The Biology of Biotech PDF
Document Details
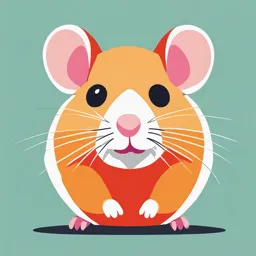
Uploaded by MagicBixbite
Tags
Related
- BIOL 150 Cell Biology 2024 Lecture Notes PDF
- NPTEL Biotechnology - Cell Biology - Module 1 - Lecture 1 PDF
- Techniques in Molecular Biology and Biotechnology Sept 1, 2021 PDF
- Cell Biology and Genetics Course - Biotechnology Certificate PDF
- Biology Study Guide PDF
- Principles of Biology - An Introduction to Biological Concepts PDF
Summary
This document provides a transcript of a course on the biology of biotechnology, covering the science behind biotech. It details the cell, DNA functions, and how mutations can lead to disease.
Full Transcript
Proprietary. Do Not Share. Transcript: The Biology of Biotech Section 1: The Cell Welcome to The Biology of Biotech: In this, we will discuss the science that drives biotech. This course will be broken into 3 sections. The first section will discuss the cell, the basic unit of life. The second sect...
Proprietary. Do Not Share. Transcript: The Biology of Biotech Section 1: The Cell Welcome to The Biology of Biotech: In this, we will discuss the science that drives biotech. This course will be broken into 3 sections. The first section will discuss the cell, the basic unit of life. The second section will discuss DNA, how the cell replicates DNA, and how the information stored in DNA is converted into proteins. Lastly, we will discuss how variation in DNA occurs, specifically through mutations, and how these mutations may lead to disease. Section 1 - The Cell Objectives In Section 1: • We will first define biotechnology. Setting the stage for why learning about the cell and its structure is important. • At the end of this section, you will be able to label all of the important structures of the cell, including the cell’s interior organelles. • Lastly, the unique functions of all of the organelles will be discussed. Biotechnology Is... Have you heard any of these buzzwords that deal with Biotechnology? Maybe you have heard about new treatment methods such as Gene Therapy or Immunotherapy. Maybe you have heard about the project to sequence the Human Genome, or maybe you have read about scientists cloning entire animals. Regardless of which of these terms you have heard or not heard, the world of Biotechnology is all around us and the possible treatment methods for diseases are getting more complex and sophisticated every day. Because of this, we created this Biotechnology course series to teach you the fundamentals of Biotechnology. It is by no means exhaustive, but it will start you on the road to understanding what medical breakthroughs could be possible in the future. All Biotechnology starts with cells, and that is where we will begin as well. Biology is The Basis of Biotech Biotechnology is the use of living systems, biological processes, or any component thereof, to make new products or technologies. This means that to understand biotechnology we must first understand the cell and how it functions. 1 Copyright 2023 Biotech Primer Inc. Proprietary. Do Not Share. Cells So, what are cells? Cells are defined as the basic unit of life, meaning that they are the smallest living things. Organisms can be made up of one cell, such as a bacteria or yeast cell, or can be composed of many types of cells that create many different types of tissues, such as a human body. No matter the complexity of the organism, the internal functions of the cell remain the same. We will next discuss what is inside the cell and what function each intracellular structure serves. The Cell: Organelles This will discuss the structure and function of all the different parts of the cell. First, we have the cell membrane that encloses all of the other components of the cell and protects them. The cell membrane is made up of molecules called lipids, specifically phospholipids. These membranes do not dissolve in water, which is very important because the body is mainly composed of water, and your cells must be able to keep excess water out. Next, we have the nucleus of the cell. This is where all the genetic information, DNA, is stored. The nucleus protects the DNA from the rest of the cell and is also the location where DNA is transcribed to messenger RNA (mRNA) so that proteins can then be made. We will discuss this process much more in section 2 of this course. On the outside of the nucleus, we find the nuclear membrane. This allows certain molecules to move in and out of the nucleus. This is also important in the production of proteins, as we will learn later. As mentioned, DNA is inside the nucleus and will be the focus of section 2 of this course. For now, it is enough to know that DNA stores all of our genetic information. The next cell structure we need to discuss is the Endoplasmic Reticulum, often called the ER. The ER processes proteins by cutting, folding, or adding necessary groups to their chemical structures, and is also the site of some of the next cell structures, ribosomes. Ribosomes are either located in the ER or move on their own through the cell. They are the protein-making factories of the cell that translate genes into proteins. The process whereby 2 Copyright 2023 Biotech Primer Inc. Proprietary. Do Not Share. these proteins are created in the ribosome is known as translation and will be discussed in detail in section 2. The next structure is the Golgi apparatus, which packages proteins that have been made by a ribosome and then transports these proteins to the cell's surface so that they can be released, or secreted, by the cell, and carry out their functions outside the cell. After the Golgi, we have the mitochondria. The mitochondria are where energy is produced inside the cell. The mitochondria are extremely important to cell function because cells take lots of energy to run smoothly. Energy is obtained from the food we eat by processing molecules such as sugar in the mitochondria. Mitochondria are especially important for cells that need a lot of energy such as cells in our heart or our brain. Consequently, these cell types have lots of mitochondria and the loss of some of these mitochondria can lead to diseases. Diseases of the nervous system such as Parkinson's disease and Alzheimer's disease, see the number of mitochondria in these cells decrease. Next, we have cytoskeletal components. Cytoskeletal components have two functions; they give structure to the cell. Believe it or not, each cell in your body has its little skeleton and that gives it a three-dimensional shape. In addition, these cytoskeletal components serve as transport pathways to move things around the inside of the cell. Lastly, we come back to the cell membrane, inside of which we find proteins known as receptors. The cells in your body do not act alone but rather in concert with one another. These receptors are key to communication between cells. And if we think about that, that's a pretty remarkable fact. Human beings have roughly 12 to 14 trillion cells as an adult. This number includes over two hundred different kinds of cells, and they all function together in your body for our entire lives. Part of what makes this possible are cell receptors. Receptors are a special class of proteins that receive information, this information is given to the receptor by a small molecule called a ligand. The ligand binds to the receptor, and they fit together like a lock and a key, with the ligand being the key and the receptor being the lock. Once the ligand has occupied the receptor, the receptor then sends a message through the membrane