Foundations of Embryology and Early Development PDF
Document Details
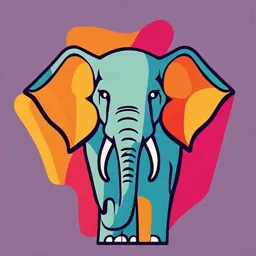
Uploaded by GratefulHyperbolic
University of Arizona
James Proffitt
Tags
Summary
Lecture notes and learning objectives for a course on embryology. The document covers topics such as directional terminology, spatial relationships, apoptosis, growth factors, and the general timeline of human development. It also details the clinical relevance of various factors in development.
Full Transcript
FOUNDATIONS OF EMBRYOLOGY AND EARLY DEVELOPMENT Block: Foundations Block Director: James Proffitt, PhD Session Date: Monday, August 05, 2024 Time: 1:00 – 2:30 pm Instructor: James Proffitt, PhD Department: Cellular & Molecular Medic...
FOUNDATIONS OF EMBRYOLOGY AND EARLY DEVELOPMENT Block: Foundations Block Director: James Proffitt, PhD Session Date: Monday, August 05, 2024 Time: 1:00 – 2:30 pm Instructor: James Proffitt, PhD Department: Cellular & Molecular Medicine Email: [email protected] INSTRUCTIONAL METHODS Primary Method: IM13: Lecture ☐ Flipped Session ☐ Clinical Correlation Resource Types: RE18: Written or Visual Media (or Digital Equivalent) INSTRUCTIONS Please read lecture objectives and notes prior to attending session. READINGS Read lecture notes prior to session. LEARNING OBJECTIVES 1. Relate general anatomy of an embryo to postnatal anatomy using correct directional terminology. 2. Describe the spatial and functional relationships between the embryo, extraembryonic tissue, and maternal tissue. 3. Describe the relevance of apoptosis, growth factors, transcription factors, and induction to normal & pathological development. 4. Define which germ layer(s) contribute to formation of a given organ 5. Describe the general timeline of human development. 6. Describe the clinical relevance of mutations, numeric/structural chromosomal abnormalities, critical periods, teratogen exposure, congenital infection, and multifactorial inheritance. 7. Apply the following terms for developmental injury: malformation, disruption, deformation, aplasia, hypoplasia, dysplasia, field defect, syndrome, sequence, association. 8. Describe the multiplication and distribution of cells in the embryo from zygote to blastocyst. 9. Compare and contrast the development of single zygotes, dizygotic twins, and monozygotic twins. 10. Describe the movement of the embryo from fertilization to implantation, including normal and pathological implantation locations. 11. Describe the development of the 2-layered embryonic disc and its spatial relationships with the amnion, primary yolk sac, Cyto-trophoblast, and syncytio-trophoblast. Block: Foundations | PROFFITT [1 of 44] FOUNDATIONS OF EMBRYOLOGY AND EARLY DEVELOPMENT 12. Describe the function of the primitive streak and its significance to sacrococcygeal teratomas and laterality defects. 13. Describe the movement of cell populations to produce the three germ layers of the embryo (ectoderm, mesoderm, endoderm). 14. Describe the folding of the embryo to produce the neural tube and body wall, including most common points of failure. CURRICULAR CONNECTIONS Below are the competencies, educational program objectives (EPOs), course objectives, session learning objectives, disciplines and threads that most accurately describe the connection of this session to the curriculum. Related Related Competency\EPO Disciplines Threads COs LOs CO-01 LO-01 MK-02: The normal Embryology H & I: Human structure and function of Development/Lifecycle the body as a whole and of each of the major organ systems CO-01 LO-02 MK-02: The normal Embryology H & I: Human structure and function of Development/Lifecycle the body as a whole and of each of the major organ systems CO-01 LO-03 MK-02: The normal Embryology H & I: Human structure and function of Development/Lifecycle the body as a whole and of each of the major organ systems CO-02 LO-04 MK-02: The normal Embryology H & I: Human structure and function of Development/Lifecycle the body as a whole and of each of the major organ systems CO-02 LO-05 MK-02: The normal Embryology H & I: Human structure and function of Development/Lifecycle the body as a whole and of each of the major organ systems CO-01 LO-06 MK-05: The altered Embryology H & I: Human structure and function Development/Lifecycle (pathology & pathophysiology) of the body/organs in disease CO-02 LO-07 MK-05: The altered Embryology H & I: Human structure and function Development/Lifecycle (pathology & pathophysiology) of the body/organs in disease CO-01 LO-08 MK-02: The normal Embryology H & I: Human structure and function of Development/Lifecycle Block: Foundations | PROFFITT [2 of 44] FOUNDATIONS OF EMBRYOLOGY AND EARLY DEVELOPMENT Related Related Competency\EPO Disciplines Threads COs LOs the body as a whole and of each of the major organ systems CO-01 LO-09 MK-02: The normal Embryology H & I: Human structure and function of Development/Lifecycle the body as a whole and of each of the major organ systems CO-01 LO-10 MK-02: The normal Embryology H & I: Human structure and function of Development/Lifecycle the body as a whole and of each of the major organ systems CO-01 LO-11 MK-02: The normal Embryology H & I: Human structure and function of Development/Lifecycle the body as a whole and of each of the major organ systems CO-01 LO-12 MK-02: The normal Embryology H & I: Human structure and function of Development/Lifecycle the body as a whole and of each of the major organ systems CO-01 LO-13 MK-02: The normal Embryology H & I: Human structure and function of Development/Lifecycle the body as a whole and of each of the major organ systems CO-01 LO-14 MK-02: The normal Embryology H & I: Human structure and function of Development/Lifecycle the body as a whole and of each of the major organ systems NOTES INTRO All of my lecture notes will contain: (1) a Key Terms list (bolded in the written lecture notes) that focuses on the most important basic vocabulary you will need to understand apply the concepts learned in this lesson (2) a curated list of helpful online resources (3) written lecture notes explaining the major concepts. Focus on applying your knowledge to clinical scenarios presented in lesson materials, ScholarRX, your USMLE practice books, or develop some on your own with friends with tools like UWorld or Anki. Additional citations are included at the end of the notes. Key Terms: Directional Terms & Anatomical Planes Cranial Superior Block: Foundations | PROFFITT [3 of 44] FOUNDATIONS OF EMBRYOLOGY AND EARLY DEVELOPMENT Caudal Inferior Ventral Anterior Dorsal Posterior Proximal Distal Lateral Medial Superficial Deep Processes Organogenesis Signaling Morphogenesis Induction Apoptosis Fertilization Dizygotic twinning Monozygotic twinning Implantation Gastrulation Neurulation Body Folding Anatomical Structures Embryo Head Brain Optic/Otic placodes Nasal Pit Pharynx Gut tube Cloaca Pharyngeal arches Limb Bud Body Wall Somites Body cavity (Coelom) Heart/Heart tube Amnion Secondary yolk sac Connecting stalk Allantois Chorion Placenta Block: Foundations | PROFFITT [4 of 44] FOUNDATIONS OF EMBRYOLOGY AND EARLY DEVELOPMENT Germ layers Ectoderm Surface ectoderm Neural plate ectoderm Neural crest cells Mesoderm Paraxial mesoderm Intermediate mesoderm Lateral plate mesoderm (Somatic & Visceral) Endoderm Epithelium Mesenchyme Zygote Inner Cell Mass (embryoblast) Outer Cell Mass (trophoblast) Blastocoel Blastocyst Epiblast Hypoblast Embryonic Disc Amniotic Cavity Amniotic Membrane Amnion Yolk Sac (primary and secondary). Cyto-trophoblast Syncytio-trophoblast Primitive streak Node Primitive groove Notochord Molecules & Genes Growth factor Transcription factor Regulatory genes Hox genes Sonic hedgehog (Shh) Wnt family genes FGF family genes Hormones Human chorionic gonadotropin (hCG) Clinical Terms Embryonic period Fetal period Mutation Chromosomal number aberration Block: Foundations | PROFFITT [5 of 44] FOUNDATIONS OF EMBRYOLOGY AND EARLY DEVELOPMENT Chromosomal structure aberration Environmental factors Multifactorial inheritance Critical period Teratogen Congenital infection Malformation Disruption Deformation Aplasia Hypoplasia Dysplasia Field defect Syndrome Sequence Association Pierre-Robin Sequence DiGeorge Syndrome Potter Sequence VACTERL Association Ectodermal Dysplasia Microcephaly Treacher-Collins Volvulus Holoprosencephaly Conjoined