Electrode Systems and Applications (Unit 1) Notes PDF
Document Details
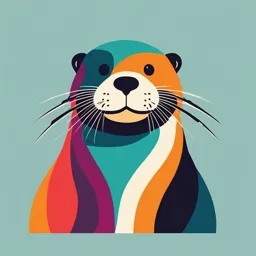
Uploaded by PamperedLimeTree
Siddaganga Institute of Technology
Dr. H.S. Lalithamba
Tags
Summary
These notes cover electrode potential and cells. They detail the introduction to electrochemical processes, classification of electrochemical cells (Galvanic, Electrolytic, etc.), and the origin of single electrode potential.
Full Transcript
Engg. Chemistry, I/II Sem Dr. H.S. Lalithamba ELECTRODE POTENTIAL AND CELLS (UNIT-1) For 2021-22 Onwards Introduction: The electrical energy is a form of...
Engg. Chemistry, I/II Sem Dr. H.S. Lalithamba ELECTRODE POTENTIAL AND CELLS (UNIT-1) For 2021-22 Onwards Introduction: The electrical energy is a form of energy that has tremendous practical significance to us on a day-to-day basis. A day without electricity from either the power corporation or batteries is unimaginable in our technological society. Electrochemistry is an area of chemistry that deals with the inter conversion of electrical energy and chemical energy. Electrochemical processes are redox reactions in which the energy released by a spontaneous reaction is converted into electricity. Therefore, understanding of the fundamental principles and applications of electrochemical cells and the thermodynamics of electrochemical reactions is highly desirable. In oxidation, a species loses one or more electrons resulting in an increase in the oxidation number. M ↔ Mn+ + n e− -------------------------- Oxidation M +ne ↔M n+ − ------------------Reduction Electrochemical cells: The basic apparatus used to the investigation of reactions in solution in terms of electrical measurements is an electrochemical cell. A cell consists of two electrodes, or metallic conductors, in contact with an electrolyte. The electrolyte is an ionic conductor which may be a solution, a liquid or a solid. The two electrodes may share the same electrolyte in which case the electrode compartment will be one. If the two electrodes are in contact with an electrolyte each (i.e. the electrolytes are different), the two compartments are joined by a salt bridge. Classification of electrochemical cells: The electrochemical cells are classified into two principal types, namely, (i) Galvanic cell and (ii) Electrolytic cell. Galvanic cell: A galvanic cell also called a voltaic cell is an electrochemical cell that produces electricity as a result of the spontaneous redox reactions occurring inside it. Galvanic cells are further classified as, a) Primary cells b) Secondary cells c) Concentration cells Primary (non rechargeable) cells: The cells in which the cell reaction is not completely reversible are referred to as primary cells. They are not capable of being easily recharged electrically and, hence are discarded after they are discharged. e.g., Dry cell 1 Engg. Chemistry, I/II Sem Dr. H.S. Lalithamba Secondary (rechargeable) cells: The cells in which the cell reaction is reversible are termed as secondary cells. They are rechargeable. Secondary cells after discharge can be recharged electrically to their original condition by passing current through them in the opposite direction to that of discharge current. These are storage devices for electrical energy and referred to as storage batteries or accumulators. e.g., Lead-acid battery, Ni-Cd battery Concentration cells: The galvanic cells consisting of both anode and cathode made up of the same element (metal or non metal) in contact with the solutions of the same electrolyte of different concentrations are referred to as concentration cells. E.g., A concentration cell consisting of copper electrodes dipped in CuSO4 solutions of different concentrations is represented as, Cu(s)|CuSO4(C1) || CuSO4(C2)|Cu(s) Electrolytic cells: An electrochemical cell in which a non spontaneous reaction is driven by an external source of current is called an electrolytic cell. Electrolytic cells have a common electrolyte and no salt bridge. e.g., all secondary cells during charging act as electrolytic cells. Single electrode potential (E): The potential that is developed when an element (metal or non-metal) is in equilibrium with its own ions in solution is called single electrode potential or half-cell potential. Origin of single electrode potential (E): When a metal ‘M’ is dipped in a solution containing its own ions, Mn+, exhibits the following two tendencies. i) The metal has a tendency to pass into solution as positively charged metal ions. M → Mn+ + n e− ii) Simultaneously, ions from the solution tend to discharge on to the metal. Mn+ → MMn+ Due to these tendencies, a potential difference arises and the origin of the potential can be explained as follows. 