to the interior of the cell, which transmits the message to the nucleus. This message tells the DNA inside the nucleus which proteins to make. Once those proteins are made, the cell is able to respond in a manner appropriate to the signaling ligand. This process will be discussed in more detail in section 2, for now, we simply want you to know all of the structures of the cell and the basic functions they 3 Copyright 2023 Biotech Primer Inc. Proprietary. Do Not Share. perform. Take a bit of time to look at this finished diagram and take note of all the structures before jumping to the next screen. Additionally, if you would like more information on the complexity of cell signaling click the button on the right to look closer. The Complexity of Cell Signaling As can be seen on this screen, cellular signaling is a very complex network of cell surface receptors as well as internal cellular signaling molecules that transmit signals from different sources to the cell nucleus. While these signals involve hundreds or even thousands of different molecules, the end result is typically a signal that the cell receives to express a certain gene. In other words, to create the protein that corresponds to this gene. This is the first time we will mention increased gene expression, but don't worry if you don't fully understand because we will discuss the specifics of gene expression later in this course. For now, we will just bring to your attention two industry applications, the antagonist and the agonist. If you would like to learn these examples click the button to look closer, otherwise, click the back button to return to the main course. Industry Application: Antagonist The first example we will talk about is an antagonist. A well-known set of ligands that bind to cell receptors are known as growth factors. This group of ligands always signals the cell to grow and divide into more cells, a process called cell proliferation. While the cell proliferation process is certainly important to normal healthy cells, it is particularly relevant to cancer cells that grow, divide, and spread faster than normal cells. This brings us to antagonists. An antagonist is a molecule that binds to a cell surface receptor just like a growth factor would. However, when the antagonist binds to the receptor it actually blocks the signal of the receptor and therefore halts cell proliferation. As you might imagine this can be important in that it could make it possible to stop cancer cells from proliferating. An example of this will be discussed in section 3 of this course in more detail. For now, click on the look closer button to learn about the opposite of an antagonist, the agonist. Otherwise, click the back button to return to the main course. Industry Application: Agonist An agonist is a molecule that activates a receptor. For example, when insulin binds to its receptor on the surface of the liver, fat, or muscle cells, it triggers a series of chemical reactions 4 Copyright 2023 Biotech Primer Inc. Proprietary. Do Not Share. inside the cell. The end result of this is the movement of a second protein, the glucose transporter protein, to the cell surface. The glucose transporter protein now pumps glucose from the bloodstream into the insulin-sensitive cell where it is stored for later use. Type 1 diabetics do not produce insulin, and so must inject themselves with it in order to control their blood glucose levels. Basic Structures of the Cell – Knowledge Check Now that you have learned the organelles of the cell let's test your knowledge of these cell organelles. Match each of the organelles on the left side of the screen to their matching location on the diagram by dragging the organelle to one of the labels. Once you match all the organelles with their location in the diagram, click the checkmark in the lower right-hand corner to submit your answer. You will have 3 chances to match all the organelles correctly; after your 3rd attempt, the correct solutions will be shown. Section 1 - The Cell Summary Summarizing Section 1: • We first defined Biotechnology as the use of living systems, biological processes, or any component thereof, to make new products or technologies. • We then learned that cells are the heart of biotechnology as well as the basic unit of life and that cells have organelles that are critical to the functions that the cell must carry out to survive and replicate. • Lastly, we learned about each of the cell structures and organelles specifically, and how each of the organelles contributes to the cell's ability to grow, divide, manufacture proteins, and communicate. 5 Copyright 2023 Biotech Primer Inc. Proprietary. Do Not Share. Section 2: DNA & Proteins Now that we have completed Section 1 on the cell, we are now ready to discuss one of the most important molecules inside the cell, Deoxyribonucleic Acid or DNA. Section 2: DNA & Proteins Objectives In Section 2: • We will first explain the structure of Deoxyribonucleic Acid (DNA). • Then focus on how DNA functions inside the cell to store genetic information. • From there, we need to understand how the information contained in DNA is converted into a protein. • Lastly, we will describe how the shape of a protein determines the protein's function. So first, let's talk about the basics of DNA and its structure. All Cells Contain DNA With one notable exception, all cells contain DNA; from unicellular organisms such as bacteria and yeast to much more complicated multicellular organisms such as plants, to the exquisitely complicated organism, the human body. Because DNA is present in all cells but one, we know that DNA is very important to the function of a cell. To learn about the exception to the rule click on the look closer button, otherwise, click next to continue. Red Blood Cells The only cell that does not contain DNA is red blood cells. This lack of DNA is due to the specific function of red blood cells in transporting oxygen inside the human body. Oxygen is highly reactive, and it is not desirable to have DNA, the molecule that contains all the genetic information of the cell, next to highly reactive oxygen because bad things could happen. These negative effects will be discussed further in Section 3 when we talk about DNA mutations To return to the main course, click the back button on the right. Deoxyribonucleic Acid (DNA) One important thing that we're going to emphasize on the next screen is the fact that DNA is just a chemical. And amazingly, every living organism has the same chemical structure of DNA. Whether we talk about a lowly bacterium like E. coli, a plant such as a tulip, or a human being, 6 Copyright 2023 Biotech Primer Inc. Proprietary. Do Not Share. the chemistry of DNA is exactly the same in every one of these living organisms. The implication of that is that DNA from one organism can be read and understood by another organism. Deoxyribonucleic Acid Structure DNA is a polymer or a chain of interconnected building blocks. The building block of DNA is called a nucleotide. As a reminder, DNA stands for Deoxyribonucleic Acid. Let's break that name down. The first part of a DNA molecule is sugar and that sugar is called ribose. That's where the ribo comes from. The deoxy means without oxygen. And if you notice the sugar, there's an OH group here. Normally on the sugar ribose, there's another OH group, but in DNA, nature has removed the second OH group and just put hydrogen there, so we have deoxyribose, that's a ribose without one oxygen. Every single DNA molecule, no matter what cell it's in has this