Engineering Materials Lecture Notes PDF
Document Details
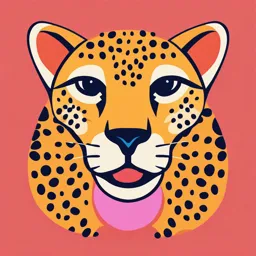
Uploaded by RomanticCedar
Central Philippine University
Tags
Summary
These lecture notes cover the chemistry of engineering materials, focusing on topics such as crystal structures and arrangements. Different types of crystal systems and structures are detailed, as well as important concepts like lattice parameters and unit cells. This document is a good resource for students studying engineering materials.
Full Transcript
THE CHEMISTRY OF ENGINEERING MATERIALS INTRODUCTION Important for our existence, day to day needs and even our survival Gold was the first metal used by mankind followed by copper Areas of science focusing on the study of materials: 1. Engineering Materials 2. Material Science 3. Materials En...
THE CHEMISTRY OF ENGINEERING MATERIALS INTRODUCTION Important for our existence, day to day needs and even our survival Gold was the first metal used by mankind followed by copper Areas of science focusing on the study of materials: 1. Engineering Materials 2. Material Science 3. Materials Engineering Atomic Arrangement of Crystalline and Amorphous Silicon Dioxide BASIC CONCEPTS OF CRYSTAL STRUCTURE BASIC CONCEPTS OF CRYSTAL STRUCTURE Lattice Point Properties of crystalline solids depend on the crystal structure of the material, or the manner in which atoms, ions, or molecules are spatially arranged Space Lattice or Lattice is a periodic arrangement of points in space with respect to three dimensional network of lines Lattice Lattice Point – each atom represented by a Space Representation of point, where the network lines intersect lattice point, array Lattice Array – arrangement of lattice points and space Lattice Space – the space covered by the lattice points BASIC CONCEPTS OF CRYSTAL STRUCTURE Unit Cell - Tiny blocks formed by arrangements of small groups of atoms Chosen to represent the symmetry of a crystal structure, and may be defined as: 1. Finite representation of infinite lattice 2. Small repeat entity 3. Basic structural unit Unit Cell Representation of 4. Building block of crystal structure unit cell BASIC CONCEPTS OF CRYSTAL STRUCTURE Lattice Parameters Six lattice parameters are: a, b, c, α, β, and γ. Typically in the order of few Angstroms (Å), equal to 0.1 nanometer Based on the length equality or inequality and the orientation (the angles between them, α, β, and γ) a total of 7 crystal systems can be defined Representation of dimensions of a unit cell BASIC TYPES OF CRYSTAL SYSTEMS BASIC TYPES OF CRYSTAL SYSTEMS BASIC CONCEPTS OF CRYSTAL STRUCTURE Bravais Lattices 14 types of crystal systems where presented, with the inclusion of the property of Centering Types of Centering 1. Face-Centered 2. Body-Centered 3. Base-Centered METALLIC CRYSTAL STRUCTURES Simple Cubic (SC) Most elementary crystal structure with three mutually perpendicular axes arbitrarily placed through one of the corners of a cell. Each corner is occupied with one atom Only polonium is packed in this manner Packing density is relatively low METALLIC CRYSTAL STRUCTURES Body-Centered Cubic (BCC) Atoms are arranged at the corners of the cube with another atom at the cube center. Has 2 atoms in one unit cell. Examples: alpha iron, chromium, tungsten, tantalum, and molybdenum Center and corner atoms touch one another along cube diagonals, and unit cell length a and atomic radius R are related through: METALLIC CRYSTAL STRUCTURES Face-Centered Cubic (FCC) Atoms are arranged at the corners and center of each cube face of the cell. Atoms are assumed to touch along face diagonals. Has 4 atoms in one unit cell. Examples: aluminum, copper, gold, lead, silver and nickel Spheres or ion cores touch one another across a face diagonal; the cube edge length a and the atomic radius R are related through: METALLIC CRYSTAL STRUCTURES Hexagonal Closed-Pack (HCP) Cell of an HCP lattice is visualized as a top and bottom plane of 7 atoms, forming a regular hexagon around a central atom. In between, these planes is a half-hexagon of 3 atoms. There are two lattice parameters in HCP, a and c, representing the basal and height parameters respectively. Has 6 atoms per unit cell. Examples: zinc, lithium, magnesium, beryllium Volume is computed as CRYSTALLOGRAPHIC DIRECTIONS Crystallographic Direction a line or vector directed between two points directions are given by specifying the coordinates (x, y, z) of a point on a vector (Pxyz) passing through the origin Notation: [xyz] CRYSTALLOGRAPHIC DIRECTIONS To determine a direction of a line in the crystal: 1. Find the coordinates of the two ends of