1) If the concentration of metal ions in solution is very low, the metal tends to pass into solution as positively charged metal ions leaving behind the electrons. This makes the metal negatively charged. As the negative charge on the metal builds up, it becomes more difficult for the metal ions to leave the metal. The metal ions that have left the metal cause a positive charge to accumulate in the solution. This positive charge in the solution tends to collect near the negative charge on the metal. Thus an electric double layer, also known as Helmholtz double layer (HDL) is built up at the electrode-metal ion interface as depicted in the figure (a). 2 Engg. Chemistry, I/II Sem Dr. H.S. Lalithamba The electrical double layer is like a capacitor with oppositely charged plates. A potential difference exists across the electrical double layer and is referred to as single electrode potential. 2) When the concentration of metal ions in solution is high, the metal ions present in the solution, tend to get deposited on the metal surface rendering it positively charged. This positively charged layer attracts the negatively charged anions present in the solution towards it. Thus, the anions collect at the surface as a layer of negative charges and an electrical double layer is built up as depicted in figure (b). The potential difference that exists across this electrical double layer is called single electrode potential. Standard electrode potential (Eo): The potential that is developed when an element (metal or non-metal) is in equilibrium with a solution containing its own ions of unit concentration (1M) at 298K is called standard electrode potential. If the electrode system involves gaseous substances, they must be passed at a partial pressure of one atmosphere. 3 Engg. Chemistry, I/II Sem Dr. H.S. Lalithamba Derivation of Nernst equation for single electrode potential: Nernst equation is a thermodynamic equation which relates the single electrode potential (E) with standard electrode potential (Eo), concentration of metal ions [Mn+] in solution and temperature (T). It is derived using the concept of free energy (G). Consider a metal ion-metal electrode of the type, Mn+ + ne− M The maximum work available from a reversible chemical process is equal to the maximum amount of electrical energy that can be obtained. Wmax = Wele Electrical energy in this case is the product of the electrode potential (volts) and the total electric charge (coulombs) that passes through the electrode. Electrical energy = volts x coulombs The total charge is determined by the number of moles of electrons (n) that pass through the circuit. By definition, Total charge = n F where F, the Faraday is the electrical charge contained in 1 mole of electrons. Wmax = Wele = - nFE E is the electrode potential The negative sign indicates that the electrical work is done by the system on the surroundings. Wmax = G. The free energy change due to the transfer of 1 mole of ions across the double layer is related to the electrode potential (E). G = - nFE --------------- (1) Both n and F are positive quantities and G is negative for a spontaneous process, so E is positive. For reactions in which the reactants and products are in their standard states, equation (1) becomes Go = - nFEo ---------------- (2) o E is positive for spontaneous process. G is related to Go and the equilibrium constant, Kc by the expression, G = Go + RT lnKc ------------ (3) - nFE = - nFEo + RT lnKc --------- (4) [reduced form] [M] Since the equilibrium constant, Kc = [oxidised form] = [Mn+], equation (4) becomes, - nFE = - nFEo + RT ln [M] ------ (5) [Mn+] Dividing equation (5) by - nF, we get E = Eo - RT ln [M] nF [Mn+] But [M] = 1 E = Eo - RT ln 1 nF [Mn+] E = Eo + RT ln[Mn+] -------- Basic Nernst equation nF or E = Eo + 2.303 RT log10[Mn+] nF 4 Engg. Chemistry, I/II Sem Dr. H.S. Lalithamba By substituting the values for the universal gas constant, R (8.314 JK−1mol−1), Faraday, F (96,500 JV−1mol−1) and putting the temperature (T) equal to 298K, the above equation further reduces to, E = Eo + 0.0591 log10[Mn+] n Effect of metal ion concentration and temperature on single electrode potential: Since both the metal ion concentration and temperature terms are found in the numerator of the Nernst equation, E = Eo + RT ln[Mn+], the single electrode potential is directly nF proportional to the metal ion concentration and temperature. Construction of a cell: A galvanic cell is formed when two electrodes in contact with suitable electrolytes, are connected