pipes. ‐ To remove elements that may hinder further treatments (de‐sanding, decanting, etc.) ‐ To increase the efficiency of downstream treatments. ‐ To reduce oxygen consumption in the sewage treatment plant. Indispensable treatment in any sewage treatment plant. Grids (1.2 – 10 cm): different grids regarding: ‐ Grid inclination: horizontal, vertical, inclined and curved. ‐ Separation between sticks: narrow (1.5 cm), medium (1.5‐5 cm) and wide (pre‐smoothing, 5‐15 cm). ‐ Grids cleaning: manual or automatic. Regarding Grid Design, it should be considered: ‐ Separation between sticks: grids in series (thick (coarse), medium and fine). ‐ Number of grids: depends of the type of installation. ‐ Wastewater flow rate: it depends on the sand and bulky elements, on the sedimentation and it also depends on the material that flows through the grids. Dimensions: They depend on the flow rate and head loss (Δh). Calculation of the head loss, two possibilities: Bernouilli or Kirschmer equations (see slides) Sieves (10-3 – 5 cm): as an alternative to the grids. Classification: - Plane and static - Curved and static - Rotating (rotary drums) - With mobile or centrifuged surfaces. Grit Chambers To reduce the number of solids in suspension (sand, clay, etc.). They are tanks where the wastewater incoming flow rate is decreased by increasing the cross section. The process could also be done in the decantation/sedimentation tanks and collect them together with the sludge, but it would complicate the sludge treatment afterwards. Particle characteristics: - They are not putrescent. - Their sedimentation rate is faster than the putrescent organic solids sedimentation rate. Water flow rate has to be lower than the precipitation limit for sand particles but should be above the organic material precipitation limit. Classification of grit chambers: ‐ Grit chambers with horizontal flux ‐ Grit chambers with vertical flux: ‐ Grit chambers with induced flux (aerated): It can be a rectangular grit chamber with air injected or novel designs. ‐ Water flow enters to the chamber tangentially. ‐ Water is forced to follow a spiral trajectory. ‐ Air injection reduces the precipitation rate of the organic material. Degreasing and de-oiling They are carried out with air and with the help of skimmers. They can be carried out simultaneously in grit chambers with aerator systems (degritter-degreaser). The main operational problems are related with the presence of grease and oil: - In narrow grids they can cause obstruction problems - A superficial layer is formed in decanters or decantation tanks. This oily or greasy layer makes sedimentation more difficult because it attracts small organic particles. - The aeration in the activated sludge process is more difficult in their presence. - Sludge digestion is more complicated. - COD is increased. An increase in section induces a decrease in velocity that eases the precipitation. 2.3.2. Primary treatment. It mainly consists of a series of physical treatments for the separation of the thinnest solids in suspension that have not been separated in the pre‐treatment. Treatments: Clarification process (coagulation‐flocculation, sedimentation, flotation) VOC’s removal (gas transfer) Neutralization process (pH control). Clarification process: Three steps: coagulation‐flocculation, decanting and filtration. Coagulation‐flocculation To make wastewater clearer by removing turbidity, which is mainly due to solids in suspension and colloidal materials. The clarification process has three steps: coagulation- flocculation, decanting and filtration. Colloids have small sizes (0.1 – 0.001 μm), they do not sediment by physical processes and they are responsible for turbidity and colour. The colloids are stabilized by electrostatic surface charges that cause repulsive forces that prevent their agglomeration. By electrolytes addition the coagulation neutralizes these surface charges and allows the particles to merge and create floccules. Colloid stability measurement: Z potential between colloid charge within the fluid. The electrolyte reduces the Z potential. Coagulation‐flocculation: coagulation, agglomeration and subsequent sedimentation. Coagulation: agglomeration of colloidal particles by adding coagulants. Electrolytes (Al3+ and Fe3+ salts) are used to reduce the Z potential. Coagulation process. Factors to be considered: ‐ Type of coagulant. ‐ Amount of coagulant. There is a relation between turbidity and the coagulant dose. Previous experimental tests are necessary. ‐ pH value depends on the coagulant type. ‐ Coagulation time. Time necessary for an appropriate mixing. ‐ Temperature. ‐ Stirring rate. ‐ Presence of coagulation nucleation centres. Flocculation: agglomerates are formed by the union of coagulated particles in a mechanic and/or chemical process. Flocculation agents are added to enhance the process. Flocculation agents: Non‐ionic organic polyelectrolytes (polyethylene oxide) cationic (polyamines) anionic (polyamide) Sedimentation One of the most important processes in wastewater treatment. It is the separation by gravity of particles with higher specific weight than water. Depending on the concentration and the interaction between particles, there are four types of sedimentation mechanisms. They can occur simultaneously. ‐ Particles that do not change their shape, weight and size during sedimentation (discreet particles): particles that sediment without interaction between them. ‐ With flocculation ‐ Delayed or by zones: interaction between particles. Bigger particles sedimentation. ‐ Compression: particles concentrated in one mass. The compression is originated by the weight of the particles. Analysis of discreet particles sedimentation Maximum velocity of a particle to settle down. For laminar regimes (Re < 0.3) → Stokes’ Law (sedimentation rate r proportional to the squared particle diameter d2): r = g∙(ρs‐ρ)∙d2/18μ μ: viscosity of water Retention time = 2‐3 h, 60‐70 % suspended solids are removed, 25‐40 % of the BOD5 is removed. The deposited solids are highly putrescent and diluted; they must be stabilized by aerobic or anaerobic treatments and dried. Design of sedimentation tanks: Particles removed by sedimentation will be those that present a final vertical rate equal or superior to q0 (overflow rate). The rate of clear water is: Q = A∙q0 A = surface area of the tank Thus, the clear water flow capacity is independent of the tank height. The depth of the basin is not a factor in determining the size particle that can be removed completely in the settling zone. The determining factor is the quantity Q/A, which has the units of velocity and is referred to as the overflow rate q0. The overflow rate q0 is the design factor for decanters and corresponds to the terminal settling velocity of the particles for a removal efficiency of 100 %. Sedimentation devices: Sedimentation tanks with scratching: rectangular horizontal flow tank and circular radial‐flow tank Flotation