sugar, deoxyribose. In order to be able to locate different positions on the sugar, scientists assign a number to each relative position. You'll notice that the number is followed by an apostrophe. This is read as prime. So, the DNA has one prime, a two prime, a three prime, a four prime, and a five prime position. The most important positions to us are the three prime and the five prime positions because this is how one nucleotide connects to the next in a DNA molecule. We will see this on the next screen. The next component of a nucleotide is called the phosphate group. What you notice here is there's phosphorus in the middle of this group. Phosphorous is the energy atom inside a cell. Phosphates provide the energy to allow one nucleotide to connect to the next. This implies DNA is just a string of nucleotides, this is in fact the case and will be illustrated on the next screen. Therefore, to quickly summarize, we have sugar, deoxyribose, and phosphate. Every single nucleotide has these two components and they're exactly the same in every nucleotide. Every nucleotide whether it's in a bacteria cell, a plant cell, or an animal cell. So, what creates the differences between the DNA in each of these organisms? The differences are created by the component of DNA called the base. The base is where the information is contained in a DNA molecule. There are four bases. These bases are guanine, adenine, thymine, and cytosine. Therefore, when we think about the sequence of DNA, this is represented by the letters G, A, T, or C. Each letter specifies the chemical structure of the base, so in the structure of each nucleotide what changes is the base. 7 Copyright 2023 Biotech Primer Inc. Proprietary. Do Not Share. The deoxyribose and the phosphate are always present, but what makes one nucleotide different from another is which base is located or attached to the one prime position, whether it's a G, an A, a T, or a C. Putting The Nucleotides Together Next, we must discuss how these nucleotides are connected. As mentioned on the previous screen this first nucleotide contains the sugar with a connected phosphate group. The base attached to the one prime position of this nucleotide is the base thymine. Then, the first strand of DNA is built from the 3' end to the 5' end, adding cytosine, adenine, guanine, and so forth by a protein, specifically an enzyme, called DNA polymerase. So, as we can see we are building a strand from the 3' to the 5' end with the phosphate group as the intermediate between the nucleotides. Therefore, the backbone of the DNA consists of sugar-phosphate, sugarphosphate, and sugar-phosphate continually repeated. This backbone is identical for every nucleotide. As mentioned, before you can see what changes is the base attached to each nucleotide. You might be asking yourself, "How does this convert into information?" It turns out that it's the sequence of the bases that store the information in DNA. So, if we focus here on the bottom three nucleotides A, C, and T, we're going to pretend that this is a word in English. So, if we look at A, C, and T, it spells ACT. If we change the sequence, or in other words change the order of the letters and we make this C, A, T instead, that spells CAT. Two completely different things. So hopefully we're able to illustrate to you that it's the sequence, the order of the bases that define the information contained in the DNA. Lastly, we have to mention that DNA does not occur as a single strand, which is what we see here. DNA actually has a matching or complementary strand in which guanine always binds to cytosine, and thymine always matches with Adenine, that is G always matches with the C and the T always matches with an A. This is called base pairing, and T to A or G to C are called complementary base pairs. The implication of this is very profound because it means that if we know the sequence of one side of the DNA then we also know the sequence of the other side of the DNA molecule. Because the bases on each strand will always be complementary base pairs. Consequently, we only need the sequence of one side of the DNA to know the whole structure. 8 Copyright 2023 Biotech Primer Inc. Proprietary. Do Not Share. This is what Watson and Crick deduced in the 1950s. The fact that DNA is double-stranded, and it forms a helix and the idea of complementary base pairing that nature designed so that G's can only fit with C's, and T's can only fit with A's. And you might be asking what happens if we make a mistake, what happens if a T fits with a G? Well, the apparatus in your cells is in some cases able to detect and correct the mistake, however, if the mistake is not corrected we get what is called a mutation. Mutations will be discussed in more detail in Section 3 of this course. DNA Sequence So, as we discussed on the last screen when we talk about a DNA sequence. what we are talking about is the order of the bases attached to the nucleotides in the DNA molecule. The sequence of these bases will be important when we use this genetic information to create proteins. However, before we get to the creation of proteins, we need to discuss how the DNA is replicated inside the cell which we will discuss on the next screen. DNA Replication The first step in DNA replication is for the DNA molecule to be separated. Once this occurs there are now two single strands of DNA that will serve as a template for the construction of the new strands. After the two strands are separated, base pairs can be added to each strand. Remember that A always pairs with T, and G always pairs with C. The first few nucleotides on the end of the molecule will actually be added by an enzyme called RNA primase. These first few nucleotides serve as a primer for another enzyme called DNA polymerase. Once the primer is in place the DNA polymerase latches onto the strand that is being created. In this case, the molecules of DNA polymerase will add a G to the DNA strand on the left, and a T to the DNA strand on the right. The DNA polymerase then moves down the DNA strand, adding nucleotides as it goes. This process continues until two new double-stranded DNA molecules that are identical to the first DNA molecule have been created. It’s possible for errors to occur during DNA replication but many times the cell's built-in proofreading mechanisms will find and fix the mistake when these mistakes aren't corrected, they lead to mutations. Mutations will be discussed in section 3 of this course. The next few screens will discuss how DNA is packaged in the nucleus. However, before proceeding to the next screen if you would like to know how we replicate DNA in the biotech 9 Copyright 2023 Biotech Primer Inc. Proprietary. Do Not Share. industry click on the closer look button to learn about Polymerase Chain Reaction or PCR. Otherwise, click the next button to continue with the main course. Polymerase Chain Reaction (PCR) PCR is a laboratory technique that is used to make multiple copies of a part of the DNA sequence known as a gene. The process of PCR is carried out with a machine called a thermocycler, and to copy a gene we need to know the sequence of the gene of interest as well as have DNA primers for that sequence, an enzyme known as a DNA polymerase to build the gene, and lastly, of course, nucleotides which are the building blocks of DNA since to create many copies of the gene we will need these building blocks. In order to see how all of these components are used to perform PCR click on the look closer button to see a full video of the PCR process. Otherwise, click