Twins Twin-twin Transfusion Syndrome Ectopic Pregnancy Situs inversus Sacrococcygeal teratoma Spina Bifida Ectopia Cordis Gastroschisis Omphalocele Online Resources Basic overview of first 3 weeks: https://www.youtube.com/watch?v=-rwqg58sxWU 3D PDFs of embryos (download to view 3D; requires up-to-date Adobe Acrobat Reader) from am 3D embryology 15–17 day Embryo: http://3datlas.3dembryo.nl/3DAtlas_CS07-8752-v2016-03.pdf 17–19 day Embryo: http://3datlas.3dembryo.nl/3DAtlas_CS08-8671-v2016-03.pdf 19–21 day Embryo: http://3datlas.3dembryo.nl/3DAtlas_CS09-H712-v2016-03.pdf 21–23 day Embryo: http://3datlas.3dembryo.nl/3DAtlas_CS10-6330-v2016-03.pdf 23-26 day Embryo: http://3datlas.3dembryo.nl/3DAtlas_CS11-6784-v2016-03.pdf 26-30 day Embryo: http://3datlas.3dembryo.nl/3DAtlas_CS12-8505A-v2016-03.pdf Block: Foundations | PROFFITT [6 of 44] FOUNDATIONS OF EMBRYOLOGY AND EARLY DEVELOPMENT Origami Embryo: https://origamiembryo.cba.arizona.edu/ An online database of known correlations between genetic mutations and congenital defects, the Online Medelian Inheritance in Man database: http://www-ncbi-nlm-nih- gov.ezproxy3.library.arizona.edu/omim If you are interested in teratology, congenital defects, and the lives of patients who have these conditions, I highly recommend the youtube channel Special Books By Special Kids: https://www.youtube.com/channel/UC4E98HDsPXrf5kTKIgrSmtQ Their videos consist of interviews with patients (young and old) who live with various congenital conditions. If you are interested in psychiatry, they also interview patients with mental health diagnoses too. Why Embryology? Why do we care about how human anatomy develops from a single cell to a fully independent infant? In my view, embryology is helpful to physicians in training in two ways. The first has to do with understanding common congenital diseases and how they can affect your patient’s health, quality of life, your treatment algorithms. Engaging with human development is helpful to understanding the most common ways that building a human can go wrong: no matter your specialty, you will encounter patients who have a range of congenital conditions that have adverse effects on your patient’s health. Furthermore, having a grasp of development will help you understand when different structures are vulnerable to environmentally-based developmental injury during pregnancy, or what kinds of medical interventions a premature baby needs to survive (e.g., are their lungs functional?). An additional thread to embryology is that it does not occur in isolation: embryological development is inextricably linked to maternal health. Although we will not be able to delve into every aspect of how women’s health is impacted by embryology (and by extension pregnancy), I want you all to keep it in the back of your minds as we go through each block toward Life Cycle. As we learn about each system through the pre-clerkship curriculum, we will work through problems so that you can practice your knowledge of clinical applications of human development. I have made an effort to focus on the most high-yield areas of clinical knowledge both for clerkship and for STEP. The second reason embryology is useful is that it can be used to help you build a conceptual framework for understanding of the how and why of anatomy (in addition to physiological and biomechanical principles). For example, how do we get four heart chambers with different functions, participating in different circulations? Well, because during development, the heart grows a wall that separates atria from ventricles, and simultaneously a wall that separates right from left sides. Why is the stapedius muscle in the inner ear, the smallest skeletal muscle in the body, innervated by a different nerve from other muscles of the inner ear? Because the stapedius develops from a different pharyngeal arch (arch 2) than the other muscles (arch 1). This might seem like esoteric knowledge at first, but learning anatomy is like drinking from a fire hose. Therefore, understanding how and why can help you organize your knowledge into discrete, learn-able chunks. During my embryology lessons, you are going to see a lot of dates (i.e., in which week a particular structure forms). I present dates so that you both have context to organize your understanding of how different structures form, and when different forming structures are most vulnerable to environmental injury. You will be expected to have a general sense of these periods of vulnerability (critical periods), and you will be expected to relate the developmental basis underlying a presented clinical problem. Block: Foundations | PROFFITT [7 of 44] FOUNDATIONS OF EMBRYOLOGY AND EARLY DEVELOPMENT For example, I could present you with a 10-year old patient that is easily fatigued and has a large systolic heart murmur. If you understand how the heart forms and can contrast the presented signs and symptoms against your understanding of normal heart anatomy and function, you should be able to develop a hypothesis of what the problem is with this patient. What I will not do is simply ask you when a certain structure forms, except in cases where it is clinically important (e.g., lung development). LO 1 Navigating an Embryo Being able to navigate an embryo in 3D is a critical skill to interpreting all of the embryology we will learn throughout the pre-clerkship curriculum, primarily because an embryo doesn’t look like a mature human and because we will be spending a lot of time looking at planes of section rather than an entire embryo. Many of the directional and planar terms are either the same between postnatal humans and embryos or are synonyms, so let’s start there (Fig. 1). Figure 1: Directional and planar terms in the embryo. (A) directional terms in a postnatal human. (B) directional terms in an embryo. Not pictured are terms that are used in the limbs in both postnatal humans and embryo: proximal = closer to trunk, distal = farther from trunk. (C) Sagittal plane of section dividing embryo into left/right halves-median plane or midsagittal is a specific cut right down the middle. (D) Transverse section dividing embryo into cranial/caudal halves. (E) Frontal section dividing embryo into ventral/dorsal halves. Moore et al. 2019. The Developing Human. Fig. 1.5 Block: Foundations | PROFFITT [8 of 44] FOUNDATIONS OF EMBRYOLOGY AND EARLY DEVELOPMENT Cranial in an embryo refers to the direction closer to the head (synonymous with superior in postnatal humans). Caudal in an embryo refers to being closer to the tail (inferior in postnatal humans). Ventral in an embryo means closer to the belly (anterior in postnatals), whereas dorsal means closer to the back (posterior in postnatals). Lateral in both embryos and postnatal humans refers to being farther from the midline (median or midsagittal plane), whereas medial refers to being closer to the midline. As in postnatal humans, superficial refers to structures nearer the skin, whereas deep refers to structures nearer the interior core of the body. For example, the gut tube in an embryo is deep to the somites. Because limbs can move and are rotated in development, two terms are used to refer to position along a limb in both embryos and postnatals. Proximal in a limb means closer to the trunk, whereas distal means farther from the trunk. LO 2 Most of our future activities will begin around the end of week 3-4 and proceed through organogenesis. To help you orient yourself, I want to introduce you to several key anatomical features of an embryo that emerge in Week 4 after the body wall has closed (Fig. 2). Spend some time trying to match these structures to the mature ones on your own body. The head of an embryo begins as quite large due to the enlarged, forming brain that sits right underneath the outer layer. On the surface of the head, the nasal pit, optic placode, and otic placode begin to form. These are surface layer components of the future nose, eye, and ear. Figure 2: Lateral view of an embryo at 28 days showing major anatomical features. The pharynx (future mouth) is not visible but is medial to the first pharyngeal arch. The cloaca (future anal/urethral openings) is present on the midline just caudal to the umbilical cord. Moore et al. 2019. The Developing Human. Fig. 5.9 Block: Foundations | PROFFITT [9 of 44] FOUNDATIONS OF EMBRYOLOGY AND