Small gas (usually, air) bubbles are introduced to separate very small and light particles. Particles adhere on the bubble and accumulate on the liquid surface. With flotation devices it is easier and faster to remove small or light particles with slow deposition rate. Once accumulated on the surface they can be collected by superficial skimming. Bubbles can be added or induced by: ‐ Flotation by dissolved air: Air is injected under pressure and then released by pressure reduction. Two types: a) total flux b) flotation with recirculation ‐ Flotation by aeration. ‐ Flotation by vacuum. Gas transfer Gas transfer between phases, usually from gas to liquid phase: ‐ O2 supply for aerobic biological treatments. ‐ Drag or desorption of Volatile Organic Compounds (VOCs). ‐ Drag of inorganic contaminants: NH3, H2S, etc. O2 supply can be done with air or pure oxygen. Gas desorption: Volatile Organic Compounds (VOCs) in wastewaters are present in a dissolved form (e.g. trichloroethylene). VOCs concentration can be decreased by two mechanisms: ‐ Volatilization: VOCs migrate to the water surface by diffusion and then vaporize to the atmosphere. ‐ Gas dragging: A different gas (oxygen, air, etc.) is injected to facilitate the volatilization of the VOCs. Neutralization processes Last step before the wastewater is transferred to the secondary treatment. Additional pH adjustment can be needed at various points of the installation. pH control: Issues to consider: ‐ Previous step to other processes: precipitation, coagulation‐flocculation, biological process. ‐ Final step before wastewater is discharged to the secondary treatment. acid + base => salt + water ‐ pH control of acid waters in stirring tank→ Bases addition (CaO, MgO, NaOH, etc.). ‐ pH control of basic waters in stirring tank → Acids addition (mineral acids, CO2, etc.). ‐ pH control by addition in multiple steps. Problems associated with the pH control of wastewaters in one step: ‐ The pH value does not have a linear relation with the reagents concentration or with the flow, specially at pH=7. ‐ The variation in pH values can be very fast. ‐ Wastewater flows can experiment fluctuations. ‐ Small volumes of reagents are mixed with large wastewater flows (volumes). 2.3.3. Secondary treatments The main objective is to remove, stabilize or transform biodegradable organic matter in wastewater by sedimentation or biodegradation. These treatments reduce BOD and stabilize biodegradable organic matter. Microorganisms are used. Main concepts about biological processes Elimination mechanisms of the organic matter: Organic matter appears in wastewater as: colloids, solids in suspension and dissolved. There is a biodegradable fraction and another not biodegradable. ‐ Physical adsorption: biodegradable organic matter (colloidal and in suspension). ‐ Air dragging VOCs. ‐ Biodegradation. Aerobic and anaerobic processes. To convert biodegradable matter into simpler products Microorganisms. Bacterial metabolism Like any other living organism, bacteria need to live: energy carbon (to synthesize new organic matter) inorganic elements (nutrients). ‐ Energy and carbon sources: heterotrophic organisms obtain carbon from organic matter and energy from oxidation chemical reactions. ‐ Inorganic elements: nitrogen and phosphorous. Biological growth: Two simultaneous processes during biodegradation: 1. New cellules and microorganisms synthesis: O.M. + O2 + (microorg.) + (nutrients) ⭢ (new microorg.) + CO2 + H2O + (insoluble waste) μ, microorg. Gr owth rate, μm maximum microorg. growth rate, (depends on the nutrients concentration until it gets to a maximum μm) ks: "half‐velocity constant"—the value of S when μ/μmax = 0.5 2. Cellular auto‐oxidation O.M. + (microorg.) + O2 ⭢ CO2 + H2O + (nutrients) + (insoluble waste) By this process microorganisms obtain energy (nutrients). Global Process: 1 + 2 (combination of new cellules / microorganisms synthesis and cellular auto‐oxidation) Net microorganisms generation rate is not equal to the substrate removing rate. Characteristics of the biological oxidation: ‐ Biodegradability: depends on the chemical structure of the O.M. Biodegradable compounds: alcohols, phenols, ketones, hydrocarbons, unsaturated compounds, etc. Non-biodegradable: ethers, polymers, paraffins, etc. ‐ Nutrients and pH: Microorganisms need small quantities of mineral nutrients and N and P. pH: 6.5 – 8.5 (depends on the microorganisms). ‐ Microorganisms: bacterial (80% of all μorg. in water). ‐ Small quantities of organic compounds (phenols), heavy metals and inorganic substances (ammonia) can affect them. Processes for the biological treatment Two types of classifications according to: ‐ Use of O2: aerobic (with O2), anaerobic (without O2), anoxic (anaerobic with nitrates and sulphates as oxidation agents) and combinations. ‐ Microorganisms placement: suspension culture (μorg. dispersed in a reactor), fixed culture (μorg. attached to an inert support) and combinations. Combinations of the above. Aerobic treatments in suspension: aerated ponds, stabilization tanks, and activated sludge processes. Aerobic treatments in fixed culture: trickling filters and rotating contact reactors. Aerobic treatments in suspended culture Aerobic treatments in suspension (low cost): aerated ponds, stabilization tanks Aerated ponds: large areas (≈ 5000 m2), depths between 1 to 5 m. Hydraulic residence time (τ=V/Q) between a few days and two weeks. Two types of aerated lagoons: aerobic and facultative. No stirring. Two zones: aerobic and anaerobic. Stabilization tanks: larger areas (≈ 60.000 m2) depths between 1 to 2 m. Hydraulic residence time (τ=V/Q) of 40 days. Three types of stabilization tanks: aerobic, anaerobic and facultative. Facultative processes: can work both in aerobic and anaerobic conditions Advantages: ‐ Natural process. ‐ Infrastructure with low cost (maintenance and construction). ‐ Stabilized sludge. Disadvantages ‐ Large areas and unpleasant odour. ‐ The depuration yield depends on the season of the year and on the weather conditions. Aerobic treatments in suspension: activated sludge process. Organic matter stays in suspension with a continuous oxygen supply. BOD decreases. Yield ~ 90%. This is the most used treatment. After 8 hours microorganisms are separated and reused. Criteria used in the selection of the type of biological reactor: ‐ Kinetics of the depuration reactions. Yield. Substrate consumption rate: ‐ Oxygen Transfer In stirred tanks the oxygen is transferred by agitation In tubular reactors there can be certain zones without oxygen. ‐ Wastewater characteristics: Urban wastewaters: high biodegradability. Filamentous organisms may appear that precipitate with difficulty, specially at high concentrations. Industrial wastewaters: with toxic compounds for the microorganisms. This effect is reduced by dilution ‐ Others: Temperature, pH, alkalinity, construction costs Aerobic treatments in fixed culture Wastewater passes through a filter or a