the line and subtract the coordinates (Head – Tail) OR draw a line from the origin parallel to the line and find its projection lengths on x, y and z axis in terms of the unit vectors a, b and c. 2. Convert fractions, if any, in to integers and reduce to lowest term. 3. Enclose in square brackets [uvw] CRYSTALLOGRAPHIC DIRECTIONS Directions in Hexagonal Crystal If [XYZ] to [xyuz] Notation: [xyuz] x = (2X-Y)/3 Conversion from four notation to y = (2Y-X)/3 three notation: u = -(x+y) or If [xyuz] to [XYZ] u = -(X+Y)/3 X = (x-u) z=Z Y = (y-u) Z=z PROPERTIES OF CRYSTALS : ASSIGNMENT 1 Define the following crystal properties, and provide the equation for each, if there is any. Compute each property for SC, FCC, BCC and HCP. COORDINATION NUMBER ATOMIC PACKING FACTOR (APF) PLANAR DENSITY LINEAR DENSITY PROPERTIES OF CRYSTALS Coordination number is the number of nearest neighbor atoms or ions surrounding an atom or ion. For FCC and HCP systems, the coordination number is 12. For BCC, it’s 8. For SC, coordination number is 6. Atomic packing factor (APF) or packing efficiency indicates how closely atoms are packed in a unit cell and is given by the ratio of volume of atoms in the unit cell and volume of the unit cell. APF = "Volume of atoms" /"Volume of unit cell" SC = 0.52 or 52% FCC = HCP = 0.74 or 74% (26% void space in unit cell) BCC = 0.68 or 68% PROPERTIES OF CRYSTALS Planar density (PD) refers to density of atomic packing on a particular plane. Planar Density = PROPERTIES OF CRYSTALS LINEAR DENSITY Linear density (LD) is the number of atoms per unit length along a particular direction Linear Density = METALS INTRODUCTION employed for various engineering purposes and requirements iron is the most popular metal in the field of engineering ALL the metals have a crystalline structure ALLOY An alloy is a mixture or compound of two or more elements, at least one of which is metallic Alloying enhances some properties, as required by engineering applications, such as strength and hardness in comparison to pure metals Classified into solid solution and intermediate phase ALLOY Solid solution is an alloy in which one element is dissolved in another to form a single-phase structure In a solid solution, the solvent or base element is metallic, and the dissolved element can be either metallic or nonmetal Solid solutions can be Substitutional Solid Solution or Interstitial Solid Solution ALLOY Substitutional solid solution - atoms of solvent element are replaced in its unit cell by dissolved element. Interstitial solid solution - atoms of dissolving element fit into vacant spaces Substitutional solid solution (left) and between base metal atoms Interstitial solid solution (right) in the lattice structure. ALLOY Intermediate phases - There are usually limits to the solubility of one element in another. When the amount of the dissolving element in the alloy exceeds the solid solubility limit of the base metal, a second phase forms in the alloy. The term intermediate phase is used to describe it because its chemical composition is intermediate between the two pure elements. Its crystalline structure is also different from those of the pure metals. IMPORTANCE OF METALS High stiffness and strength – can be alloyed for high rigidity, strength, and hardness Toughness – capacity to absorb energy better than other classes of materials Good electrical conductivity – metals are conductors Good thermal conductivity – conduct heat better than ceramics or polymers Cost – the price of steel is very competitive with other engineering materials METALS USED IN MANUFACTURING PROCESS Cast Metal - starting form is a casting Wrought Metal - the metal has been worked or can be worked after casting Powdered Metal - starting form is very small powders for conversion into parts using powder metallurgy techniques CLASSIFICATION OF METALS FERROUS METALS These metals contain iron as main constituent Ferrous metals are classified as cast iron, wrought iron, and steel depending upon ingredients and percentage of carbon content NON-FERROUS METALS These metals practically do not contain iron FERROUS METALS Cast Iron (C.I.) Cast Iron contains a higher percentage of carbon ranging from 2 to 4.23 Cast iron is manufactured by melting of pig iron with coke and lime stone. The furnace used is known as CUPOLA. Properties of Cast Iron 1. It can be hardened by heating and sudden cooling, but it cannot be tempered. 2. It does not rust easily. 3. It is fusible. 