on the back button to return to the main course. DNA Tools – PCR A PCR reaction is set up to contain a small amount of sequence DNA. Two oligonucleotide primers, nucleotides, and the heat resistance DNA polymerase taq polymerase. The mixture is placed in a thermocycler, a machine that controls the temperature of the PCR reaction. The DNA is heated to 94 degrees Celsius causing the double-stranded DNA to separate. The reaction is then cooled to 42 degrees Celsius allowing the primers to hybridize into the complementary sequences in the DNA. The DNA present between the two primers is the only portion of DNA that will be amplified. This section of the DNA is called the Target. The action is heated to 72 degrees Celsius, the optimal temperature for taq polymerase to amplify the DNA. This completes cycle 1. Cycle 2 is the same process repeated. Double-stranded DNA is separated into two single strands. The primers hybridize taq polymerase amplifies DNA. During each subsequent cycle, the number of DNA copies grows exponentially until after 30 cycles over 1 billion copies of target DNA are made. DNA Replication DNA is packaged in structures called chromosomes. Humans have 23 pairs of chromosomes, each of which is composed of long strands of DNA that are wrapped around proteins called histones. This wrapping structure of DNA condenses the genetic information into a smaller package so that it can fit in the cell's nucleus. A good analogy for this would be a zip folder on 10 Copyright 2023 Biotech Primer Inc. Proprietary. Do Not Share. your computer. A zip folder packages files into a folder so that it can take up less space on your computer. Like your computer, a cell is able to extract all of the DNA out of the chromosomes when needed. Genome A complete set of all the chromosomes of an organism is known as the organism's genome. Each organism has a different number of chromosomes and therefore contains a different number of base pairs that make up different amounts of DNA. The Book of Life An analogy that we like to use for the genome is that of a book. If you imagine that the entire genome of an organism is a book then the bases in DNA are the letters of the book, the genes in DNA are the sentences of the book, and the chromosomes are the chapters of the book. And just like with a book, only after reading the whole book does it make sense. In other words, only by examining the entire genome of an organism will we be able to learn all about the genetic makeup of that organism. Comparative Genomics This screen shows the comparison of the number of chromosomes, bases, genes, and bases per gene for different organisms. Of specific note here is that all mammals from mice to humans have nearly the same number of bases and the same number of genes. Humans, as mentioned previously, have 46 chromosomes (23 pairs), approximately 3 billion bases, and 22,000 genes with 1 gene per 14,000 bases. Also of note is that a large portion (approximately 99%) of the human genome is identical to the chimpanzee genome. Now that we have learned about how genetic information is packaged in the human body, we can now move on to have this genetic information be used to create proteins that allow our body to function. DNA Contains Genes That Code for Proteins Now that we understand what a DNA sequence is, the next question is how is this DNA sequence used by the cell to create protein? How does the cell take the information in a base sequence and convert that information into a protein? 11 Copyright 2023 Biotech Primer Inc. Proprietary. Do Not Share. To answer this question, we will use an analogy. A specific sequence of DNA that is a specific order of the letters, G’s, A’s, T’s, and C’s, is called a gene. A gene is really like a recipe or a set of directions in order to make a protein. Therefore, your DNA is subdivided into several different genes. Human beings have roughly 22,000 different genes. To review, a gene is a specific sequence of DNA that contains the information or the directions to make a protein. A gene is not a protein; it's just the directions to make a protein. With so many different genes in the human genome, how does the cell know what genes to copy so that they can be made into proteins? Cells Express Genes Based on Signaling Your cells do not use all 22,000 genes at once. They select genes based on the information received from a ligand that connects to a receptor on the cell membrane. Cells create the proteins for the specific genes that are required based on the information it receives for external cell signaling. In this way, the genes that the cell is expressing can be changed quickly by simply changing the signals that the cell receives. This process is known as gene expression. Gene Expression Gene Expression is the process by which the instructions in DNA are converted into a functional product, such as a protein. Gene expression can be altered by cell signaling and involves the processes known as transcription and translation. These processes will be discussed in detail later in this section. First, let's look at the basic steps of gene expression. The Process of Gene Expression The general process of gene expression has 3 main steps: 1. A cell receives a signal when a ligand binds to a receptor on the cell surface. 2. The signal received by the cell then cascades through a network of signaling molecules that eventually take the signal to the nucleus where the cell "turns on" or expresses a specific gene based on the signal received. 3. The gene that was turned on is then used to create a protein in two steps called transcription and translation. This gives you the basics of how cell signaling leads to gene expression, the specific processes involved will be discussed in the next few Main Topics. 12 Copyright 2023 Biotech Primer Inc. Proprietary. Do Not Share. Gene Expression: Transcription Transcription is the first step in gene expression, it is the process of copying a specific gene into a corresponding piece of RNA known as a messenger RNA (or mRNA). Notice that RNA is a copy of a gene, but that it is single-stranded rather than double-stranded. This is because we only need one-half of the information to make a protein. The RNA is made in the nucleus of the cell, it is then shipped out and taken to the ribosome where the process of converting the mRNA to proteins, known as translation, occurs. Gene Expression: Translation The translation is the second step of gene expression. It is the process of reading the information contained in the RNA transcript and converting that information to a protein. Translation occurs inside the ribosome. The ribosome is kind of like the decoder ring which reads the RNA and assembles the protein based on the mRNA sequence. Cells Receive Signals & Make Proteins To summarize, the process of the cell creating proteins known as gene expression starts with an external signal that occurs when a ligand binds with a receptor on the cell's surface. Proteins Are Chains of Amino Acids Proteins are composed of amino acid chains. Amino acids are one of 20 different molecules that come together to form proteins. The sequence of amino acids of a protein determines each protein's structure and function. The chemical structure of amino acids is shown on the bottom left of the screen. All amino acids have an amino group and an acidic carboxyl group as shown. The "R" group is variable, with the specific R group determining the amino acid. Proteins Take Shape The different R groups on the amino acids have different chemical and physical characteristics – some are negatively charged, some are positively charged, and some are neutral. Some are relatively large, and some consist of only one atom and therefore do not take up much space. How these different R groups are ordered determines how they interact with each other, which in turn determines how the protein folds and therefore its ultimate structure. It is important to note here that small changes in this amino acid sequence can lead to profound changes in the 13 Copyright 2023 Biotech Primer Inc. Proprietary. Do Not Share. structure of the protein and therefore its ability to function. Examples of this will be discussed in Section 3. Fun Fact – Amino Acids As mentioned, there are 20 amino acids, 10 that are known as non-essential amino acids and 10 essential amino acids. An essential amino acid means that the amino acid cannot be created by the cell and thus must be provided to the cell through the organism's diet. One example of an essential amino acid is tryptophan. Tryptophan is found in turkey, thus leading to the urban legend that turkey makes you sleepy after Thanksgiving dinner. Tryptophan is converted in the body to sleep-promoting serotonin. However, truth be told turkey has about the same level of tryptophan as other meats such as chicken or beef. What is more likely the cause of your sleepiness is the large consumption of carbohydrates in the meal. Carbohydrate consumption also increases serotonin release in your body. Proteins Are Sensitive to Their Environment Proteins are exquisitely sensitive to their environment, even small changes to the environment can change the protein's shape. A great example of this is the hemoglobin protein. Hemoglobin is a protein in red blood cells that transports oxygen from the lungs to the tissues of the body and carbon dioxide from these tissues back to the lungs for exhalation. This process is controlled by slight changes in the pH that the cell encounters. A slightly higher pH in the lungs makes hemoglobin take on the conformation that makes it bind to oxygen. This oxygen is then released by hemoglobin when it encounters the slightly lower pH of the rest of the body. Protein structures can be complicated even further by post-translational modifications, which we will discuss on the next screen. Post-Translational Modifications Some proteins need to be modified with additional molecules to be fully functional. The most common examples of this are phosphorylation, or the addition of a phosphoryl group (shown on the left), and glycosylation, or the addition of a glycan group to a protein. The presence of these post-translational modifications will be important in Biotech 201 when we discuss the creation of recombinant proteins. Now that we have seen from a high level the entire process of creating proteins, let's take a closer look at how proteins are made inside the cell. 14 Copyright 2023 Biotech Primer Inc. Proprietary. Do Not Share. DNA to Protein This screen will review and walk through the creation of proteins at the molecular level. First, the DNA is unzipped. As it is unzipped an mRNA is assembled based on the activated gene in the DNA. This process is known as transcription. Next, the mRNA leaves the nucleus and enters the ribosome, the sight where the mRNA is translated into a protein. The ribosome pulls the mRNA through itself and as this occurs there are 3 base pair groups called codons. These codons correspond to different amino acids. Another type of RNA known as Transfer RNA (tRNA) binds to the amino acid on one end and an anticodon, the complementary base pairs for each codon, on its other end. In this way, the amino acids are put close together so that they can bind to each other so that as the RNA is pulled through the ribosome the chain of amino acids that will become the protein is formed. In order to see a video explaining the creation of proteins click on the look closer button to see a full video of the process, otherwise, click the forward button to continue the main course. Proteins – Protein Expression A gene is a segment of DNA with a specific sequence of nucleotides that contains the information for making a protein. The gene is bound on either end by two sides: the promoter site and the termination site. Transcription begins when an enzyme called RNA polymerase binds to the promoter site. As RNA polymerase moves along the DNA, the two strands are separated. It is important to note, that whenever a protein is being made, only the segment of DNA that encodes for that protein is separated. The entire length of DNA is not separated. Complementary RNA bases bind to the unpaired DNA bases on one of the exposed strands and are then attached to one another by RNA polymerase. These individual RNA bases are found floating freely in the cell. Recall uracil is the complementary nucleotide to Adenine in RNA. When the RNA polymerase reaches the termination site, the separated DNA strands bind to reform the double-Helix and the new RNA molecule moves away. This RNA is a copy, a transcript of the information contained in the gene. The machinery which reads the mRNA and synthesizes the protein is called the ribosomes. Ribosomes have two sub-units, one large and one small which assemble on the mRNA. The large subunit has two binding sites for another type of RNA called transfer RNA or tRNA. tRNa is the molecule responsible for leading a codon to its specific amino acid. tRNA has an amino 15 Copyright 2023 Biotech Primer Inc. Proprietary. Do Not Share. acid attached to one end which will be used in the assembly of the protein. The other tRNA end has a three-nucleotide sequence called the anti-codon which is complementary to the codon on mRNA. The ribosome assembles on the start codon of the mRNA A U G, tRNA with the anti-codon U A C, and amino acid methionine binds to the A U G codon. This tRNA is in the P site of the ribosome. The A site is open for the second tRNA whose anti-codon is complementary to the next mRNA codon. Once the second tRNA is in place, the first tRNA is an amino acid, Methionine binds to the second tRNA amino acid. The first tRNA then leaves the P site and the ribosome slides down the tRNA reposition to the second tRNA in the P site. You can see that this tRNA contains the growing polypeptide chain. A third complementary tRNA enters the now empty A site. The polypeptide chain on the P site tRNA is transferred to the amino acid in the A site. Again, the P site tRNA and the ribosome slide down the mRNA. This cycle continues as the ribosomes move down the mRNA. The amino acids are stitched together to create a polypeptide chain according to the order of the codons on the mRNA. When the ribosome reaches a stop codon, the release factor binds to the A site; it carries no amino acid. This release factor signals the ribosome to release the newly synthesized protein. The ribosome then disassembles from the mRNA and moves on to find another mRNA molecule. Messenger RNA (mRNA) There are a couple of differences between mRNA and DNA that should be discussed here. As mentioned previously RNA is single-stranded rather than double-stranded like DNA. Additionally, RNA does not contain the base Thymine (T). All T's in the RNA sequence are replaced by Uracil (U). As such the sequences of 3 base pairs in mRNA made up of G's, C's, A's, and U's is called a codon and each codon codes for a specific amino acid in the protein sequence. To look closer at how a codon codes for an amino acid click the button on the right. Otherwise, click next to continue. Codon Chart This screen shows the list of the 3 base codons and the amino acids for which they code. To use the table, we start with the first base pair of the codon, in this case, that first base pair is C, that takes us to the second row of the table. 