porous material. Microorganisms are adhered to this support. Trickling filters: Material: grinded siliceous stones, metallurgical coke, plastic material. bed height: 1.5 – 4 m. Organic load: 0.08 – 6 kgBOD/(m3∙day). Hydraulic load: 1‐200 m3/(m2∙day). BOD decrease: 50‐90%. To be effective the diameter must be around 50‐60 m. Mechanism: Advantages: Low cost for the O2 supply (natural process); less sensitivity to organic loads. Disadvantages: filter obstruction; complex process. Rotating contact reactors (bio‐discs or bio‐cylinders): The system is very similar to the trickling filter, but it is applied to lower hydraulic loads. Circular plastic structures arranged around a horizontal axis. Their rough surface allows the attachment of microorganisms. They are partially sunken (40‐90%). Aeration by rotation. Advantages: low areas, low solids production, low energy consumption. Disadvantages: Temperature control and rotational speed. The turbulence created during rotation enhances the degradation because of the extra aeration. They are suitable for medium ‐Small urban wastewater facilities. 2.3.4. Tertiary treatments Tertiary treatments remove contaminants that have not been treated before. These treatments are more aggressive than previous treatments. The goal of tertiary treatments is to remove unwanted elements such as solids in suspension, COD (solid and colloidal), phosphorus and specific compounds (pesticides, metals, detergents, and so on). They are designed to improve the quality of purified water so that it can be discharged into the natural environment or re‐used. Treatments Non-biodegradable organic matter Dissolved inorganic matter Disinfection. Removal of non-biodegradable organic matter Adsorption on solids: non‐destructive method. Retention of a molecular or ionic species (adsorbate) on the surface of a solid (adsorbent) by physical‐chemical interactions. Adsorbents: activated carbon, silica, clays, zeolites, etc. Activated carbon: carbon materials (organic or inorganic origin) treated to obtain high specific surfaces (500 – 2000 m2/g). Organic origin: wood, coconut, almond shells, etc. Inorganic origin: coal, lignite. Activation: physical (water stream, 800 – 950 ºC) or chemical (H3PO4, KOH, ZnCl2, 500 ºC). Adsorption capacity: ‐ Porosity: micropores (dp 5μm). However, very small particles (< 5 μm) cannot be separated efficiently. They are cheap (low maintenance costs). Electro‐filters Separation of the electrically charged particles by an electric field. This technology acts exclusively on the particles and not on the entire mass of gas. It must be applied under conditions of high gas flow loaded with particles of small size and only if high efficiencies are required. Only for particles that can be ionized, otherwise this technology would not work. Advantages (compared to other gas cleaning technologies): ‐ High removal yields (< 99 %), small particles. ‐ High volumes of gases treated, low pressure drop ‐ Can operate with hot effluents (below 500 ºC) Disadvantages: ‐ High investment costs. ‐ Lack of flexibility at varying operating conditions (humidity, gas composition and T, solids nature). ‐ Large equipment. Operation: ‐ Particles are charged ‐ Migration of the particles towards the surface ‐ Uptake of particles ‐ Cleaning and collection of particles Tubular Electrofilters and with flat planes Bag Filters Filtration through fabric, paper or other materials. Small particles are separated with high efficiency; dry powders removed are collected for further treatment The main function of the filter material is to serve as support for the dust layer (cake) responsible of the high filtration efficiency of small particles. Advantages (compared to other technologies): ‐ High separation efficiency of small particles ‐ Low sensitivity to variations in the particle loads from the effluent ‐ The solid is collected dry ‐ Low pressure drops Disadvantages (compared to other technologies): ‐ Space needed ‐ The filter material may be sensitive to elevated T and acid / base conditions ‐ Special materials are needed for T above 275 ºC ‐ Risk of fire or explosion with easily oxidized powders Wet scrubbers They can simultaneously capture particles AND absorb gaseous and liquid pollutants The use of scrubbers reduces the risk of fire or explosion in the case of flammable or explosive particles. The gas stream is in contact with a liquid; typically, water or using chemicals in an aqueous solution (e.g. amines). Separation mechanism: impact dissolution and condensation. Types of wet collectors (high variety) ‐ Chambers (spray towers) ‐ Fluidized collectors ‐ Cyclonic chambers ‐ Packed towers ‐ Venturi Collectors Unit 2: Air pollution Lecture 6. Management of gaseous pollutants. 6.1 Absorption. 6.2 Adsorption. 6.3 Incineration. 6.4 Catalytic reduction.. 6.1 Absorption. By contacting the contaminated gas with a liquid, the contaminant in the gas is dissolved in the liquid. There is just one requisite: the pollutant has to be soluble in the absorbent liquid. The driving force in the process is the difference in concentration between the liquid and gaseous phases. In most systems and operating conditions, the equilibrium values are ruled by Henry's law: y = (H/P) ∙ x = K ∙ x y: molar fraction of the pollutant in the gas. x: molar fraction of the pollutant in the liquid. P: total pressure. H: Henry's constant. Properties of absorption liquids: ‐ High solubility of the pollutant in the liquid (absorption is accelerated, and less liquid is needed). ‐ Liquid: ‐ Low volatility (losses are reduced and introducing other pollutant in the gas phase is avoided). ‐ Non corrosive. ‐ Low viscosity (transfer rate increases, fewer losses). ‐ Non toxic, non flammable. ‐ Chemically stable Examples: ‐ H2O and hydrocarbons (physical absorption) ‐ H2O / NaOH ‐ H2O / ethanolamine Applications: The following substances can be removed by absorption: ‐ Gases, fumes and inorganic vapors: chromic acid (H2CrO4), hydrogen sulfide (H2S), ammonia (NH3), chlorides, SO2. ‐ Volatile organic compounds (VOCs) especially those soluble in water: methanol, ethanol, isopropanol, butanol, acetone, etc. ‐ Particles and harmful contaminants from the atmosphere. Absorption equipment: Scrubber columns. Packed columns: chemical, metallurgical, food industries, etc. Plate columns: gas desulfurization, control of emissions from the combustion of coal and oil. Packed columns. Filler materials: Selection of the filler material: ‐ They must be inert and chemically resistant materials (glass, ceramic, plastic and steel) ‐ They should have high mechanical strength, support the weight of the column. ‐ They should have high void volume fraction and reduce pressure drop. ‐ High surface area per unit volume, high contact between phases. Filler types: Raschig rings (cheap but not so effective) Intalox and Berl saddles (expensive but effective) Pall rings (homogeneous flow and low ΔP) Plates column. The gas‐liquid contact is not continuous but