4. It is hard and at the same time brittle. FERROUS METALS Cast Iron (C.I.) Classification 1. Grey Cast Iron - The carbon content is about 3% ad when fractured gives a grey appearance. It is soft and readily melts. Its strength is weak and is used for casting cylinders, pistons, manholes etc. 2. White Cast Iron - Its carbon content is 2.0 to 2.5%. It contains carbon in chemical form and on fracturing gives silver white luster. It is hard, not workable on machines and is used for preparing pump liners, drawing dies etc., FERROUS METALS Cast Iron (C.I.) Classification 3. Chilled Cast Iron - Its carbon content is 3.3%. It is produced by casting the molten metal against a metal chiller to obtain a surface of white cast iron. This is hard to a certain depth from the outer surface, which indicates the white iron. The inner portion of the body is made up of grey iron which is soft. It is used for manufacturing rail car wheels, dies, sprockets etc. FERROUS METALS Cast Iron (C.I.) 4. Malleable Cast Iron - Its carbon content is 2.3%. The composition of this is so adjusted that it becomes malleable. It is done by extracting a portion of carbon from cast iron. It has high field strength and used for manufacture of automobile and railway equipment such as rail cars, crank shafts gear boxes etc. 5. Toughened Cast Iron - It is obtained by melting cast-iron with wrought iron scrap. The proportion of wrought-iron scrap is about 1/4 to 1/7th of cast-iron. FERROUS METALS Wrought Iron It is an almost pure iron. Its carbon content is 0.15%. It is manufactured by the following stages: Refining Puddling Shingling Rolling FERROUS METALS Wrought Iron Properties 1. It is soft at white stage of heat. It can be easily forged and welded. 2. It is ductile, malleable and tough. 3. Its melting point is 1500°C. 4. It is resistant to corrosion. FERROUS METALS Steel Steel is defined as the iron alloy with a carbon content of up to 2.0%. Types of steel include low carbon steel or mild steel, medium carbon steel, and high carbon steel. FERROUS METALS Steel Carbon Properties Uses Content Low Carbon or 0.10 to 0.3% It can be easily hardened and Sheets, Tin Plates Mild tempered It is malleable and ductile It can be forged and welded It rusts easily Specific gravity is 7.8 Medium Carbon 0.3 to 0.6% These steels have high strength, Boiler plates, Railway toughness, hardness and stiffness tyres, pressing dies High Carbon 0.6 to 1.50% It can be easily hammered and Springs, Hammers, tempered Drills, Chisel It can be magnetize permanently It is granular in structure Specific gravity is 7.9 FERROUS METALS Alloy Steel Steel, to which elements other than carbon are added in sufficient quantity, in order to obtain special properties, is known as alloy steel. Examples of alloy steels include chromium steel, cobalt steel, manganese steel, tungsten steel, vanadium steel and Nickel steel. FERROUS METALS Alloy Steel Alloying elements Properties Uses added Chromium Chromium up to 0.9%, Highly ductile, can be Used for locomotive Steel Vanadium up to 0.15% easily worked and springs, pistons and welded bolts Cobalt Steel Cobalt is added to high Possesses magnetic Used for making carbon steel properties permanent magnet with strong magnetic field Manganese Manganese up to 1.90% It is hard, strong and Used for gears Steel ductile. It gives resistance to abrasion. Tungsten Tungsten content up to It is hard and maintains Used for lathe tools, drill Steel or High 7% cutting power at high cutters Speed Steel temperature Nickel Steel Nickel up to 3.5% It is hard and ductile Used for boiler plates, structural steel propeller shafts Vanadium Vanadium content up to Strong and ductile, Used for automobile NON-FERROUS METALS Aluminum It is a very light and useful non- Uses of Aluminum ferrous metal. It is produced mainly 1. For manufacturing of electrical from bauxite which is hydrated oxide conductors. of aluminum. 2. Making alloys. Properties of Aluminum 3. For manufacture of cooking utensils, 1. a tin white metal. surgical instruments, etc. 2. good conductor of heat and 4. For making parts of air crafts. electricity. 5. In manufacture of paints 3. malleable, ductile and very light. 4. highly electro positive element. NON-FERROUS METALS Copper Uses of Copper Copper is one of the most useful non- ferrous metals. It occurs in nature in a 1. For the manufacture of electrical free state as well as combined state. cables and wires and lightning conductors. Properties of Copper 2. For making alloys. 1. Bright shining metal of reddish color. 3. For house hold utensils. 2. high tensile strength. 4. For bolts and nuts. 3. very malleable and ductile. 5. For tubes, etc. 4. a good conductor of heat and electricity. 