externally by a conducting material, i.e. a metallic wire and internally by means of a salt bridge and thereby produce an electric current. When two different electrolyte solutions are in contact in a cell, such a liquid-liquid contact introduces an additional potential known as liquid junction potential (across the interface of the two electrolytes). As a result, the observed emf will be more than the actual emf of the cell. The contribution of liquid junction potential to the emf of the cell can be reduced by joining the electrolyte compartments through a salt bridge. The success of the salt bridge lies in the fact that the liquid-liquid junction potentials at either end are largely independent of the concentration of the two dilute solutions, so they nearly cancel. Electrodes and Electrode reactions: The representation of a Daniel cell and the electrode reactions are depicted below. Zn(s)ZnSO4(aq)║CuSO4(aq)Cu(s) Anode: The electrode at which oxidation occurs is called the anode. Cathode: The electrode at which reduction takes place is the cathode. At anode, the metal passes into solution as metallic ions with the release of electrons. At cathode, the metal ions present in the solution get deposited on the metal surface. These ions gain the electrons that are released at the anode and are conducted to the cathode through the external circuit and get reduced to metal atoms. At anode : Zn(s) → Zn++(aq) + 2e− (oxidation) At cathode: Cu (aq) + 2e → Cu(s) ++ − (reduction) Overall reaction: Zn(s) + Cu (aq) → Zn (aq) + Cu(s) ++ ++ 5 Engg. Chemistry, I/II Sem Dr. H.S. Lalithamba In a galvanic cell, the cathode has a higher potential than the anode. The species undergoing reduction withdraws electrons from its electrode (cathode), so leaving a relative positive charge on it (corresponding to a high potential). At the anode, oxidation results in the transfer of electrons to the electrode, so giving a relative negative charge (corresponding to a low potential). During the operation of a galvanic or voltaic cell, a chemical reaction occurs at each electrode as described above and it is the energy of these reaction which provide the electrical energy of the cell. In many cells, there is a overall chemical reaction and in such cases the energy of the overall reaction gets converted into electrical energy. EMF of a cell (emf or Ecell): The work that given transfer of electrons can accomplish in a cell depends on the potential difference between the two electrodes and such a potential difference is called the cell potential. It is represented as ‘EMF’ or ‘emf’ or ‘Ecell’ or cell potential. It is expressed in volts. The cell potential is said to be large, when a given number of electrons travelling between the electrodes can do a large amount of work. When the cell potential is small, the same number of electrons can do only a small amount of work. The cell potential becomes zero, when the overall cell reaction is at equilibrium and subsequently the cell can do no work. EMF of a cell is the difference in the single electrode potentials of the two electrodes constituting the cell. Ecell = Ecathode- Eanode or Ecell = Eright electrode - Eleft electrode Eo cell = Eo cathode- Eo anode Ecell = E o cell + 0.0591 log10 [Products] n [Reactants] Measurement of single electrode potential: The single electrode potential of an electrode cannot be determined directly. To determine the single electrode potential, the electrode under examination is coupled to a standard reference electrode, say calomel electrode. E.g. Zn(s) | Zn++(aq) || KCl(sat),Hg2Cl2(sat) | Hg(l), where Zn(s) | Zn++(aq) is the electrode whose potential is to be determined. We know that, Ecell = Ecathode- Eanode or Ex = Ecalomel- Ezn++|Zn Since Ex and Ecalomel are known, Ezn++|Zn can be easily evaluated. Ezn++|Zn = Ecalomel - Ex Cell notation and cell conventions Notation: (i) The phase boundaries, i.e. the interface between the metal and the solution containing its own ions, are denoted by a vertical line. (ii) A double vertical line represents the salt bridge. (iii) An arrow mark is used to indicate the flow of electrons from one electrode to other. 