by stages in each of the plates along the column. The plates increase the residence time, increasing the efficiency. By fixing the flows (slope), the number of plates needed to obtain a certain Y1 can be determined. With the number of plates fixed, the flows (slope) to obtain a certain Y1 can be determined Comparison between packed columns and plate columns. Packed columns: Plates column. ‐ Lower pressure drops (ΔP) ‐ Used with effluents having solids that form ‐ Used with corrosive gases clusters and result in large ΔP inside the ‐ Lower amount of metal parts (plastic or column. ceramic fillers). ‐ Better hydrodynamic behaviour, the liquid ‐ More suitable for treating liquids prone does not follow preferential paths. to forming foams. ‐ They favour heat exchange when needed ‐ Recommended when only small spaces (coils can be incorporated into the plates). are available (column ø between 0.5 and ‐ Recommended for absorption + chemical 1 m) reaction processes: Increased residence time of the absorbent on the plates. ‐ They are recommended when large columns are needed (column diameters > 1 m) 6.2 Adsorption. The contaminated gas is put in contact with a solid, the contaminant is retained on the solid surface. Two types of adsorption processes: ‐ Physical (physisorption) Based on the attracting Van der Waals forces between molecules of the gas and the surface of the solid adsorbent. ‐ Chemical (chemisorption): Chemical reactions occur on the surface of the adsorbent. Properties of the adsorbents (solids): All solids can adsorb gases. To be effective as adsorbents for retaining contaminants, they must have a porous structure and high surface area. Activated carbons, alumina, silica gel, zeolites. Applications: ‐ Removal of organic compounds from gaseous effluents. ‐ Removal of SO2 from gaseous effluents ‐ Removal of other sulfur compounds from gaseous streams (e.g. H2S) ‐ Removal of water vapor ‐ Elimination of solvents and odors in air ‐ Removal of CO2 from natural gas ‐ Separation of mixtures : N2/O2 , CO2/CH4 , H2/CO. Adsorption equipments: Three types: fixed bed fluidized bed rotary bed 6.3 Incineration. Complete destruction of organic contaminants by means of combustion at very high temperatures to form CO2 and H2O. CxHyOz + (x + y/4 – z/2)O2 → x CO2 + y/2 H2O The products of complete combustion: CO2 and H2O. They are not toxic. Highly exothermic reactions: the heat generated can be utilized to preheat the reactants, generate steam, or co‐generating electricity. Destructive process: the organic pollutant is not recovered. (absorption and adsorption are not destructive). Simple and convenient technology, but also strongly criticized: -The energy generated by the combustion is not enough to raise the temperature to the desired levels → fuels such as natural gas are commonly co‐fed. ‐ Incomplete combustion can create pollution problems with the formation of new VOCs. Excess air and very high temperatures are needed to ensure complete reaction. ‐ The presence of elements other than C, H and O, can create problems of contamination and the need for additional purification systems, before or after combustion, such as absorption columns. Combustion devices: Torches: Direct burning of the polluntants. (Flare them) Temperature is very important because the process has to be instantaneous. Thermal incinerator: this is the conventional procedure. Temperatures = 700 ‐ 1200 ° C; residence times = 0.2 ‐ 2 s. Disadvantages: high fuel consumption and possible NOx generation. Catalytic incinerators: It is an alternative with significant advantages: increased reaction rate decreased temperature (in comparison to thermal incineration): 300‐600 ° C reduction of the reactor volume Devices: Torches (flares): when pollutant gases are emitted at concentrations above the flammability limits and at variable flow rates. (Last option) Incinerators: When gases have low contaminants concentration and the gas mixture is below the lower flammable limit. It produces sludges and ashes. 6.4 Catalytic reduction. It is a technology for NOx removal. Nitrogen oxides are reduced to N2 and H2O. Depending on the mechanisms of formation, NOx can be: ‐ Thermal: Formed from high temperature air. ‐ Prompt: from hydrocarbon radicals reacting with N2. ‐ Structural: from N compounds which may be contained in the contaminants or in the fuel. Molecule inside fuel that contains Nitrogen, if N2 gets oxidized. Structural and thermal NOx can be controlled by tuning the operating conditions. Selective catalytic reduction (SCR): the reducing agent reacts with NOx. Reducing agents: NH3, alcohols and hydrocarbons. A catalyst is needed. 4 NO + 4 NH3 + O2 → 4 N2 + 6 H2O 2 NO2 + 4 NH3 + O2 → 3 N2 + 6 H2O Non‐selective catalytic reduction (NSCR): The reducing agent reacts with NOx and other reagents. Reducing agents such as CO and hydrocarbons. 2 NO + 4 CO + O2 → N2 + 4 CO2 Unit 3: Contamination of soils Lecture 7. Solid Wastes. Definition and Characteristics. 7.1 Definition and classification. 7.2 European Union Common Strategy for Waste Management. 7.1 Definition and classification. Definitions of solid wastes: Materials generated in the activities of production and consumption that have not reached an economic value in the context in which they were produced, due to: - A lack of appropriate technology for its use - A lack of markets for recovered products All materials in solid or paste form that have lost their value and, therefore, they must be disposed of. Full or partial valorization of these wastes can be accomplished with proper management of the wastes. Any substance or object listed in any of the categories from the European Waste Catalogue (EWC) Factors contributing to the generation of waste: - World’s population growth. - Concentration of population in urban areas. - Use of packaging for many products. - Early obsolescence for many items (throwaway/single-use products, etc.). E-waste: Electronic waste NIMBY: Not in my backyard Not all the wastes have the same impact. Problems arising from waste generation and an inadequate management (waste dumping): - Contamination of soil and aquifers. - Emissions of gases (e.g.: CH4, dioxins, ash, etc.) - Visual impact of landfills on the landscape. - Risks of landslides or mudslides due to the displacement of large masses of waste. 