5. It melts at 1083°C NON-FERROUS METALS Tin Tin is a very important non-ferrous metal Uses of Tin which is obtained from ore, tin pyrites or 1. Used for plating. tinstone. 2. For lining and lead pipes. Properties of Tin 3. For making alloys and solders. 1. When a bar of tin is bent, a peculiar noise takes place which is known as a 4. For making trunks, boxes, cans, cry of tin. pans, etc. 2. white metal with brilliant luster. 3. soft and malleable. 4. withstands corrosion due to acids. 5. melts at 232°C. NON-FERROUS METALS Zinc Zinc is one of the most extensively used 4. can be rolled into sheets. non-ferrous metals. Occurs in wide 5. melting point is 420°C variety of combination in nature. Zinc is manufactured from ores by roasting and subsequent distillation with carbon. Uses of Zinc Properties of Zinc 1. Used for galvanizing steel sheets. 1. bluish white metal. 2. For making roofing sheets, pipes, 2. easily fused. ventilators. 3. brittle when cold, but malleable at a 3. Used in brass making. high temperature. 4. For making negative poles of batteries. NON-FERROUS METALS Lead Lead is extracted from galvena ores. It is Uses of Lead a very cheap, but useful nonferrous 1. For making shots and bullets. metal. It is produced from the ores by smelting in a reverberatory furnace. 2. Used for making gas pipes. Properties of Lead 3. Printers type letters. 1. very soft, heavy blush grey in color. 4. Flushing tank. 2. can be easily cut with a knife. 5. Roof covers. 3. makes impression on paper. 4. melting point is 327° and boiling point is 1600°C. SUPER ALLOYS Three Groups of Superalloys 1. Iron-based alloys - in some cases iron is less than 50% of total composition 2. Nickel-based alloys - better high temperature strength than alloy steels 3. Cobalt-based alloys - ~ 40% Co and ~ 20% chromium SUPER ALLOYS Importance: Room temperature strength properties are good in comparison to other metals, but not outstanding High temperature performance is excellent – tensile strength, hot hardness, creep resistance, and corrosion resistance at very elevated temperatures Operating temperatures often in the vicinity of 1100°C (2000°F) Have many applications Example is that it is used in systems in which operating efficiency increases with higher temperatures e.g., gas turbines, jet and rocket engines, steam turbines, and nuclear power plants SUPER ALLOYS Importance: Room temperature strength properties are good in comparison to other metals, but not outstanding High temperature performance is excellent – tensile strength, hot hardness, creep resistance, and corrosion resistance at very elevated temperatures Operating temperatures often in the vicinity of 1100°C (2000°F) Have many applications Example is that it is used in systems in which operating efficiency increases with higher temperatures e.g., gas turbines, jet and rocket engines, steam turbines, and nuclear power plants METAL PROCESSING processing of metals should be carried out carefully Affects the mechanical properties of metals Grain Size Effect Grains in metals tend to grow larger as the metal is heated metals with small grains are stronger but they are less ductile METAL PROCESSING Quenching and Hardening Most steels may be hardened by heating and cooling rapidly (quenching) metals are quenched in water or oil Produce very hard but brittle metal Annealing a softening process in which metals are heated and then allowed to cool slowly Tempering heating a hardened metal and allowing it to cool slowly produce a metal that is still hard and less brittle results formation of small Fe3C (iron carbide or cementite) precipitates in the steel, which provides strength METAL PROCESSING Cold Working refers to the process of strengthening a metal by changing its shape without the use of heat also known as plastic deformation or work hardening, involves strengthening a metal by changing its shape. the metal is subjected to mechanical stress so as to cause a permanent change to the metal's crystalline structure METAL PROCESSING Cold Working METAL MANUFACTURING: PRODUCTION CASTING Melting and molding - molten metal is poured into a mold cavity where, the metal take on the shape of the cavity once it cools For small intricate parts (Dies, jewelry, plaques, and machine components ) SHOULD NOT be used for products that require high strength, high ductility, or tight tolerances a. Expendable Mold Casting - the mold must be destroyed in order to remove the part b. Permanent Mold Casting - the mold is fabricated out of a ductile material and can be used repeatedly. METAL MANUFACTURING: PRODUCTION Powder Processing treats powdered metals with pressure (pressing) and heat (sintering) to form different shapes Powdered