6 Engg. Chemistry, I/II Sem Dr. H.S. Lalithamba The Daniel cell in which the liquid junction potential is assumed to be eliminated is denoted as, → Zn(s) | ZnSO4(aq) || CuSO4(aq) | Cu(s) A liquid junction is denoted by so the Daniel cell is written as, Zn(s) | ZnSO4(aq) CuSO4(aq) | Cu(s) Conventions: (i) The electrode at which oxidation takes place i.e. anode is always written on the left side while the electrode at which reduction occurs i.e. cathode is written on the right side. (ii) The electrode potential always refers to reduction potential and is represented as EMn+|M. Sign conventions: 1) Electrode potential : In a cell, oxidation takes place at anode and reduction at cathode. Accordingly, the potential at anode is called oxidation potential and that at cathode is called reduction potential. As per convention, the electrode potential is generally expressed as reduction potential. The reduction potential of anode can be obtained by simply reversing the sign of its oxidation potential. e.g., Oxidation potential of Zn|Zn++ = + 0.76 V Reduction potential of Zn++|Zn = - 0.76 V The following latest convention has been followed in respect of sign conventions for electrode potential. i) The electrode at which reduction occurs with respect to standard hydrogen electrode has positive sign for reduction potential or assigned negative sign for oxidation potential. The potential of the electrode Mn+|M is a measure of tendency of the metal ions to get discharged, i.e. for the ions to be reduced. ii) The electrode at which oxidation occurs with respect tot standard hydrogen electrode has a positive sign for oxidation potential or negative sign for the reduction potential. The potential of the electrode M|Mn+ represents the tendency for the metal to pass into solution as ions, i.e. for the metal atoms to be oxidised. 2) EMF: The emf of a cell is assigned a positive sign when the left hand electrode acts as a source of electrons (i.e. releases electrons by undergoing oxidation), while the right hand electrode removes them with the redox reaction being spontaneous in the forward direction (i.e. metal ions gain electrons and there by get reduced). If the emf of a cell is negative, the reverse is true. Concentration cells: The galvanic cells consisting of both anode and cathode made up of the same metal in contact with the solutions of the same electrolyte of different concentrations are referred to as concentration cells. A concentration cell consisting of the electrodes of the same metal, M dipped in solutions of their own ions, Mn+ of concentrations C1 and C2 is represented as, M|Mn+(C1)|| Mn+(C2)|M Anode Cathode In a concentration cell, the electrode at which the metal ion concentration is low compared to the other would become an anode while the other electrode at which the metal ion 7 Engg. Chemistry, I/II Sem Dr. H.S. Lalithamba concentration is high would act as a cathode. It is apparent that the electrode potential depends on the concentrations of the ions used. E.g, A concentration cell consisting of copper electrodes dipped in CuSO4 solutions of different concentrations is represented as, Cu(s)|CuSO4(C1)||CuSO4(C2)|Cu(s) Consider a case of copper electrodes put into two aqueous solutions of CuSO4 at 0.1M and 1.0M concentrations. The two solutions are connected by a salt bridge, and the electrodes are connected by a wire. According to Le Chatlier’s principle, the tendency for the reduction Cu++(aq) + 2 e− → Cu(s) increases with increasing concentration of Cu++ ions. Therefore, reduction should occur in the more concentrated compartment (cathode) and oxidation should take place on the more dilute side (anode). The cell notation is Cu(s)|CuSO4(0.1M)||CuSO4(1.0M)|Cu(s) Anode Cathode Half-cell reactions : At anode : Cu → Cu++ (0.1M) + 2e− At cathode : Cu++ (1.0M) + 2 e− → Cu Net cell reaction: Cu++ (1.0M) → Cu++ (0.1M) EMF of a concentration cell: The emf of a concentration cell, M | Mn+(C1) || Mn+(C2) | M is given by, Ecell = Ecathode - Eanode = (Eo + 0.0591 log10C2) - (Eo + 0.0591 log10C1) n n Ecell = 0.0591 log10C2 n C1 When C1> C2, Ecell is negative. When C2> C1, Ecell is positive. When C1=C2, Ecell is zero. Concentration cells with transference and without transference: Concentration cells with transference: In a concentration cell where the two solutions connected through a salt bridge. Such a cell is called a concentration cell with transference. 8 Engg. Chemistry, I/II Sem Dr. H.S. Lalithamba Ex: A concentration cell consisting of copper electrodes dipped in CuSO4 solutions of different concentrations. Concentration cells without transference: There are some concentration cells where the two half-cells are not connected through a salt bridge. They are connected through solutions of same electrolyte of equal concentration but contain different quantities of metal electrodes dissolved in mercury. Such cells are called concentration cells without transference. A typical example of concentration cell without transference is the Weston cell. It is represented using the cell notation, Cd (Hg), [Cd] = C1 | CdSO4 (aq) | Cd (Hg), [Cd] = C2 Significance