7.2 European Union Common Strategy for Waste Management. Directive In Europe, we currently use 16 tons of material per person per year, of which 6 tons become waste. Only a limited share (~36 %) is recycled. Each person produces on average, 500 kg of household waste. Only 40 % of it is reused or recycled. The 7th Environment Action Programme (ending in 2020) sets the following priority objectives for EU’s waste policy: To reduce the amount of waste generated; To maximise recycling and re-use; To limit incineration to non-recyclable materials; To phase out landfilling to non-recyclable and non-recoverable waste; To ensure full implementation of the waste policy targets in all Member States. Main elements of EU waste legislation: Waste framework legislation Waste stream legislation Landfilling and incineration Shipment of waste Implementation and reporting Review of EU waste policy A life‐cycle approach All products and services have environmental impacts, from the extraction of raw materials for their production to their manufacture, distribution, use and disposal. Life‐cycle thinking involves looking at all stages of a product’s life to find out where improvements can be made to reduce environmental impacts and use of resources. The new Waste Framework Directive has introduced the concepts of life-cycle thinking and circular economy into waste policies. Landfilling Landfilling is the oldest form of waste treatment and the least desirable option because of the many potential adverse impacts that can have. However, this may sometimes be the only option left for certain wastes. The most serious impact is the production and uncontrolled release of methane (CH4) into the air, an important greenhouse gas. Each CH4 molecule released contributes to the global warming 25 times more than each molecule of carbon dioxide (CO2). In addition to methane, the decomposition of biodegradable wastes inside landfill sites may release chemicals such as heavy metals, resulting in run‐off streams called leachates. These liquids can contaminate local groundwater, surface water and soil. Uncontrolled landfills (waste dumping) could represent future environmental bombs. Design, construction and operation of landfills must be done carefully!! Energy recovery Modern waste incineration plants can be used to produce electricity, steam and heating for buildings. Waste can also be used as fuel in certain industrial processes. Poor or incomplete combustion of waste materials can result in environmental and health damage through the release of hazardous chemicals, including dioxins and acid gases. Energy recovery through incineration is often not the most efficient way of managing used materials, particularly those that are difficult to burn or which release toxic chemicals at high T. Member States are encouraged to use life-cycle thinking to assess the possible environmental benefits and drawbacks when deciding whether to incinerate waste. Recycling Much of the disposed waste can be recycled. Recycling reduces the amount of waste that ends up in landfill sites, while cutting down on the amount of raw materials needed from the natural ‘environment. The EU has set recycling targets for many types of waste, including old vehicles, electronic equipment, batteries and packaging, municipal waste and waste from construction and demolition activities. Member States are working hard to put systems in place to ensure these targets are met. Re‐use Re‐use involves the repeated use of products and components for the same purpose for which they were conceived. For example, refrigerators, ink cartridges, computer printers… all of them can be refurbished for re‐use. The re‐use of products or materials such as clothes and furniture that would otherwise become waste has social, economic and environmental benefits, creating jobs and making products available to consumers who could not necessarily afford to buy them new. Prevention Good waste management begins by preventing waste from being produced in the first place – after all, what it is not produced does not have to be disposed of. Waste prevention is becoming more and more important as the global population increases and depletes the Earth’s finite supply of natural resources. One of the key tools being used to encourage waste prevention is Ecodesign, which focuses on environmental aspects during the conception and design phase of a product. Eco‐friendly products should be made using recycled secondary raw materials and should avoid the use of hazardous substances. These products should consume less energy during the use phase and should be able to be recycled once they have been discarded. Unit 3: Contamination of soils Lecture 8. Solid Wastes (Municipal solid waste MSW). 8.1 Definitions and characteristics. 8.2 Composition. 8.3 Physical, chemical and biological properties 8.4 Treatment of urban wastes 8.1 Definitions and characteristics. EU Waste Framework Directive (2008/98/EC) Municipal wastes (MW, now labeled as domestic wastes in Ley 22/2011, de 28 de julio, de residuos y suelos contaminados – BOE 181, 29th July 2011 – replacing the former Ley 10/1998, de 21 de abril): Those residues generated in homes, shops, offices and services, as well as those wastes not classified as dangerous, including: Waste electrical and electronic equipment (WEEE), construction debris, clothes, furniture, batteries and street sweeping. Waste generation and composition are important factors in their management. In Europe, annual average generation of MW is around 500 kg/capita In Spain: around 0.8 – 1.8 kg of waste per person are collected each day 8.2 Composition. The composition describes the individual components from the waste as well as their relative distribution. Urban waste includes organic and inorganic materials, which can also be classified as inert, fermentable, metal, hazardous substances, sanitary, etc. Their composition may be very different depending on their origin: domestic, commercial, institutional, municipal, industrial, agricultural, etc. The composition may also be variable, depending on the way of life. As the standard of living increases in a given society, the percentage of organic matter (OM) decreases, while the percentage of elaborated products increases. Components: ‐ Food waste: fermentable materials. ‐ Paper and cardboard packaging: newspapers, boxes, etc. ‐ Plastic bags, drink containers, plastic packaging ‐ Glass bottles, drink containers, etc. ‐ Metal cans and canned soft drinks. ‐ Tetrabricks. ‐ Other: textiles, expired medicines, wood, light bulbs, etc. Other wastes. Waste collection points: Certain wastes can cause serious pollution problems if not adequately collected → they cannot be managed by means of regular recollection services: ‐ Batteries ‐ Various oils and lubricants (industrial machinery, food, etc.) ‐ Various chemicals (solvents, paints, etc.) ‐ Appliances (washing machines, refrigerators…) ‐ Clothes (textil industry) ‐ Fluorescent tubes and bulbs 8.3 Physical, chemical and biological properties In addition to composition, some properties of the wastes must be taken into account for their correct treatment and management: Physical Properties: ‐ Density: it determines the volume levels needed for the collection and transportation equipment, together with the sizes of the deposits, landfill capacity, etc. ‐ Humidity: it affects the processes of transformation, leachate production, incineration treatments, etc. ‐ Particle size distribution: it is important in the recovery of wastes: sieves, trommel screens and magnetic separators. trommel Biological properties: ‐ Biodegradability: biological degradation of the O.M. residues Chemical properties: Several types of tests/analyses. ‐ Elementary (ultimate) and proximate analyses of the residue components: ‐ Percentages of C, H, O, N, S, and ash content (proximate analysis). ‐ Knowing the C/N ratio is important for biological processes (composting) ‐ Heating value: heat produced in their complete combustion. 