metallurgy (processing of powdered metals) is known for its precision and output quality – it keeps tight tolerances and often requires no secondary fabrications metal powder is compacted into the desired shape and heated to cause the particles to bond into a rigid mass. incredibly costly and generally only used for small, complex parts Powder processing is NOT appropriate for high-strength applications. METAL MANUFACTURING: PRODUCTION Powder Processing treats powdered metals with pressure (pressing) and heat (sintering) to form different shapes Powdered metallurgy (processing of powdered metals) is known for its precision and output quality – it keeps tight tolerances and often requires no secondary fabrications metal powder is compacted into the desired shape and heated to cause the particles to bond into a rigid mass. incredibly costly and generally only used for small, complex parts Powder processing is NOT appropriate for high-strength applications. METAL MANUFACTURING: PRODUCTION Forming raw metal (usually in sheet metal form) is mechanically manipulated into a desired shape Unlike casting, metal forming allows for higher strength, ductility, and workability for additional fabrications METAL MANUFACTURING: FABRICATION Deformation bending, rolling, forging, and drawing Deformation processes include metal forming and sheet metalworking Application of stresses to the piece which exceed the yield stress of the metal There are two types of deformation processes: a. Bulk Processes - characterized by large deformations and shape changes surface area to volume ratio is relatively small include rolling, forging, extrusion and wire and bar drawing. b. Sheet Metalworking - performed on metal sheets, strips and coils having a high surface area to volume ratio. use a punch and die to form the workpiece Bending, drawing and shearing METAL MANUFACTURING: FABRICATION Machining any fabrication method that removes a section of the metal also known as material removal processing Cutting, shearing, punching, and stamping are all common types of machining fabrication. a. Machining Operations - These are cutting operations using cutting tools that are harder than the metal of the product. They include turning, drilling, milling, shaping, planning, broaching and sawing. b. Abrasive Machining - In these methods material is removed by abrasive particles that normally form a bonded wheel. Grinding, honing and lapping are included in this category. c. Nontraditional Processes - These methods use lasers, electron beams, chemical erosion, electric discharge and electrochemical energy instead of traditional cutting and grinding tools When planning for machining in your supply chain, hardening processes should happen AFTER machining processes. Hardened metals have a high shear strength and are more difficult to cut. METAL MANUFACTURING: FABRICATION Joining Joining, or assembly, is one of the last steps of the metal manufacturing process includes welding, brazing, bolting, and adhesives. Assembly can be done by machine or by hand, where multiple parts are connected either permanently or semi-permanently to form a new entity. Finishing includes everything from galvanization to powder coating, and can take place throughout the manufacturing process MECHANICAL PROPERTIES OF MATERIALS Strength It is the capacity of the material to withstand the breaking, bowing, or deforming under the action of mechanical loads on it. Elasticity It is the property of a material to come back to its original size and shape even after the load stops acting on it. Plasticity It is the property of a material that makes it to be in the deformed size and shape even after the load stops acting on it. Ductility It is the property of a material that allows it to deform or make into thin wires under the action of tensile loads plastically. Tensile strength It is the property of a material that allows it to deform under tensile loading without breaking under the action of a load STRENGTH OF MATERIALS STRESS NORMAL STRESS the strength of a material per unit either tensile stress or compressive area or unit strength stress the force on a member divided by Tension or tensile force applied results area, which carries the force to Tensile Stress (increase in length) maximum stress in tension or Compression or compressive force compression occurring over a section applied results to Compressive Stress normal or perpEndiculat to the load (decrease in length) expressed in psi, N/mm2 or Mpa STRENGTH OF MATERIALS SHEARING STRESS BEARING STRESS results from forces applied parallel to the contact pressure between the