EARLY DEVELOPMENT The developing embryo also has a pharynx (oral opening) and cloaca (future opening of anus and urethra). These openings start out covered by a thin membrane which erodes away early in development. These openings, like in a postnatal adult, are connected by a gut tube, which later differentiates into the GI tract. Surrounding the pharynx on either side are a series of 5 pharyngeal arches (also called branchial arches). These structures contribute to a large variety of structures in the head, face, and neck. Looking at the body of the embryo, early in development are a series of segmented prominences running along the future spinal cord and vertebral column. These segmented structures are called somites, which are important to the development of bones and muscles. There are also a series of 4 limb buds, which correspond to the future limbs. Alongside the gut tube, if you were to cut into the embryo transversely you could observe the large heart forming up near the head (visible even externally). Each of these organs lie within the body cavity (coelom) which houses the developing internal organs and in the future will form the thoracic, abdominal, and pelvic cavities. Figure 3: Relationship of the embryo with the amnion, yolk sac, connecting stalk, chorion, and placenta. The allantois is the small structure pictured between the blood vessels of the connecting stalk. The chorion is pictured as the chorionic plate, which lies on the placenta. Sadler (2019), Langman's Medical Embryology, Fig. 8.16A Aside from the embryo itself, it is surrounded by a series of extraembryonic structures (Fig. 3) that serve various functions and integrate the embryo with the placenta, which allows for nutrient and gas exchange between the embryo and the mother. The first of these is the amnion, which forms a fluid- filled sac that mechanically supports the developing embryo, maintains constant atmospheric pressure, and serves as a reservoir for waste disposal later in development. Another is the secondary yolk sac (or just yolk sac), which is important early in development for generating blood cells. Block: Foundations | PROFFITT [10 of 44] FOUNDATIONS OF EMBRYOLOGY AND EARLY DEVELOPMENT Anchoring the embryo to the tissue of the uterus is the connecting stalk (or umbilical cord), which contains blood vessels that carry in O2 and nutrients from the placenta and remove CO2. Within the connecting stalk is the small allantois, which runs from the primitive bladder and early in development does waste disposal before the amnion takes over. Lining the outside of the amnion is the chorion, which forms the intermediate layer between the embryo and the placenta. The chorion is the location of blood vessel projections (villi) that exchange with the maternal circulation. The placenta itself is composed of an inner embryonic component and an outer component from the maternal endometrium of the uterus. Block: Foundations | PROFFITT [11 of 44] FOUNDATIONS OF EMBRYOLOGY AND EARLY DEVELOPMENT LO 3 Mechanisms of Development: Cellular & Molecular Control The developmental story we have begun to tell does not happen by magic: it is the result of numerous processes that occur within and between cells. These processes are mediated by cellular communications that alter gene translation to communicate developmental orders within and between cells (signaling). This is how cells “know” what to develop into. These signaling pathways result in the changing anatomy of the developing embryo (morphogenesis), which is achieved through highly regulated cell movements, controlled cell death/proliferation, as well as changes to the behavior, size, and shape of individual cells. Disruption of embryonic signaling and morphogenesis is a primary cause of birth defects. Cells talk to each other through several means, including: Direct communication with adjacent cells through gap junctions Adhesion to molecules on adjacent cells which alters gene expression Signaling to neighbors with growth factors that act on neighboring cells to alter gene expression. The process by which neighboring cells influence other nearby cells to follow a particular developmental program is called induction. All of these communication methods result in changes to activities within the cells, including the expression of regulatory genes that, when translated, produce transcription factors that directly alter gene activity in a cell. When activated across a whole cell population, these regulatory genes are turned on or off in different configurations to assemble tissues. Think of these regulatory genes as master switches that control the fate of cells-they can tell a cell they will be a certain type of tissue, or they can even tell a population of cells where they are in the body. An important example of regulatory genes that control positional identity include the Hox genes. These genes are organized into gene clusters with up to 9 related genes in a row along the genome. These genes are primarily involved in providing positional information and are highly evolutionarily conserved among bilaterian animals. In all mammals there are 4 Hox gene clusters, labeled a, b, c, d. The order of the genes in human DNA is the same as the order in fruit flies, which goes to show how important they are! From the hindbrain segments through to the tail, each segment of the embryo is specified by a pattern of expression of one or more Hox genes. The different combinations of Hox gene expression designate a general “address” along the craniocaudal axis of the embryo (Fig. 4). Block: Foundations | PROFFITT [12 of 44] FOUNDATIONS OF EMBRYOLOGY AND EARLY DEVELOPMENT Figure 4: Hox genes and vertebral patterning in a mouse (Larsen, 3rd ed, Fig. 4-22) Clinically, there are many genes involved in development that are important, however I am going to focus on a small cast of characters that you will encounter in the future for high-yield concepts (Table 1). Gene Product Impact Hox genes Transcriptoin factors programming Aids in segmental “identity” of position al information structures in embryo. Issues can cause cervical ribs or other “location” problems. Sonic hedgehog Shh (sonic hedgehog)-an important Limb differentiation, CNS growth factor development, anteroposterior axis formation Wnt family genes Wnt growth factors e.g. Wnt-7 Limb development, reproductive differentiation FGF family genes FGF growth factors Limb growth Table 1: Important genes and their products in human development Block: Foundations | PROFFITT [13 of 44] FOUNDATIONS OF EMBRYOLOGY AND EARLY DEVELOPMENT Clinical Correlation: Holoprosencephaly Mutations in the Sonic hedgehog genes (Shh) can result in a condition where the hemispheres of the brain fail to develop called holoprosencephaly. This condition ranges highly in severity (Fig. 5), but most cases result in death. The most extreme cases cause failure to lateralize in the face, resulting in ocular, nasal, and oral defects. Figure 5: Holoprosencephaly is highly variable in severity, ranging from cyclopia with a proboscis, to cleft palate, to few externally visible signs. Blaas et al. (2018). Obstetric Imaging: Fetal diagnosis and care. Fig. 39.4. We won’t get into too many specifics about the ways cells change shape or behavior to facilitate development, but one major mechanism I want you to focus on in particular is apoptosis, which is programmed cell death. Pre-programmed cell death is essential to healthy development and is not to be confused with pathological cell death (i.e., necrosis). Disorders of apoptosis can occur during development. For example, insufficient apoptosis: during limb formation can result in tissue retained between digits (syndactyly), which is a common (1 in 2000 births) congenital defect. Apoptosis is one of our very first lines of defense against cancer. The body can identify cells that are dividing out of control and cause them to undergo programmed cell death. Only cells that escape apoptosis go on to cause cancer. Block: Foundations | PROFFITT [14 of 44] FOUNDATIONS OF EMBRYOLOGY AND EARLY DEVELOPMENT LO 4 Germ Layers: The Building Blocks of Development Figure 6: Embryo at approximately 3 weeks showing 3 distinct cellular layers: Ectoderm, mesoderm, and endoderm. These layers will form all tissues of the body. Note that the chorion and part of the amnion here is labelled as