8.4 Treatment of urban wastes Reuse and recycling: Wastes are processed in industries for bringing the materials back to a condition in which they can serve again their original purpose (reuse) or in any other application (recycling). Recycled materials, two categories of markets: Raw materials for the industry ‐ Paper, cardboard, etc. ‐ Bottles, aluminium cans ‐ Tyres for road pavement ‐ Organic waste: compost, chemicals, etc. Raw materials for energy production: ‐ Organic waste: fuel production. Recycling of organic matter. Composting: Recovery of fermentable organic materials for their use in agriculture as natural fertilizers. The organic fraction of MSW can be converted to fertilizers (compost) by modifying its properties. A biological transformation is carried out under aerobic conditions. Characteristics: ‐ The non‐fermentable materials (glass, etc.) are previously separated. ‐ The fermentation time may vary between 3‐4 weeks (open system ‐ natural fermentation) and 1‐2 weeks (closed system ‐ closed fermentation). ‐ Temperatures can range between 50 and 70 ° C, and aerobic conditions must be secured (by regularly mixing the garbage). Control parameters in composting: ‐ Temperature: controls the biological process and eliminates pathogens. ‐ Moisture percentage: controls the final quality. Must be between 40 and 60 %. ‐ C / N ratio: 25 to 50 %. Ensures cell synthesis and energy supply. ‐ pH: between 5 and 7. Ripening: storing of the material in order to obtain certain properties Recycling of other components: MSW contains components that are not biodegradable and can be recovered. Using recycled materials saves energy and resources (less raw materials). Glass recycling: It does not lose its properties after recycling. Three main types of glass in MSW: Food and beverage containers, flat glass and pressed glass. The separation can be performed by colours: white, green and amber. Stages in glass recycling: ‐ Cleaning and colour separation ‐ Shredding and breaking into glass culets. ‐ Screening ‐ Final processing: melting in furnaces at 1500 ºC → the presence of recycled glass reduces the melting point. ‐ Uses: New containers and bottles, fiber glass, tiles, etc. Recycling of plastics: In MSW: mainly packs of food, drinks and cleaning products. The use of this type of containers has dramatically increased, replacing metal containers, glass and paper. Classification from the viewpoint of recycling: Thermostable: resistant materials, heat‐hardened, not recyclable. Thermoplastics: softened with heat and can be recycled. Plastics that are separated for recycling: ‐ Polyethylene terephthalate (PET): recycled into manufacturing polyester fibbers, and pillows. ‐ Polyvinyl chloride (PVC, used in wire covering, mouldings, profiles, etc.): recycled as raw material for manufacturing pipes, toys, etc. ‐ Polypropylene (PP, used in caps, bottle labels…): can be recycled for serving the same purpose again ‐ Polystyrene (PS): used for food trays. Incineration: Thermal process applied to solid wastes for energy recovery: A hot product gas stream is obtained → Energy can be recovered in heat exchangers. Ash residues are also obtained. Advantages: Disadvantages: ‐ Potential for energy recovery. ‐ Incomplete elimination of wastes, landfilling ‐ Wastes of very different is still required (though in less nature can be treated easily proportion). (hazardous, sanitary…) ‐ The gases must be treated: highly toxic and ‐ Can be easily implemented in pollutant the urban environment. ‐ External energy input is required ‐ Sometimes it may require of an alternative treatment system coupled to it Parameters to be controlled for complete combustion: ‐ Residence time of the wastes inside the incinerator. ‐ Oxygen‐to‐waste ratio. ‐ Temperature. Due to the heterogeneous nature of the wastes, it is almost impossible to burn with stoichiometric amounts of air. In practice, an excess of air is used and also turbulence (solid‐gas contact and mixing are favoured). By regulating the air flow the combustion temperature can be controlled. The temperature of the flue gases can also control odours and avoid the release of toxic compounds: T < 790 ° C: the emission of odorous compounds can occur. T > 980 ° C: dioxins, furans, etc. are minimized. Components of MSW incineration plant: The wastes are burned at temperatures between 950 and 1200 °C in furnaces: (mainly grates and rotary kilns). Firing grates: Two main streams are generated: solid waste (bottom ash and fly ash) and gases. Rotary ovens (kilns): Three main streams are generated: ashes, wastewater and gases. Air pollutants from MSW incineration: NOx: formed at high temperatures and in excess of oxygen. SOx: formed by the presence of materials containing S. CO: formed in areas at low temperature and in defect of oxygen. Particles: formed by the incomplete combustion of fuel and from non-combustible materials. Metals: due to the heterogeneity of MSW. Acid gases: HF and HCl (derived from certain plastics like PVC) (when burned can form hydrochloric acid) Dioxins and furans: some isomers among the most toxic substances. Sources: ‐ They can be naturally present in the wastes ‐ formation during combustion: usually generated in cold areas (250‐400 °C) of the post‐combustion chambers Landfills: Procedure for disposal of MSW or final treatments (e.g.: ash from incineration). Waste dumping (uncontrolled, illegal in the EU): several problems caused by rodents (mices, rats, etc.) and insects, risk of uncontrolled fires, odours, water and air pollution. Controlled Landfilling: Avoids most of the problems from free dumping. Management of non‐hazardous, inert and hazardous wastes. Reactions between components of the waste yield gaseous and liquid products. These effluents must be collected and treated. It is necessary to do a location study, especially hydrogeologic, for building up new landfills in environmentally safe places. Advantages of MSW landfill management: Disadvantages of controlled management of ‐ Easy implementation MSW landfills: ‐ Low installation and operation costs ‐ Resources / materials are not reused, recycled ‐ Ability to absorb variations in the nor valorised amounts of waste generated ‐ Large areas of land are required ‐ Possibility to reuse the land once the ‐ Transportation costs of the wastes to the landfill. landfill is definitively closed The volume should be minimal and should have a safe composition. Prior to landfilling, recycling and recovery processes must be carried out. Types of landfills. Different classifications according to: a) landfilling procedure: ‐ Trench: created