16 Copyright 2023 Biotech Primer Inc. Proprietary. Do Not Share. We then look at the second base of the codon, in this case, an A, and that narrows it to the third column on the table. So we know that the amino acid is in the second row, the third column. Lastly, we take a look at the last base, in this case, another C. This lets us know that the codon CAC will code for the amino acid Histidine. It is also important to note that while some changes to the codon will change the amino acid, some mistakes, such as CAU instead of CAC in this case will still code for the correct amino acid. This redundancy of codons is one safeguard against protein mutations. What Are Proteins? So now that we know how proteins are made let's discuss what proteins do in the cell. There are several classes of proteins. One of the most important classes is enzymes. Enzymes catalyze chemical reactions. Cells perform thousands of chemical reactions every second. One key example of a chemical reaction that we have discussed here is copying DNA into RNA. This process is carried out by an enzyme called a polymerase. Enzymes also break down the food that you eat including meats, sugars, and lipids, and convert it into small molecules that can then be turned into energy or reassembled into other molecules in your cell – two processes that are also carried out by enzymes! Receptors are proteins generally embedded in the cell membrane that receive information from outside the cell and then relay that information inside the cell. Receptors receive this information by interacting with a signaling molecule known as a ligand. Examples of receptors are neuroreceptors and growth factor receptors. There are hundreds and hundreds of receptors. Structural proteins give shape and infrastructure to the cell. These are what make up the cytoskeleton. Two examples of structural proteins that you may be familiar with are actin and collagen. Lastly, antibodies are a very important protein not only in your body but also as a product of the biotech industry. Antibodies are proteins that recognize and bind to molecules extremely specific to each antibody called antigens. When the antibody binds to its antigen, whether it be another protein, a virus, or bacteria, it signals to the immune system that the antigen does not belong in the body and should be targeted for destruction. Antibodies are further discussed in the Online Biotech Primer Course on Immunology. 17 Copyright 2023 Biotech Primer Inc. Proprietary. Do Not Share. Section 2: DNA Summary In Section 2: • We explained that DNA is made of chemical building blocks called nucleotides. These nucleotides make up DNA in all organisms from bacteria to human beings. • Next, we introduced the idea of genes as parts of the DNA sequence that serve as recipes for cells to create proteins. The first step to the creation of proteins is to take a gene in the DNA sequence and transcribe it to a singlestranded RNA molecule known as a messenger RNA (mRNA). This process takes place inside the cell's nucleus. • From there, the mRNA leaves the nucleus and travels to the ribosome where it is translated into a protein. • Once we discussed how proteins are created, we then discussed a few different types of proteins and how the sequence of amino acids that make up a protein as well as the protein's shape directly correspond to the function of the protein. Even small changes in the environment the protein is in, or the sequence or shape of the protein can drastically affect its functionality of the protein. Next, we will move on to the last section of this course on Genetic Variation. 18 Copyright 2023 Biotech Primer Inc. Proprietary. Do Not Share. Section 3: Genetic Variation Now that we have discussed the genetic information of humans, DNA, in section 3 we will focus on how variations in DNA can occur, specifically through mutations, and how these mutations can lead to disease. Section 3: Genetic Variation Objectives In Section 3: • First, we will discuss DNA mutations, and what causes these mutations. • And ultimately how certain DNA mutations can lead to disease. • Finally, we will describe how differences in a person's DNA can influence the diagnosis and treatment of various diseases. Chromosomes & Genetic Variation Before discussing mutations, however, we must first discuss how genetic information is normally passed from one generation to the next. As discussed in the last section, humans have 23 chromosome pairs, or 46 chromosomes total in all of the cells in their body except for one cell type. This cell type is the reproductive cells - the egg for females and the sperm for males. These reproductive cells have 22 single chromosomes plus an X chromosome in the egg, and either an X or a Y chromosome in the sperm cell. When the egg and the sperm cells combine, a zygote is formed that will become a child. This zygote has the full complement of 23 pairs or 46 chromosomes in total. As we can see in the image, half of these chromosomes come from the mother, and half of these chromosomes come from the father. If an X chromosome is passed from the father, then the offspring will have 2 X chromosomes and be female, and if a Y is passed from the father, then the offspring will have an X and a Y and will be male. Now that we have seen how DNA is passed from one generation to the next, we can now begin to discuss how this process can sometimes lead to disease. Normal & Abnormal Chromosomes A person's chromosomes can be examined using a process known as karyotyping or determining a person's karyotype. A karyotype is simply a picture of the person's chromosomes. The chromosomes are isolated and stained with a dye so that they can be seen under a microscope. The chromosomes are then rearranged by chromosome size with the largest being chromosome 1 and the smallest being chromosome 22. 19 Copyright 2023 Biotech Primer Inc. Proprietary. Do Not Share. Lastly, the so-called sex chromosomes, the X and Y chromosomes are shown. Karyotyping can also be used to determine if a person has a genetic disease. In this case, this abnormal karyotype shows something called trisomy 21. This is where the person has the presence of 3 chromosome 21s instead of the usual matched pair of chromosomes. Trisomy 21 is also known as Down syndrome. DNA Determines Traits Each individual's DNA determines many traits. Traits are notable features or characteristics of an individual that are inherited from their parents. Traits that are controlled by DNA include physical characteristics such as ear shape, eye color, and hair color. These physical characteristics are likely the first thing that we think of when we think of hereditary traits, however, traits can control other things such as behavioral traits as well as predispositions to certain diseases. On the upcoming screens, we will discuss a few diseases that are tied to heredity. Alleles In order to understand how diseases can be passed from generation to generation it is important to understand alleles. Alleles are alternative forms of a gene located at a specific position on a chromosome. These DNA sequences determine the traits that are passed down from parent to offspring. A dominant allele is an allele that will produce the trait even if only one copy of the trait is present. An example of a dominant allele that causes a disease is Huntington's disease. If