extraembryonic mesoderm. Carlson (2019), Human Embryology & Developmental Biology, Fig. 5.6. Embryology involves a lot of four-dimensional changes (through space and time) that are challenging to visualize. Germ layers form the most basic subdivisions (chunks) on which all future morphological interactions in the embryo and fetus will be based-each of the 3 germ layers and their types form all tissues in the body. A solid grasp of the behaviors of these epithelial (lining cells ectoderm and endoderm) and mesenchymal (undifferentiated connective tissue cells like mesoderm and neural crest) tissues will inform your understanding of normal and abnormal anatomy and histopathology in future blocks. We will begin our journey with germ layers by covering the basics of each of the 3 subtypes: Ectoderm, Mesoderm, and Endoderm. At the end of week 3, the embryo looks like a sandwich where each of these cellular populations forms one “layer” of the sandwich (Fig. 6). Type 1: Ectoderm Ectoderm Type Position Derivatives Surface Ectoderm Dorsal surface of embryo Skin/glands, hair and nails; tooth enamel; part of lateral to notochord pituitary; lens of the eye; inner ear; nasal lining. Neural Plate Dorsal surface of embryo Central nervous system (brain, spinal cord); retina; Ectoderm dorsal to notochord somatic (conscious) motor neurons of brain and spinal cord. Neural Crest Cells Sit on either side of neural Bones/cartilages of face/pharynx/larynx, optic muscles, plate, migrate to different cartilages, cornea, pulp of teeth, parathyroid and thymus areas. glands, sensory neurons, autonomic (involuntary) motor neurons, enteric plexus of gut, Schwann cells, septa of great vessels of heart, pigment cells, adrenal medulla, meninges of central nervous system. Table 2: Summary of Ectoderm Derived Structures The outermost layer and the “top” layer of the germ layer is the ectoderm (Table 2), comprising 3 divisions: surface ectoderm, neural plate ectoderm, and neural crest ectoderm. Block: Foundations | PROFFITT [15 of 44] FOUNDATIONS OF EMBRYOLOGY AND EARLY DEVELOPMENT The surface ectoderm is involved in most of our integumentary structures (skin, hair, nails, enamel of teeth). It also interacts with the neural plate ectoderm to create hormonal and sensory structures as well. An outpocketing of surface ectoderm forms part of the anterior pituitary gland, for example. Areas of thicker surface ectoderm form at specific locations on the developing head to incorporate into sense organs (nasal pit, optic placode, otic placode). The neural plate ectoderm is found along the midline dorsal surface of the embryo and will become the central nervous system. It also contributes to other nervous structures (e.g., retina, voluntary motor neurons, etc). The last division of ectoderm is the most confusing: the neural crest cells. Neural crest cells form along each side of the neural plate ectoderm. Neural crest cells are among the most diversified cells in the embryo and contribute to a wide range of structures by migrating to different areas. They are particularly important in the developing face but form many other things too. Note that they have a habit of contributing to structures built by other germ layers (e.g., adrenal medulla). Block: Foundations | PROFFITT [16 of 44] FOUNDATIONS OF EMBRYOLOGY AND EARLY DEVELOPMENT Clinical Correlation: Ectoderm Disorders Surface ectoderm: Ectodermal dysplasia (Fig. 7). Failure of any of more than 180 individual genes can disrupt the normal development of surface ectodermal structures, leading to sparse hair, absent or pegged teeth, and an inability to sweat (anhydrosis due to sweat gland aplasia). Figure 7: Patient exhibiting ectodermal dysplasia, including pegged teeth, fine hair, and pointed ears. Fig. 668.1, Abidi et al., 2020, Nelson Textbook of Pediatrics Neural plate ectoderm: Anencephaly (Fig. 8). Lack of folic acid or teratogen exposure can impact the formation of the nervous system from the neural plate ectoderm. This can prevent the brain from forming correctly, resulting in an open lesion. Figure 8: Anencephaly. Note the vascular defect indicated by the arrow-this feature was visible on ultrasound. Kamaya et al. 2019. Diagnostic Ultrasound for Sonographers. Neural crest ectoderm: Treacher-Collins (Fig. 9). Causes patients to have underdeveloped facial bones characterized by insufficient neural crest cell division in the face, which can cause pain and difficulties in chewing, breathing, and speech. Hearing impairment is also typical. Figure 9: A 30 year old patient exhibiting Treacher Collins syndrome (top row) with post-operative comparison (bottom row). Note reduced facial structure in preoperative example. Fig. 10; Case 2, Wolford et al. 2015, Oral and Maxillofacial Surgery Clinics. Block: Foundations | PROFFITT [17 of 44] FOUNDATIONS OF EMBRYOLOGY AND EARLY DEVELOPMENT You will see neural crest cells again and again throughout our embryology journey. For example, neural crest cells act in heart development: they help to build the tissue that separate heart chambers, as well as the wall that separates inflow/outflow tracts of the heart Abnormal migration, proliferation, and differentiation of neural crest cells can result in congenital problems in the heart. Therefore, it is not uncommon to see facial congenital defects and heart congenital defects in the same patient (think of this later when we talk about DiGeorge syndrome in a later LO). Type 2: Mesoderm The second germ layer, sandwiched between the ectoderm and the endoderm, is the mesoderm (Table 3). The mesoderm forms much of the musculoskeletal system and is also responsible for the kidneys and gonads. Based on its position relative to the other germ layers and the midline of the embryo, we can divide into three types: Paraxial, intermediate, and lateral plate mesoderm. Lateral plate mesoderm, in turn, has its own two divisions: somatic (lining body wall) and visceral (lining endodermal organs) (Fig. 10). Mesoderm Division Derivatives Paraxial Mesoderm Muscles of head, neck, body wall, and limbs; tongue; posterior bones of skull, ribs, vertebrae Intermediate Mesoderm Kidneys; Cortex of suprarenal glands; gonads, reproductive ducts; Lateral Plate Mesoderm Somatic: bones, fat, and connective tissue of limbs; internal (parietal) lining of organ bursae against the body wall; allantois Splanchnic: Heart; spleen; smooth muscle of GI and respiratory tracts; mesenteries and supportive tissue of GI structures; allantois, secondary yolk sac. All layers Blood vessels Table 3: Summary of structures derived from mesoderm Figure 10: Division of the mesoderm into separate segments. Paraxial mesoderm here is only labelled as as omite. Note the splitting of the lateral plate mesoderm into somatic and visceral (splanchnic) sections, making room for the coelomic cavity. Most paraxial mesoderm divides into the somites, repeating epithelial balls lined up on either side of the neural tube. Along with the pharyngeal arches, somites show a segmented pattern Block: Foundations | PROFFITT [18 of 44] FOUNDATIONS OF EMBRYOLOGY AND EARLY DEVELOPMENT that is present in vertebrate animals but obscured by adult anatomy. Through somites paraxial mesoderm forms axial skeleton, the skin dermis, and most skeletal muscles of the body. Lateral to the somites, the intermediate mesoderm will form mesodermal structures of the urinary system, adrenal cortex, and the reproductive system. Lateral to the intermediate mesoderm, the lateral plate mesoderm (LPM) will split into two layers, an inner layer and outer layer, separated by the body cavity (coelom). The outer layer is the somatic (or parietal) mesoderm, forming the outer/dorsal layer in contact with the surface ectoderm. It will go on to form bones, fat, blood vessels, and connective tissues of the limbs, and the non-muscular parts of the body wall. The inner layer visceral (aka splanchnic) mesoderm is the inner/ventral layer in contact with the endoderm. It contributes the heart and spleen; and to smooth muscle, mesenteries and supportive connective tissue of the GI tract, respiratory tract, and lowermost urinary/reproductive tracts. Also contributes to extraembryonic structures like the allantois and secondary yolk sac. All layers of the mesoderm contribute to the formation of blood vessels (angiogenesis). The differences between somatic and