by digging the ground ‐ Area or zone: it is not possible to dig the ground. Wastes are disposed in piles ‐ Depression: in a depression, natural or artificial. b) Degree of waste compaction: ‐ Low density: Density: 500‐600 kg / m3. Daily covering of waste ‐ Medium Density: Density: 750‐900 kg / m3. More frequent covering. Presence of insects, rodents, etc. ‐ High‐density: Density: 1100 kg / m3. Residues are compacted with heavy machinery. Waste is not covered. Presence of insects, rodents, odours, etc. Planning, design and operation of landfills: During the planning, locating and design stages the following issues must be taken into consideration to prevent them: ‐ Soil contamination. ‐ Groundwater and surface water pollution. Leachate collection must be ensured. It is achieved by a geological barrier and a waterproof lining underneath the waste piles. The thickness of this insulating layer in a MSW landfill can vary between 0.5 and 1 m (depends on the waste type). Reactions in landfills: Main components of the landfill: ‐ O2 diffused from the atmosphere ‐ H2O from waste and runoffs ‐ Microorganisms present in the waste ‐ Waste composition: mainly biodegradable O.M. and some inorganic compounds. Processes that can occur in the landfill depend on: nature of the residues internal moisture pH, temperature and pressure Biological and chemical activity are time dependent. Most important reactions: Biochemical and reduction‐oxidation (redox) Evolution of processes in a landfill: Leachates are produced by precipitation, runoff and uncontrolled decompostion processes of the O.M. from the wastes. Leachate composition changes over time, depending on the decomposition stage going on. Initially: aerobic fermentation processes. The temperature may indicate the activity of the waste in the landfill. pH can also be indicative of the overall status of the landfill (see next slide): Fresh or mature landfills depending on the aging status. Analysis of the leachate composition: Provides information about chemical and biological activities taking place. Evacuation of leachate: ‐ Recirculation to the landfill. ‐ Collected in a pond for further treatments as contaminated wastewaters. Biological processes: anaerobic fermentation (biogas). Transformation of O.M. into biogas (CH4, CO2 and others) and a solid fraction (called digestate) of poorer quality than compost. Other treatments: Thermochemical processes: Gasification and pyrolysis. Pyrolysis: Thermal decomposition of the O.M. in the absence or defect of O2. It is an endothermic process (450 ‐750 °C). Three product fractions are obtained: Gas: H2, CO, CH4, CO2 and light HC Liquid: known as pyrolysis oils, bio‐oils, tars… etc. Solid: charcoal. Gasification (see gasification of sewage sludge slides in Lecture 2): Decomposition of the solid matter into a combustible gas in an oxygen‐deficient atmosphere. Unit 3: Contamination of soils Lecture 9. Industrial Waste. 9.1 Definitions and classifications. 9.2 Hazardous Waste Management. 9.1 Definitions and classifications. From 1 June 2015, waste classification is based on: 1. Commission Decision of 18 December 2014, amending Decision 2000/532/EC on the list of waste pursuant to Directive 2008/98/EC of the European parliament and of the Council (2014/955/EEC). 2. Commission Regulation (EU) No 1357/2014 of 18 December 2014, replacing Annex III to Directive 2008/98/EC of the European Parliament and of the Council on waste and repealing certain Directives. This waste classification system applies across the EU and is the basis for all national and international waste reporting obligations. The List of Waste (LoW) (Appendix 1) The LoW provides a harmonised list for coding all wastes. The different types of waste in the list are fully defined by the six‐digit entry for the waste, including the respective two‐digit and four‐digit chapter headings. Waste can have one of the following three entry types: Non‐hazardous Hazardous (marked with an asterisk) Mirror (two different codes may apply simultaneously for a given type of waste –either hazardous or non‐hazardous. Without prejudice to the provisions of Article 7(3) of Directive 2008/98/EC, if a waste has a non‐hazardous entry, it is non‐hazardous without further assessment. If a waste has a hazardous entry, it is hazardous without further assessment. Industrial wastes are generated because of the industrial activity. Great diversity of processes and wastes. Different codes are assigned according to the type of waste and industrial sector and activity It is important to correctly identify and classify the types of wastes generated in a certain industrial activity A specific industrial waste category does not exist. Wastes generated in the industry must be classified into one of the following categories: ‐ Assimilable to urban waste: organic waste from food, paper packaging, etc. ‐ Inert waste: rubble, slag, ash, etc. The waste does not undergo any significant physical, chemical or biological transformations. ‐ Hazardous wastes: those included on the list of hazardous wastes → EU Framework Directive 2008/98/CE and Ley 22/2011 de Residuos y Suelos Contaminados (Annex III). Also, containers and packages that have contained them. Hazardous wastes can also be considered all those not included in the Ley 22/2011 and meeting certain conditions or reactivity characteristics: Explosives: substances which may explode under the effect of a flame. Oxidising: substances which react exothermically. Highly flammable: substances with flammable point below 21 °C or which can easily ignite on contact with air at room temperature. Flammable: substances with a flammable point ≥ 21 °C and < 55 °C Irritant: They can cause irritation upon contact with the skin or mucous membranes. Harmful: When inhaled or ingested, or if they penetrate the skin, they may cause limited health risks. Toxic: When inhaled or ingested, or if they penetrate the skin, they may cause serious risk to health, even death. Carcinogenic: they can cause cancer by inhalation, ingestion or skin penetration. Corrosive: substances that can destroy living tissues. Infectious: substances containing micro‐organisms that can cause diseases. Toxic for reproduction: They can produce non‐hereditary congenital malformations by inhalation, ingestion or skin penetration. Mutagenic: They may induce hereditary genetic defects if inhaled or ingested, or if they penetrate the skin. Other possible wastes that could be tagged as hazardous: Substances that release toxic gases in contact with air, water or acids. Substances that, after disposal, can yield another substance which could be classified as hazardous according to the different categories above listed. Dangerous for the environment: substances that may present immediate or delayed risks for the environment. 