the dominant allele for this disease is passed along from either parent to the child, this is enough to cause the disease in the child. A recessive allele on the other hand is an allele in which both copies of the allele, one from each of the parents, must be recessive for the trait to be produced in the child. An example of this is cystic fibrosis, a disease in which both parents must pass along the recessive allele for the child to have the disease. The passing of alleles for disease from one generation to the next is one way that a person can contract a disease based on their genetic information. Another way for a person to develop a disease is from mistakes known as mutations that occur when copying DNA, we will discuss mutations next. Introducing Mutations 20 Copyright 2023 Biotech Primer Inc. Proprietary. Do Not Share. In section 2 of this course, we discussed how a DNA sequence eventually leads to proteins, we now need to discuss what happens when there is a mistake in this process. Remember that the codons are three base pairs grouped in a DNA sequence that codes for an amino acid in a protein. Therefore, if there is a change in the sequence of the DNA, the change in the codon sequence could potentially change the amino acid. Furthermore, since we know that the sequence of amino acids leads directly to the shape and therefore function of the protein, we know that changing an amino acid in a protein can alter the function of the protein and that change could lead to disease. This change in the sequence of the DNA that leads to errors in proteins is called a mutation, and there are several ways that mutations can arise. Causes Of Mutations One way that a mutation can arise is when we have replication errors, meaning an error that occurs when replicating DNA inside the cell. Your DNA is composed of 3 billion nucleotides, A's, G's, C's, and T's, and our cells take roughly 20 hours to divide. Therefore, given the sheer number of bases that need to be copied, cells occasionally make mistakes in the replicating process. The cell does have an editing system that can correct these errors, but not all errors are corrected. The types of replication errors will be discussed on the next screen. Another cause of the mutation is exposure to things in the environment such as radiation or certain types of chemicals. With radiation, the DNA simply gets exposed to energy and that energy can chemically alter the DNA, causing breaks in the DNA, and erroneous repair of this DNA. Therefore, radiation is another source of mutations. Some chemicals react with your DNA. Similar to radiation, they can cause damage to the DNA and that damage can sometimes be repaired correctly and sometimes not. For any one of these mechanisms, whether it's a replication error, exposure to radiation, or exposure to a chemical, the result is that the DNA sequence is altered. This is known as a mutation. The mutation to the DNA in turn alters the amino acid sequence of the protein and now the protein may not be able to function properly. The Nature of Mutation 21 Copyright 2023 Biotech Primer Inc. Proprietary. Do Not Share. On this screen, we can see examples of the different types of replication errors. We start with what we call the normal or wild-type sequence. This is the correct DNA sequence. The first error shown is known as a substitution error in which the cell in this case has added a C instead of a T. Therefore, instead of having the codon ATG, we have the codon ACG. And if we look in the codon table, the little decoder key, we could see that that may change what the amino acid is and that may change the function of the protein. This error is called a substitution error. Another type of error is deletions which are instances when the cell does not add a base that should be added to the sequence. In this example, we can see that the base T is missing from what is present in the Normal/Wild Type DNA. This type of error not only affects the codon where the error occurs but also changes all the codons past that error. As a result, this type of error in most cases will result in a non-functioning protein and can sometimes be fatal to the cell. Lastly, there are insertion errors when the cell adds bases to the sequence that should not be in the sequence. In this example, we can see that the cell has added two extra bases, a G and a C. We therefore now have an AGC codon instead of an ATG codon, and just like the deletion error every codon that follows this codon will be altered due to this error, and again this will in most cases lead to non-functioning proteins and/or cell death. With mutations, they can be deleterious, or have a negative effect, as we have discussed. That is the mutation leads to damaged gene function, incorrect protein creation, and therefore disease. However, not all mutations result in a negative effect. There are also a number of mutations that are neutral, meaning they do not affect the function of the cell. This could be because the change in the DNA sequence occurred somewhere in the DNA sequence where there is no gene. Therefore, if a mutation occurs in an area of the sequence that does not code for a protein the error is not propagated further and it does not affect the function of the cell. Lastly, some mutations can be adaptive, meaning they may lead to an advantage. For example, this could be a mutation that makes you more resistant to this year's flu virus. Genetic Variation Now that we understand what mutations are and the potential consequences, it is important to note that mutations also lead to what is called genetic variation, that is, how one person is slightly different from the next due to differences in their DNA sequence. And it turns out that through 22 Copyright 2023 Biotech Primer Inc. Proprietary. Do Not Share. sequencing multiple human beings' DNA, scientists have predicted that there is only a 0.1% difference in the DNA sequences from one person to the next. So that means that essentially, we are all 99.9% identical when it comes to our DNA. But that 0.1% difference is enough to make us different in appearance from one another. Most of the differences between individuals are what are called single nucleotide polymorphisms or SNPs (note to narrator SNP is pronounced SNIP). If we dissect the words in SNP; single means one, nucleotide means the molecules of DNA, in this case, the bases A, G, T, or C, and the polymorphism means many different shapes. If we put that in the context of the cell using DNA to make a protein, what happens here is we have a single change to a base in the DNA sequence, for instance, an A in place of a T or an A in place of a G, that changes the amino acid sequence of the protein. By changing the amino acid sequence in our protein, the shape of the protein can change, and that's what we call polymorphism. That is what is being illustrated on the right with the different mister potato heads. Each Mr. Potato Head could have slight differences in their DNA sequence that can lead to very recognizable changes in appearance. The changes in DNA can lead to differences such as eye color, hair color, and so forth. Additionally, from a healthcare perspective, these differences can be even more profound and even more important. Things like differences in your susceptibility to a certain disease, how you respond to environmental factors, or how you would respond to certain drug treatments or vaccines. Genetic Basis of Disease To illustrate more specifically how mutations can lead to disease. Let's discuss the genetic basis of disease. In