visceral lateral plate mesoderm is demonstrated by the development of the heart. The heart develops from splanchnic lateral plate mesoderm due to signals from the adjacent endoderm. The embryonic heart begins to grow inside the embryonic thoracic cavity (intraembryonic coelom) and is surrounded by somatic lateral plate mesoderm. This surrounding mesoderm will form the parietal (outer) and fibrous layers of the pericardial sac, which surrounds and protects the heart. Block: Foundations | PROFFITT [19 of 44] FOUNDATIONS OF EMBRYOLOGY AND EARLY DEVELOPMENT Clinical Correlation: VACTERL Association Problems with the mesoderm typically manifest in correlated sets of congenital problems, known as a VACTERL association. This acronym lists the most common manifestations: (V)ertebral malformations (A)nal atresia (due to failure of the mesodermal septum to divide the cloaca appropriately) (C)ardiac defects (T)racheo-Esophageal fistula (again due to failure of the mesodermal septum to divide the trachea from the esophagus) (R)enal anomalies (L)imb abnormalities (Fig. 11). The causes of VACTERL association are not fully understood, but evidence indicates multiple genetic and environmental (teratogen) causes. If you detect more than one of these in a newborn (or the stem of a board question), it is worthwhile to screen for the others. Someone with a VACTERL diagnosis typically has at least 3 of these features. Remember that major problems with a germ layer can result in correlated outcomes across that germ layer’s derivatives! Figure 11: Common upper limb malformations observed in VACTERL association. Carli et al., 2014, The Journal of Pediatrics, Fig. 3 Block: Foundations | PROFFITT [20 of 44] FOUNDATIONS OF EMBRYOLOGY AND EARLY DEVELOPMENT Type 3: Endoderm The last germ layer we will cover is the endoderm, which is involved in the formation of many of the internal organs (Table 4). The endoderm is the bottom layer of the “sandwich” of the germ layers. Endoderm Division Derivatives Gut Tube Pharynx; thyroid gland, foregut (esophagus, stomach, ½ of duodenum); midgut (1/2 of duodenum, small intestine, cecum, appendix, ascending colon, transverse colon); hindgut (descending colon, sigmoid colon, rectum); Outpocketings off Gut Tube Trachea & lungs; liver; gallbladder; pancreas; secondary yolk sac Table 4: Summary of endoderm derivatives The endoderm begins as a single layer of cells connected to the secondary yolk sac. As the embryo grows to form the body wall the endoderm becomes enclosed inside the body, with the exception of the connection to the yolk sac. This new gut tube is connected at one end to the pharynx and at the other end to the cloaca (Fig. 12) The gut tube remains attached to the yolk sac via an open vitteline duct. At the caudal end of the gut tube, proximal to the anal membrane, there is an outpocketing of the tube that forms a primitive bladder, which is drained via the allantois. Figure 12: Lateral view of endodermal structures (gut tube; budding structures; connections to extraembryonic structures) enclosed within the embryo. Note that the opening of the pharynx is labelled as the stomodeum in this image. Often due to signals from nearby tissues, the gut tube begins to differentiate into the lining and tissues of different visceral organs. This process allows the endoderm to differentiate into its many derivatives. Block: Foundations | PROFFITT [21 of 44] FOUNDATIONS OF EMBRYOLOGY AND EARLY DEVELOPMENT Clinical Correlation: Volvulus The endoderm undergoes many processes of differentiation and looping to fit inside the body. An example of looping gone wring is called volvulus (Fig. 13). This can compromise blood supply and result in hypoplasia of affected regions of the gut or even full strangulation. Figure 13: Volvulus in the developing gut tube. Here the volvulus has occured in the beginning of the developing small intestine. Schoenwolf et al. (2021). Larsen’s Human Embryology, Fig. 14.20 Block: Foundations | PROFFITT [22 of 44] FOUNDATIONS OF EMBRYOLOGY AND EARLY DEVELOPMENT Figure 14: Flowchart of major human germ layer derivatives. Carlson et al. (2019); Human Embryology & Development Fig. 6.29 By the end of your preclerkship work, you should be able to name most major body structures and recall its developmental origin (Fig. 14). DO NOT TRY TO MEMORIZE Fig. 18 NOW. Instead, focus on the main patterns described in the lecture notes above and refer back to it as you need. Some derivatives are not shown. Note that some structures have a dual origin. Block: Foundations | PROFFITT [23 of 44] FOUNDATIONS OF EMBRYOLOGY AND EARLY DEVELOPMENT LO 5 Outline of Human Development Human development from fertilization to birth typically takes about 38 weeks. In embryology, each week is numbered according to how much time has passed since fertilization. Often, 40 weeks is quoted as a healthy full term pregnancy-this is because the time from the last menstrual period is added to the total number of weeks. Irritatingly, the trimester system (3 periods of 12-14 weeks) only begins counting from the first missed period. Therefore, once a woman has missed her first period the embryo is on average 2 weeks into development (assuming a constant frequency of menstruation every 4 weeks with ovulation occurring in the middle of that period). Figure 15: Timeline of development including ovulation prior to fertilization. Shows general pattern of cell division and growth prior to and following implantation (day 6). Moore et al. 2019. The Developing Human. Fig. 1.1 The initial two weeks of development consist of initial cell growth, implantation into the endometrium, and development of the embryo into a two-layered structure (Fig. 15). Block: Foundations | PROFFITT [24 of 44] FOUNDATIONS OF EMBRYOLOGY AND EARLY DEVELOPMENT The next period is called the embryonic period (weeks 3-8). During this period most of the major organs begin forming (organogenesis), the body wall closes, and the embryo begins to take on a more human-like appearance (Figs. 16, 17). Organs generally form from cranial to caudal, so organs closest to the head form/mature first. Figure 16: Weeks 3-6 of development. Note the changes in embryo shape and the beginning of organ formation. Moore et al. 2019. The Developing Human. Fig. 1.1 Block: Foundations | PROFFITT [25 of 44] FOUNDATIONS OF EMBRYOLOGY AND EARLY DEVELOPMENT During weeks 8-40 the embryo enters the fetal stage and continues to grow and mature (Fig. 17). The overall body size of the fetus increases dramatically. Figure 17: Generalized outline of embryo/fetal growth from weeks 7-10. Note that most organs have begun forming by the end of week 8. As noted above, note that organs that are more caudal (e.g., genitals) form later than other organs. Moore et al. 2019. The Developing Human. Fig. 1.1 LO 6 Congenital defects can occur for multiple reasons, and in fact the cause of most defects are unknown (Fig. 18). In general, we conceptualize congenital defects as occurring due to the following factors: Mutation: changes in the nucleotide sequence of a gene that affects its function (which can include regulation of other genes) Numeric chromosome abnormalities (aneuploidy): there is an incorrect number of a chromosome or chromosomes (too many or too few). This can affect autosomes or the sex chromosomes. Structural chromosome abnormalities: the chromosomes are not arranged correctly (for example, a piece of one chromosome has translocated to another chromosome from a different pair) Environmental factors: exposure to environmental agents such as chemicals, viruses, bacteria, medications, or radiation can interfere with development. These factors can also induce mutations or influence chromosomes. Mutifactorial Inheritance: developmental diseases that are caused by both genetic and environmental factors acting in tandem. Block: Foundations | PROFFITT [26 of 44] FOUNDATIONS OF EMBRYOLOGY AND EARLY DEVELOPMENT Figure 18: Causes of human birth defects sorted by percentage frequency. Note that the majority are unknown and the second largest category involves a combination of genetic and environmental factors. Moore et al. 2019. The Developing Human. Fig. 20.3 Figure 18: Outline of critical periods for different organs. Note that the nervous system is sensitive longer than other systems and that the type of developmental injury can change