9.2 Hazardous Waste Management. The treatment given to a hazardous waste is not always the same. Circumstances and factors that can condition the type of treatment selected: Cost of the treatments. Existence of treatment centres and distance to them. Market price of materials recovered. Management costs of the materials obtained. Technological changes. Position of the administration regarding the different treatments. Sources of hazardous waste: ‐ Waste generated in the process of transformation. ‐ Products that have completed their life cycle. ‐ Waste from other wastes. Ranking of main industrial activities generating hazardous wastes: 1st Chemical industry (~30 %) 2nd Pulp and paper industry (~30 %) 3rd Metal transformations (~25%) 4th Basic metal industries (steelwork factories, foundries, etc.) (~10 %) 5th Textile industry (~3%) 6th Leather, shoemaking (< 3%) 7th Food, beverages and tobacco (< 3%) 8th Wood industry (< 3%) 9th Non‐metallic mineral products (< 3%) 10th Electronic and electric appliances (< 3%) Obligations of producers of hazardous waste: ‐ To separate and not mix various hazardous wastes, especially when their danger increases after being mixed. ‐ To package and label containers holding hazardous waste. ‐ To keep records of the hazardous wastes produced and their destination. ‐ To provide information to authorized management companies. ‐ To submit an annual report to the Administration stating: The amount of hazardous waste produced and imported, nature and destination. Any losses of wastes have to be reported too. Waste management includes the collection, storage, transportation, recovery and disposal of waste. Monitoring of storage sites is also included even after their definitive closure. Two types of actions can be implemented: ‐ Preventing the production of waste or minimizing it. ‐ Reducing the impact of waste generated, correcting their effect. Action Hierarchy: ‐ On‐site reduction of waste generation. ‐ Recovery of the resources contained in waste. ‐ Elimination or reduction of hazardous waste. ‐ Deposit or controlled storage. Minimization: Adoption of organizational and operational measures to decrease the quantity and hazardousness of products and contaminants generated. Key actions to achieve this: ‐ Prevention: avoiding generation and disposal. ‐ Source reduction: waste reduction through changes in the manufacturing process. ‐ Recycling: reuse and recycling of waste as raw material. ‐ Treatment: transforming waste into less hazardous substances. ‐ Landfilling: Controlled emissions. Lines of action minimization plan: ‐ Prevention techniques: Minimizing the amount and hazardous nature of waste at source. Using good operational practices (Best Available Technologies, BAT). Use of less polluting raw materials. Changes in the production process. Replacing or changing the final product. Flowchart of a facility for treating hazardous wastes: Factors to consider in the selection of a management system: ‐ Type of waste (properties and concentrations). ‐ Volume of waste. ‐ Possible recovery of materials or energy use. ‐ Cost of treatment. Basic systems used in the treatment of hazardous wastes: ‐ Incineration. ‐ Physical‐chemical treatments. ‐ Landfilling (safety deposits) Incineration: Organic pollutants are destroyed, the volume of waste is significantly reduced, and a less toxic or harmful residue is generated. Products of incineration: solid (slag and ashes), gaseous and liquid effluents: new waste streams are obtained. It is a final solution. Technology widely used for hazardous wastes in the 1970‐1980’s. Other related thermal processes include: vitrification (immobilization of inorganic residues) thermal desorption (removal of semi‐volatile or volatile constituents at temperatures lower than that of incineration). Using high temperatures requires the use of appropriate materials and, depending on the calorific value of the waste, to co‐feed an external auxiliary fuel. The waste composition is highly variable → an excess of oxygen (air) is required. This excess affects the temperature and composition of the combustion products: ↑ O2→ the combustion temperature decreases: affects the composition of the gaseous effluent emitted. wide range of contaminants are generated. Not all hazardous wastes are incinerated: Explosives, radioactive and non‐organic materials cannot be incinerated. Types of furnaces used in the waste incineration: ‐ Rotary ovens. They are the most versatile. All sorts of solid, liquid, sludge, etc. can be processed. Temperatures: 800‐1650 °C. ‐ Liquid injection chambers. Only for liquids with low viscosity. T: 1000‐1700 °C. ‐ Fluidised bed systems. Inert particles (sand, alumina, etc.) are used as coadjutant solid which reduces the combustion temperature (760‐870 °C). Pyrolytic furnaces. Two‐stage combustion: In the first step, the residue is treated in defect of air (50‐80% stoichiometric). Combustion products (HC) are burned in a second stage. ‐ Grate furnaces. They are used to treat wastes with high moisture content because of their thermal efficiency. Flows resulting from the incineration process: Solid waste from incineration ash Fixed ashes (bottom ash): SiO2 , Al2O3, MexSO4. (Me: metals) Fly ash: in the gaseous effluent. More reactive than bottom ash. Solids and sludge from the treatment of gaseous effluents. Wastewater: caused by the treatment of gaseous effluents. They are usually treated by evaporation or by physicochemical techniques. Gases: they have a very high content of solids and other species having different chemical nature (unburnt species, acid gases, etc.) Sources of contaminants in a waste incineration plant: Physicochemical treatments: ‐ Physical processes: These are basically operations for preparing the material. Their objective is to reduce the volume of waste: filtering and screening, sedimentation, flotation, centrifugation, etc. ‐ Chemical processes: they try to reduce the danger of the wastes or to make them inert by some chemical reaction: neutralization, oxidation, reduction, etc. Landfilling: The objective is to avoid as much as possible the use of this management system due to the associated problems of contamination of soils and groundwaters. But it is a mandatory step for the comprehensive treatment process of hazardous wastes. It is therefore the last stage of the management for this type of wastes. During the storage of wastes leachates and gas can be generated, it is necessary to have mechanisms for their proper collection and treatment (see previous Lecture 8). Area requirements for a hazardous wastes landfill: ‐ Absence of groundwater. ‐ Natural impermeability of the ground. ‐ Climatology: low rainfall rate, winds should disperse odors, and smells and particles must not reach population centers. ‐ Reduced impact on the landscape. ‐ Not close to population centers (over 2 km). ‐ Well connected to the industrial centers. ‐ Area having no records of flooding or earthquakes. Fundamental factor → impermeability: natural or through barriers. Thickness: the barriers must be at least: 5 m thick for hazardous wastes, 1 m for non‐hazardous (e.g. MSW) or inert wastes