other words, what diseases are inherited and passed down from one generation to the next? In classifying genetic diseases, we will look at two broad categories. The first are called monogenic diseases, where one gene causes the disease. And the second category is polygenic diseases where many genes contribute to the disease. An example of a monogenic disease is sickle cell anemia in which an A is substituted for a T in a specific genes sequence. We will discuss sickle cell anemia in more detail on the next screen. Another example is cystic fibrosis in which there aren't any substitutions but in this case a deletion of three bases. In this case, the A's, G's, and T's are deleted from a particular gene, 23 Copyright 2023 Biotech Primer Inc. Proprietary. Do Not Share. dramatically changing the shape of a specific protein in the lungs called the chloride ion channel. This change in protein shape stops the chloride ion channel from functioning properly, and the lungs begin to die. As the cells inside the lungs begin to die, they release DNA into the lungs, and this DNA clogs the lungs, making these patients prone to serious lung infections. A third example of a monogenic disease is Huntington's disease. This disease is caused by the insertion of extra bases into a particular gene. In this case, it is a very specific insertion of a trinucleotide repeat called the CAG repeat. This 3-base sequence repeats over and over, and if you have 30 or more CAGs in a row then you will absolutely get Huntington's disease and this disease is unfortunately always fatal. Though we know that the CAG repeat is the cause of the disease, we are just now beginning to understand how this repeated sequence affects the proteins in the cell and then how that ultimately leads to damage to the brain and ultimately death. Examples of polygenic diseases or diseases that are caused by more than one gene are cancer, heart disease, and Parkinson's Disease. The current belief is that several cancers are affected by 50 to 200 or more genes that have been mutated as cancer evolves. Therefore, the identification of these genes and targeting them for treatment is extremely difficult. This same issue applies to heart disease and the neurodegenerative disease Parkinson's disease as well. The complexity of cancer will be further explored later in this course. Although it is difficult to treat these diseases because knowing one gene that is mutated doesn't necessarily give us the whole answer, we can use that mutated gene as a predictor of the disease. These genes are known as susceptibility genes. These are certain genes that indicate your susceptibility or the likelihood that you will get a disease. Susceptibility genes have been shown to exist for heart disease for instance. The presence of these mutations makes the development of heart disease more likely, however, they do not guarantee that the person will develop the disease. Another example of a susceptibility gene is the BRCA gene for breast cancer. However, its mechanism of creating a higher risk of breast cancer is different. The BRCA gene codes for a DNA repair protein, a protein whose function is to repair proteins after mutations have occurred. Due to this protein's repair function, a mutation to this protein means it is more likely for more mutations to other proteins to occur and not be repaired. Thus, this increased risk of mutations also leads to an increased risk of cancer. 24 Copyright 2023 Biotech Primer Inc. Proprietary. Do Not Share. Another example of a susceptibility gene is APOE. The APOE gene is a gene that codes for a specific protein called apolipoprotein E. This protein combines with fats in the body to form molecules called lipoproteins. There are three major alleles of this gene known as the e2, e3, and e4 alleles. The most common of these alleles is the e3 allele which is found in more than half of the population. However, the e4 version of the gene has been shown to increase an individual's risk of developing late-onset Alzheimer's disease, and those who receive two copies of this version of the gene (one from each parent) are at even higher risk. It is not fully understood how this allele leads to an increased risk of Alzheimer’s. However, researchers have found that the allele is associated with increased protein clumps called amyloid plaques, a well-known physiological symptom of Alzheimer's disease. Again, it is important to note that like the BRCA gene, the presence of the e4 allele does not mean the person will develop Alzheimer's disease, it just means that they are at higher risk. Alzheimer's disease, like many types of cancers, is an extremely complicated disease that is affected by many genes as well as possibly lifestyle and environmental factors, none of which are currently fully understood. Monogenic Disease: Sickle Cell Anemia Let's expand a little bit on the idea of how a change in the DNA sequence can relate to a particular disease state. As mentioned, the classic example here is the monogenic disease called sickle cell anemia. In sickle cell anemia, a mutation in a single gene gives rise to the disease. This gene is the hemoglobin gene. The hemoglobin gene contains a sequence of nucleotides, A's, G's, T's, and C's, that contains the information to make the protein hemoglobin. On the top left we see part of the normal sequence for the hemoglobin protein. As we have learned this DNA sequence is transcribed into a messenger RNA which then is translated to the protein in the ribosome. We can see part of the normal sequence of the amino acids in the protein here as well. If we then look at the sequence that corresponds to a person with sickle cell anemia, you'll notice that we've changed a T to an A. This A is shown in red. This is a single nucleotide polymorphism, and by only changing this T to an A, the amino acid is changed from glutamate to valine. When valine is added to the amino acid's sequence in place of glutamate, it causes the protein hemoglobin to fold differently, altering the protein's shape. This means that all of the millions of hemoglobin protein molecules in the cell are misfolded and therefore have the wrong shape. Because of this incorrect shape, the proteins begin to clump together which in turn causes the entire cell to change shape leading to the characteristic sickle shape. 25 Copyright 2023 Biotech Primer Inc. Proprietary. Do Not Share. Because these cells no longer have the nice round spherical shape, as they begin to travel through your blood vessels they begin to catch on to things because they're not nice and round and they don't bounce off and roll away. This is particularly true at branch points of the blood vessels. Finally, the cells begin to crash into each other and can rupture, releasing the hemoglobin. Another key component of hemoglobin is the molecules of the metal iron. This release of iron into the bloodstream begins to damage other cells and leads to all the classic effects of sickle cell anemia. And unfortunately, even though we know exactly which gene is responsible for the disease, and even know which nucleotide has been changed, despite all of that knowledge, it's still difficult to create an effective therapeutic. As a monogenic disease, sickle cell anemia is a good candidate for gene therapy or genome editing treatments. Polygenic Disease: Cancer A more complex disease in terms of genetics is cancer. Cancer is a polygenic disease, and as cancer begins to develop multiple genes become altered or mutated. So what this screen shows is the evolution, in general