throughout the critical period. We will learn what a lot of these individual congenital anomalies are throughout pre-clerkship-for now just focus on the fact that the earlier the injury the more severe the outcome. Moore et al. 2019. The Developing Human. Fig. 20.1 Clinically, understanding the general timing of when major organs forms impacts when these systems are most vulnerable to environmentally-derived injury (Fig. 18). These time periods are called critical periods. Typically, early in development (weeks 1-2) is known as the “all or nothing” phase- developmental injury during this period will typically result in death of the embryo and subsequent Block: Foundations | PROFFITT [27 of 44] FOUNDATIONS OF EMBRYOLOGY AND EARLY DEVELOPMENT abortion of the pregnancy. Generally, developmental injuries during the embryonic period (weeks 3-8) will result in more severe congenital anomalies, whereas developmental injuries during the fetal period (weeks 9-38) will cause relatively more minor anomalies or functional injuries. Developmental injuries that occur during critical periods can be caused by exposure to teratogens or by congenital infection where a pathogen passes from mother to embryo (see Table 5 for some examples). Agent Subtype Common Defects Alcohol Recreational drug-teratogen Cognitive disability, fetal alcohol syndrome Nicotine Recreational drug-teratogen Low birth weight, preterm labor, placenta issues from vasoconstriction. ACE Inhibitors Medication-teratogen Renal failure, skull defects, oligohydroamnios Warfarin Medication-teratogen Bone defects, death, eye anomalies, cognitive defects. Hepatitis B Virus-congenital infection agent Preterm birth, macrosomia Varicella Virus-congenital infection agent Scars, neurological defects, cognitive problems, skeletal anomalies, urogenital anomalies X-Rays Radiation Microcephaly, cognitive delay, skeletal problems. Table 5: Relevant teratogens and pathogens that cause developmental injury LO 7 Types of developmental injuries are differentiated by several factors, including whether it is genetically determined, whether it is influenced by environmental causes or outside forces, and where the injury is located (Table 6). Terms also exist to describe the impact these injuries can have on tissues (Table 6). Term Subcategory Definition Malformation Injury Type Injury is caused by a defective developmental process (e.g., chromosomal abnormality, inherited mutation). Disruption Injury Type Injury is caused by normal development being interrupted by an extrinsic factor (e.g., teratogen, virus). Deformation Injury Type Injury is caused by exposure to outside forces (e.g., physical pressure on the embryo). Aplasia Impact on Tissue Tissue does not form Hypoplasia Impact on Tissue Tissue forms normally but is small Dysplasia Impact on Tissue Tissue is disorganized and forms incorrectly Field Defect Disease Type Multiple defects resulting from injury to a localized area Syndrome Disease Type Multiple defects across multiple regions that are pathogenetically related Sequence Disease Type Multiple cascading defects caused by a single known structural defect or mechanical factor Association Disease Type Nonrandom occurrence of two or more defects where the direct causal link between them is not fully known. Table 6: Glossary of common terms for congenital injuries and diseases. Block: Foundations | PROFFITT [28 of 44] FOUNDATIONS OF EMBRYOLOGY AND EARLY DEVELOPMENT Clinical Correlation: Examples of developmental disease types Examples of different developmental injuries can help clarify how each. Note that the types are not mutually exclusive: for example, Prader-Willis is an example of a field defect as well as a sequence ( Field Defect example: Pierre-Robin Sequence (Fig. 19). Typified by development of a small lower jaw. However, it is also a sequence because micrognathia results in displacement of the tongue, which impacts palatal development too. Figure 19: Pierre-Robin Sequence. Note the tiny lower jaw. Sadler (2019), Langman's Medical Embryology, Fig. 17.16A Syndrome example: DiGeorge Syndrome (Fig. 20). Caused by a problem with neural crest cells, which are part of the ectoderm germ layer, due to a defect on chromosome 22. Impacts jaw and face formation, palatal formation, heart formation, parathyroid and thymus gland formation, cognition, etc. Figure 20: DiGeorge Syndrome. Note the small lower face, low-set ears, and reduced furrow in upper lip. Sadler (2019), Langman's Medical Embryology, Fig. 17.16B Sequence example: Potter sequence. Caused by a primary failure in kidney development, this causes a lack of urine production, compression of the fetus due to lack of amniotic fluid, and subsequent hypoplasia of lungs (inhalation of amniotic fluid is key to lung inflation). Association example: VACTERL Association. VACTERL is typified by a series of defects that commonly occur together, including issues with vertebrae, heart, trachea and esophagus, anus, etc. Related to the mesoderm germ layer-see below for more info. Block: Foundations | PROFFITT [29 of 44] FOUNDATIONS OF EMBRYOLOGY AND EARLY DEVELOPMENT LO 8 Week 1: Fertilization to Implantation (Rule of 1s) The initial form of the embryo is a diploid (23 pairs of chromosomes), single-celled zygote wrapped in a glycoprotein shell. The zygote is formed by the combination of haploid sperm and egg (23 individual chromosomes each), which is called fertilization. Fertilization typically takes place in the uterine tube. The fertilized zygote then travels down the uterine tube via movement of ciliated epithelial cells and muscular contraction of the tube into the uterus over the course of the next few days (Fig. 21). Figure 21: Diagram demonstrating the fertilization of the embryo and growth of the zygote as it travels into the uterus (left); schematic view of a blastocyst-stage embryo, illustrating the inner cell mass that becomes the embryo, and the outer cells (trophoblast) that becomes the placenta (right) During the embryo’s journey into the uterus, the zygote divides four times. This process yields a ball of small cells organized into inner and outer layers. This layered organization is important because the inner cells (called the inner cell mass or embryoblast) will be the cells that form the body, whereas the outer cells (outer cell mass or trophoblast) will form the embryonic contribution to the placenta. As the embryo enters the uterus (around day 4-5), the trophoblast cells pump fluid from the uterine cavity into small spaces between the cells of the embryoblast. This process creates a small cavity (blastocoel) that is lined by trophoblast and embryoblast cells, resulting in a stage known as the blastocyst (Fig. 21). LO 9 Typically, human pregnancy results in one child. The development and birth of two children is known as twinning, and it can occur via two processes. Fraternal or dizygotic twinning is the result of two eggs being ovulated by separate sperm. These eggs then grow together in the uterus with separate placentas (although exceptions with shared placenta exist, see (1)). Dizygotic twins are genetically only as related as any typical siblings. Identical or monozygotic twinning is the result of a single fertilized egg dividing into two embryos sometime between the 2-cell and primitive streak stage (Fig. 22). Division into two separate blastocysts (division by the morula Block: Foundations | PROFFITT [30 of 44] FOUNDATIONS OF EMBRYOLOGY AND EARLY DEVELOPMENT stage) can result in two placentas and separate extra-embryonic membranes (25%), or a single placenta and some shared extra-embryonic membranes (75%), depending on the timing. Later division after the blastocyst stage (rare) results in identical twins within a single amnion and chorion or conjoined twins (even more rare). Twins are typically detected via ultrasound and are at much higher risk of pre-natal health problems or death. Shared placenta (monochorionic) is the most risky, as it means twins share vascular supply. One risk includes Twin-Twin Transfusion Syndrome caused by uneven flow of blood across interconnected vessels, resulting in imbalanced amniotic fluid (typical TTTS) or unidirectional blood flow from one twin to the other (TAPS variant of TTTS). Shared amniotic sacs (monoamniotic) can result in twisted umbilical cords, strangulation, or conjoining. Figure 22: Types of monozygotic twinning based on timing. Note how division after 4 days can result in monochorionic twinning. Twinning even later (after 8 days) can result in monoamnionic twinning including conjoined twins. LO 10 As it approaches the uterine lining, the blastocyst sheds its shell and attaches to the thick, vascularized uterine lining (endometrium) of the mother. This process is known as implantation. The trophoblast then begins to differentiate into different layers to integrate with the endometrium and starts production of the hormone hCG (human chorionic gonadotropin), which maintains hormonal activity by the ovary that sustains the endometrium. Thus week 1 of development yields: 1 embryonic structure (inner cell mass or embryoblast) 1 fluid filled sack (blastocoel) 1 placental precursor layer (trophoblast). Block: Foundations | PROFFITT [31 of 44] FOUNDATIONS OF EMBRYOLOGY AND EARLY DEVELOPMENT Clinical Correlation: Ectopic Pregnancy Rarely, implantation of the developing embryo occurs outside of the uterine cavity. This is known as an ectopic pregnancy. The vast majority of ectopic pregnancies occur within the uterine tube, but implantation on the ovary, cervix, or even structures within the abdominal cavity can occur (Fig. 23). Growth of an ectopically-implanted embryo is almost always life-threatening due to rupture of the structures surrounding the growing embryo potentially resulting in severe hemorrhage, and is typically diagnosed via ultrasound and hCG serum tests. The ectopic embryo is then removed either through medication or surgery. Figure 23: Common locations of ectopic pregnancy implantation LO 11 Week 2: Getting Set for Gastrulation (Rule of 2s) Week 2 is primarily set dressing for week 3 when gastrulation occurs. This involves 3 main processes: 1. The embryoblast separates into two distinct layers, a top layer that will form the embryo (epiblast) and a bottom layer (hypoblast) that supports and sends organizing signals to the embryo. Together these form the embryonic disc (Fig. 24). Block: Foundations | PROFFITT [32 of 44] FOUNDATIONS OF EMBRYOLOGY AND EARLY DEVELOPMENT Figure 24: Cross section of the blastocyst at around 9 days. Note the divided embryoblast with the amniotic sac in between the two layers. Note also how the blastocoel has been coopted to form an extracoelomic cavity with the primary yolk sac. 2. The epiblast divides into two layers and pumps fluid between them to form the amniotic cavity. Epiblast tissues in contact with the hypoblast will contribute to the embryo. The remainder forms the amniotic membrane. The amniotic membrane will later expand and be joined by a layer of tissue from the newly formed mesoderm germ layer (see below) to form the amnion, a fluid-filled sack that surrounds and supports the embryo. The hypoblast also expands until it connects across the blastocyst and forms a second extraembryonic membrane (primary yolk sac). This layer is involved with the development of extraembryonic tissues like the chorion (2). The primary yolk sac is later replaced by a definitive secondary yolk sac connected to the gut, which is involved in several developmental processes like early hematopoiesis and origin of the primary germ cells (future sperm and eggs). 3. As the epiblast divides, the trophoblast also divides into two layers. The inner layer stays cellular (cyto-trophoblast layer) and the outer layer fuses into a mass of large cells with multiple nuclei and shared cytoplasm called a syncytium (syncytio-trophoblast layer) that interacts with maternal tissues (Fig. 25). We will return to this structure during the Life Cycle Block, but for now recognize that placenta is formed out of multiple layers of embryonic tissue interacting with maternal endometrium. Block: Foundations | PROFFITT [33 of 44] FOUNDATIONS OF EMBRYOLOGY AND EARLY DEVELOPMENT Figure 25: Implantation and development of the bilaminar embryonic disc and two layers of placental tissue. Note the developed amniotic cavity and primary yolk sac During week 2, the embryonic disk increases greatly in size due to cellular division. Most of the expansion is in the cranial direction and the disk changes from a circular to an ovoid shape (wider at the head end like a lightbulb). By 16 days, the embryonic disk is about 1 mm long and about 0.5 mm wide. This is about the point when a patient might notice a late period. Thus week 2 yields: 2 embryonic layers (epiblast & hypoblast) 2 extraembryonic membranes (amnion and primary yolk sac) 2 placental cell types (cyto-trophoblasts and syncytio-trophoblasts). LO 12, 13 Week 3: Gastrulation (Rule of 3s) The third week consists of a process of cell proliferation and movement called gastrulation. Gastrulation results in a series of three germ layers (Fig. 26). The germ layers represent layers of cells that will go on to form the different components of the adult body. All bilaterians (basically animals more complex than a jellyfish) go through a developmental period when they consist of three different germ layers: outer (ectoderm), middle (mesoderm) and inner (endoderm). During subsequent development, organs and tissues derived from the same germ layer will share certain traits, due to their common origin. Block: Foundations | PROFFITT [34 of 44] FOUNDATIONS OF EMBRYOLOGY AND EARLY DEVELOPMENT Figure 26: Summary of gastrulation, showing the origin of the three primary germ layers. A series of three ‘organs’ form during gastrulation: the primitive streak, the notochord, and the neural plate. The primitive streak is a visible line that starts at the tail (caudal) end of the embryo and extends toward the head end (rostral) on the dorsal side of the embryo (Fig. 27). The line has a narrow groove (primitive groove) surrounded by a thickening at each side and at the head end. The thickening at the rostral end is the node. Cells of the node and primitive groove secrete proteins that control the definition of left-right and dorsal-ventral body axes. Figure 27: Dorsal view of human embryos showing the primitive streak. Fig. 5.1 from Sadler (8th ed). Block: Foundations | PROFFITT [35 of 44] FOUNDATIONS OF EMBRYOLOGY AND EARLY DEVELOPMENT Clinical Correlation: Situs inversus Mutations to cells of the primitive streak that affect the direction and strength of protein gradients that differentiate the right from left side can cause laterality defects in organ placement, which are typically correlated with clinically-relevant functional and structural deficiencies. An example is situs inversus (1 in 10000 births), wherein some structures that are typically on the left are transposed to the right, and vice-versa (Fig. 28). The most extreme example is situs inversus totalis, which affects all organs of the thorax and abdomen (e.g., liver is on the left side, heart is oriented toward the right side). Unlike other laterality defects, situs inversus totalis is rarely symptomatic. Where it is relevant clinically, is in the physician’s ability to localize structures for purposes of examination, imaging, or surgery. For example, a patient with situs inversus totalis and appendicitis may experience pain in the lower left quadrant, rather than the lower right quadrant. Figure 28: A case of situs inversus totalis observed in an PA chest radiograph. Note the shadow of the heart oriented toward the right. Case courtesy of Dr Maulik S Patel, Radiopaedia.org, rID: 32958 As the primitive streak forms, some epiblast cells migrate toward the midline. Upon reaching the primitive streak, some of these cells move through the primitive streak into the interior of the embryo (toward the hypoblast; Fig. 29). The first cells to migrate through the primitive groove insert into the hypoblast, displacing it laterally, and form the embryonic endoderm (day 14 and 15), which will form the future gut tube. Block: Foundations | PROFFITT [36 of 44] FOUNDATIONS OF EMBRYOLOGY AND EARLY DEVELOPMENT Figure 29: Movement of cells through the primitive streak to form the endoderm (left) and mesoderm (B) The next cells to move internally (day 16 onwards) take up a position between the epiblast and the endoderm to form the third germ layer, the mesoderm. When all three layers are present, the remaining epiblast layer becomes known as ectoderm (Fig. 30). The mesodermal cells continue to migrate laterally and cranially, between the ectodermal and endodermal