separate area of the resisting force bodies also known as tangential stress differs from compressive stress, as it is an internal stress caused by compressive forces STRENGTH OF MATERIALS SHEARING STRESS BEARING STRESS results from forces applied parallel to the contact pressure between the separate area of the resisting force bodies also known as tangential stress differs from compressive stress, as it is an internal stress caused by compressive forces STRENGTH OF MATERIALS SIMPLE STRAIN Also known as unit deformation the ratio of the change in length caused by the applied force, to the original length Dimensionless STRENGTH OF MATERIALS STRESS-STRAIN DIAGRAM the graph of quantities with the stress σ along the y-axis and the strain ε differs in form for various materials Metallic engineering materials are classified as either ductile or brittle Ductile material is one having relatively large tensile strains up to the point of rupture like structural steel and aluminum Brittle materials have a relatively small strain up to the point of rupture like cast iron and concrete. STRENGTH OF MATERIALS STRESS-STRAIN DIAGRAM Proportional Limit (Hooke's Law) “within the proportional limit, the stress is directly proportional to strain” The constant of proportionality k is called the Modulus of Elasticity E or Young's Modulus and is equal to the slope of the stress-strain diagram from O to P. Then, STRENGTH OF MATERIALS STRESS-STRAIN DIAGRAM Elastic Limit (E) limit beyond which the material will no longer go back to its original shape when the load is removed Elastic and Plastic Ranges The region in stress-strain diagram from O to P is called the elastic range. The region from P to R is called the plastic range Yield Point Yield point is the point at which the material will have an appreciable elongation or yielding without any increase in load STRENGTH OF MATERIALS STRESS-STRAIN DIAGRAM Ultimate Strength The maximum ordinate in the stress- strain diagram is the ultimate strength or tensile strength Rapture Strength Rapture strength is the strength of the material at rupture. This is also known as the breaking strength POLYMERS POLYMERS Polymers are engineering materials of very high molecular weight Created by joining together single units called monomer to make a single large monomer molecule One of the common examples of polymers is polyethylene, composed of repeating ethylene molecules (C2H4) extensively used in food packaging, clothing, home furnishing, transportation, medical devices, information technology, and other multifarious applications Natural polymers: silk, wool and cotton POLYMERS Polymerization is a chemical reaction in which monomer units combine to form larger molecules that contain repeating structural units Proper polymer names are derived from the individual structural unit. For example: Ethylene polymerizes to polyethylene Propylene polymerizes to polypropylene Styrene polymerizes to polystyrene Vinyl Chloride polymerizes to polyvinyl chloride Types of Polymerization a. Chain-reaction Polymerization or Addition Polymerization b. Step-Reaction Polymerization or Condensation Polymerization POLYMERS Chain-reaction Polymerization or Addition Polymerization In this reaction, monomers simply add to one another to form the polymer. Examples of this are LDPE, HDPE, PVC, PP, and PS POLYMERS Step-Reaction Polymerization or Condensation Polymerization Two monomers add in a condensation reaction under the release of water or another small molecule like hydrogen chloride Examples of this are PET, PU and PA POLYMERS Polymer Branching Polymer may have similar molecular weights, but can have different properties, which mainly depends on the degree of branching Branching can be distinguished by density of the polymer High-density polyethylene (HDPE) is resulted when highly linear polyethylene molecules can be closely packed Low-density polyethylene (LDPE) are highly branched molecular chains which cannot be packed closely together Linear-low density polyethylene (LLDPE) which has short controlled side branches POLYMERS Polymer Linking Homopolymer made by linking only one type of small molecule or monomer Copolymer two different types of monomers are joined in the same polymer chain Terpolymer Three different types of polymer are joined in one polymer chain Purpose of copolymerization: combine properties of different monomers to enhance and improve the property of the final product or material POLYMERS Polymer Linking Terpolymer Homopolymer Copolymer POLYMERS Polarity and Material Properties Polarity is the way in which atoms bond with each other. one of the atoms