layers, until they occupy almost the entire middle layer of the embryo except for around the future mouth and anal openings. Figure 30: Overview of the trilaminar embryo. Note the 3 separate germ layers, and the growth of the notochord from the primitive node cranially. Also visible are the oral and cloacal membranes. Fig. 3-12 from Schoenwolf et al. 2015. Larsen’s Human Embryology During the formation of the three layers, mesodermal cells originating at the node migrate cranially and form a rigid rod (notochord) until they reach the site of the future oral opening. This rod is the second ‘organ’ formed and it serves as a simple embryonic spine. Later in development, the notochord contributes to the nucleus pulposus of the intervertebral disks of the spine. At this early stage, it is also an essential source of signaling molecules to the adjacent mesoderm and overlying ectoderm. In the ectoderm, it triggers the formation of the third ‘organ’ the neural plate, which will roll into a neural tube by week 4 and later form the entire Central Nervous System. As development proceeds, the node and primitive streak regress caudally and lay down a notochord all the way to the tail end of the embryo (like pulling back a toothpaste tube and Block: Foundations | PROFFITT [37 of 44] FOUNDATIONS OF EMBRYOLOGY AND EARLY DEVELOPMENT leaving behind a rod of toothpaste). The overlying neural plate similarly extends toward the tail to form the precursor of the spinal cord. The streak finally disappears by about day 30. Clinical Correlation: Sacrococcygeal Teratoma If the primitive streak does not disappear completely, it can give rise to embryonic tumors in the coccygeal region containing cells from all three germ layers (Fig. 31). These tumors are known as sacrococcygeal teratomas. These tumors are the most frequent tumors in newborns (1 in 37000 births) and are most common in female infants. These tumors are removed postnatally to improve quality of life and prevent malignancy. Postoperative secondary problems are common, in particular urinary and fecal incontinence. Figure 31: Sacrococcygeal teratoma in female infant. Fig. 4.7, The Developing Human, Moore et al., 2020, pg. 47-63. During this period, the mesodermal and endodermal germ layers combine to form the third extraembryonic membrane (allantois), a primitive sack off of the bladder for removal of nitrogenous waste until the anal membrane opens and urine can be excreted into amniotic fluid for removal by the mother’s circulation. The amnion remains and the primary yolk sac is replaced by a secondary yolk sac of endodermal origin. Thus week 3 yields: 3 germ layers (ectoderm, mesoderm, and endoderm summary in Table 1) 3 extraembryonic membranes (amnion, secondary yolk sac, and allantois) 3 ‘organs’ (primitive streak, notochord, and neural plate). Block: Foundations | PROFFITT [38 of 44] FOUNDATIONS OF EMBRYOLOGY AND EARLY DEVELOPMENT LO 14 Week 4: Body Folding The germ layers begin the process of forming organs in week 4, however we will leave that material until future blocks. For now, let’s focus on the major shape changes that happen to the embryo to transform it from a flat, 3-layered disc into a “tube-within-a-tube” more typical of the animals we know. The first process we will focus on is shape changes in the ectoderm to create the neural tube (future CNS). This process is called neurulation (Fig. 32). Figure 32: Summary of neurulation. During neurulation, the cells of the neural plate change chape to “roll” each side together into a tube. The surface ectoderm then covers this new structure, forming a hollow neural tube that will Block: Foundations | PROFFITT [39 of 44] FOUNDATIONS OF EMBRYOLOGY AND EARLY DEVELOPMENT become the brain and spinal cord. The neural crest cells nearby become mesenchymal, and will subsequently migrate to their different destinations. Clinical Correlation: Spina Bifida Failure of the neural tube to fuse correctly results in birth defects called neural tube defects or NTDs. Anencephaly (‘no brain’) is caused by failure of fusion at the cranial end while Spina Bifida (‘split spine’) is caused by a failure to fuse in the middle or at the caudal end (Fig. 33). Public health programs to supplement folic acid (Vitamin B9) levels in the diet of women likely to become pregnant have led to a decrease in birth defects including NTDs, although the mechanistic link between folic acid and neural tube fusion is not established. Figure 33: Patient suffering from spina bifida (myelomeningocele variant, where the meninges and spinal cord are exposed in the lumbar region). Thompson et al. 2010, Paediatrics and Child Health. Fig. 1 The next process involves folding in two directions: a cranial to caudal fold to place the embryo in the “fetal position” and lateral folding to form the body wall and enclose the endoderm in the body cavity (coelom). Craniocaudal folding is accomplished primarily by growth of the brain, causing the body of the embryo to flex. The head fold and tail folds move ventrally to partially enclose the gut tube and pinch the secondary yolk sac into a smaller area (Fig. 34). Block: Foundations | PROFFITT [40 of 44] FOUNDATIONS OF EMBRYOLOGY AND EARLY DEVELOPMENT Figure 34: Craniocaudal folding in sagittal view. Sadler (2019). Langman’s Medical Embryology, Fig. 7.2. The next process is lateral folding (Fig. 35), where the mesoderm forming the body wall moves ventrally to enclose the gut tube, leaving only openings at the connecting stalk and secondary yolk sac. Block: Foundations | PROFFITT [41 of 44] FOUNDATIONS OF EMBRYOLOGY AND EARLY DEVELOPMENT Figure 35: Summary of lateral folding. Note the position of the different types of lateral plate mesoderm relative to the endodermal gut tube. Note the space between them forms the body cavity (coelom). This folding process unites the left and right halves of the somatic lateral plate mesoderm, forming the body wall that will eventually be composed of muscles, bones, and fascial linings of organs. The visceral lateral plate mesoderm remains adjacent to the endoderm to form smooth muscle and supporting tissue of internal organs. Block: Foundations | PROFFITT [42 of 44] FOUNDATIONS OF EMBRYOLOGY AND EARLY DEVELOPMENT Clinical Correlation: Disorders of Lateral Folding Like the neural tube, the body wall can fail to close along its craniocaudal length, resulting in the herniation of organs outside the body wall. From cranial to caudal, these conditions can include: Ectopia cordis (Fig. 36): In very rare cases folding of the body wall leaves the heart outside of the chest. This is usually lethal around birth, but some individuals have survived beyond 30 years of age Figure 36: Ectopia cordis in a premature fetus. Sadler (2019). Langman’s Medical Embryology, Fig. 7.3 Gastroschisis or Omphalocele (Fig. 37): Gastroschisis is a fairly common birth defect (1 in 2000 births) where visceral organs, usually intestine, lie outside of the body wall due to incomplete closure of the body wall. Unusually, the incidence of gastroschisis in increasing, especially in infants of thin women younger than 20 years old. The difference between gastroschisis and omphalocele has to do with the nature of the herniation: in gastroschisis free loops of intestine herniate outside the body wall, whereas in omphalocele they herniate into the connecting stalk and are covered by amnion.. Figure 37: Gastroschisis (left) and omphalocele (right). Sadler (2019). Langman’s Medical Embryology, Fig. 7.3 Bladder or Cloacal Extrophy (Fig. 38): Abnormal closure of the body wall in the pelvic region can result in herniation of the bladder or more seriously the bladder and rectum. Genital development is often compromised in these conditions. Figure 38: Bladder extrophy (left) in a male & cloacal extrophy (right) in a female. Sadler (2019). Langman’s Medical Embryology, Fig. 7.3 Block: Foundations | PROFFITT [43 of 44] FOUNDATIONS OF EMBRYOLOGY & EARLY DEVELOPMENT References 1. Quintero, R.A. et al. 2003 Twin-twin transfusion syndrome in a dizygotic monochorionic-diamniotic twin pregnancy. The Journal of Maternal-Fetal and Neonatal Medicine. 14: 279-281. 2. Ross, C. and Boroviak, T.E. 2020. Origin and function of the yolk sac in primate embryogenesis. Nature Communications. 11: 3760. https://doi.org/10.1038/s41467-020-17575-w Block: Foundations | PROFFITT [44 of 44]