exerts a stronger attractive force on the electrons in the bond, resulting to unequal sharing of electrons A polymer is polar if it has positive and negative poles. If non, then it is nonpolar. Polar Materials Nonpolar Materials POLYMERS Polarity and Material Properties Factors Affecting Polymer Polarity influences material properties Properties such as: 1. Elemental Composition 1. Melting point 2. Polarity 2. Solubility 3. Size or Molecular Weight 3. Barrier 4. Shape of Molecule 4. Coefficient of Friction of Stickiness 5. Thermal History 6. Mechanical History POLYMERS MOLECULAR STRUCTURE AND PROPERTIES 1. Crystallinity Highly ordered molecular arrangements -“crystalline” Completely random arrangements - “amorphous A polymer with lower crystallinity will have better clarity 2. Molecular Weight Distribution Polymers don’t have a specific molecular weight The greater the molecular weight distribution, the easier to melt the plastic. 3. Viscoelasticity materials exhibiting both viscous and elastic characteristics also known as anelasticity, which is present in systems when undergoing deformation. Accordingly, rapid application of a load to plastic will cause it to bend, and will return to its original shape once the load is released. NANOMATERIALS NANOMATERIALS Nanotechnology is science, engineering, and technology conducted at the nanoscale, which is about 1 to 100 nanometers. Nanomaterials are nano-scale particles, tubes, rods, or fibres, used in healthcare, electronics, cosmetics, textiles, information technology and environmental protection Concept: “There’s Plenty of Room at the Bottom” by physicist Richard Feynman at an American Physical Society meeting at the California Institute of Technology (CalTech) on December 29, 1959 NANOMATERIALS PROPERTIES OF NANOMATERIALS 2. Chemical properties 1. Physical Properties Molecular structure Their size, shape, specific surface area, and Composition, including purity, and known ratio of width and height impurities or additives Whether they stick together Whether it is held in a solid, liquid or gas Size distribution Surface chemistry How smooth or bumpy their surface is Attraction to water molecules or oils and Structure, including crystal structure and any fats crystal defects How well they dissolve NANOMATERIALS HOW TO MANUFACTURE MATERIALS 1. Top-down - reduces large pieces of materials all the way down to the nanoscale, like someone carving a model airplane out of a block of wood. This approach requires larger amounts of materials and can lead to waste if excess material is discarded. 2. Bottom-up - creates products by building them up from atomic- and molecular-scale components, which can be time-consuming. NANOMATERIALS HOW TO MANUFACTURE MATERIALS: NEW PROCESSES Chemical vapor deposition - a process in which chemicals react to produce very pure, high-performance films Molecular beam epitaxy - one method for depositing highly controlled thin films Atomic layer epitaxy - a process for depositing one-atom-thick layers on a surface Dip pen lithography - a process in which the tip of an atomic force microscope is "dipped" into a chemical fluid and then used to "write" on a surface, like an old fashioned ink pen onto paper Nanoimprint lithography - a process for creating nanoscale features by "stamping" or "printing" them onto a surface Roll-to-roll processing - a high-volume process to produce nanoscale devices on a roll of ultrathin plastic or metal Self-assembly - describes the process in which a group of components come together to form an ordered structure without outside direction NANOMATERIALS POTENTIAL EFFECTS OF NANOMATERIALS In health. There is experimental evidence of a range of possible interactions with biological systems and health effects of manufactured nanoparticles. In experimental systems in the laboratory they can affect the formation of the fibrous protein tangles which are similar to those seen in some diseases, including brain diseases. Airborne particles might cause effects in the lungs but also on the heart and blood circulation similar to those already known for particulate air pollution. There is some evidence that nanoparticles might lead to genetic damage, either directly or by causing inflammation. In environment. Wider use of nanomaterials will lead to increases in environmental exposure. Since they are minute, little is known about how they may then behave in air, water or soil. As a result of their diversity, nanomaterials may have a wide range of effects. Some kill bacteria or viruses. Experiments so far have also shown possible harmful effects on invertebrates and fish, including