of concentration cell: Membrane potentials, the potentials established across the membrane of a biological cell due to unequal concentrations of the same ions can be calculated by treating the cell as a concentration cell. Types of electrodes: The electrodes constituting a reversible cell must themselves be reversible. Several types of such electrodes are known. They are, 1. Electrodes of the first kind 2. Electrodes of the second kind Electrodes of the first kind or metal-metal ion electrodes: The electrodes of this kind consist of an element (metal or non-metal) in contact with a solution of its own ions. e.g. Zn in ZnSO4 solution, Cu in CuSO4 solution, Hydrogen in solution containing H+ ions, Oxygen in solution containing OH− ions. The electrodes of this type are reversible w.r.t. ions of the electrode material. Electrodes of the second kind or metal-metal insoluble salt electrodes: The electrodes of this kind consist of a metal and a sparingly soluble salt of the metal in contact with a solution of a soluble salt of the same anion. e.g. Calomel electrode, Hg(l)|Hg2Cl2(s), KCl(solution) Ag(s)|AgCl(s); KCl(solution) The electrodes of this type are reversible w.r.t. a common anion, i.e., the chloride ion in the above electrodes. Electrodes of second kind are of great value in electrochemistry, because they permit the ready establishment of an electrode reversible with respect to anions. Reference electrodes: The electrodes of standard potentials with which the potentials of other electrodes can be computed are known as reference electrodes. e.g. Standard hydrogen electrode (primary), calomel electrode (secondary). 9 Engg. Chemistry, I/II Sem Dr. H.S. Lalithamba Limitations or drawbacks of using hydrogen electrode as a reference electrode: 1. It is difficult to maintain hydrogen ion concentration always unity. 2. It is difficult to pass hydrogen gas always at 1 atmosphere. 3. The deposition of impurities on platinum foil causes hindrance in maintaining the equilibrium between the gas and H+ ions. 4. Hydrogen electrode cannot be used in solutions containing oxidising agents such as nitrates, permanganates, ferric salts, etc. Calomel electrode: Calomel electrode is a secondary reference electrode. Pt wire KCl Solution (sat.) Hg A paste of pure Hg + Hg2Cl2 + KCl Highly pure Hg Calomel electrode consists of a long glass tube having two side tubes as depicted in figure. Highly pure mercury is placed at the bottom of the tube and is covered by a paste of pure mercury, calomel (Hg2Cl2) and potassium chloride solution. The electrical connection is made by means of platinum wire sealed into another small glass tube having little mercury at its bottom. The rest of the tube is filled with an appropriate concentration of KCl solution saturated with calomel. The calomel electrode can be connected to another electrode to make the cell complete by means of a salt bridge. Calomel electrode can be represented as, Hg|Hg2Cl2(sat), KCl(solution) The reaction that occurs at calomel electrode is, At anode : Hg(l) + Cl−(aq) ½ Hg2Cl2(s) + e- ½ Hg2Cl2(s) + e− Hg(l) + Cl−(aq) The electrode potential of calomel electrode depends on the concentration of KCl solution with which the tube is filled. The reduction potentials for the various KCl concentration at 25oC are, 0.1M KCl solution 0.3338V 1.0M KCl solution 0.281V Saturated KCl solution 0.2415V Advantages of calomel electrode: 1. It is simple to construct and operate. 2. It gives reproducible electrode potentials. 10 Engg. Chemistry, I/II Sem Dr. H.S. Lalithamba Silver-silver chloride electrode: In recent times, silver-silver chloride electrode has been frequently employed as a reference electrode for the accurate determination of standard electrode potentials. Since it is a reversible electrode, it can be used to determine the Eo values by the use of cells which contain chlorides and are free from liquid junction potentials. Silver-silver chloride electrode is constructed as follows. Pt wire KCl solution (sat.) AgCl Ag Pt wire A small sheet or a short foil of platinum is first coated with silver by electrolysis of an argentocyanide solution. The silver is then partly converted into silver chloride by making it an anode in KCl solution and by passing a current of low density for 30 minutes. Finally it is sealed to a platinum wire and dipped in KCl or HCl solution in a glass tube. The electrode is reversible with respect to chloride ions. The silver-silver chloride electrode is represented as, Ag(s) | AgCl(s), KCl(aq). The reaction that occurs at silver-silver chloride electrode is, At anode: Ag(s) + Cl−(aq) AgCl(s) + e− At cathode: AgCl(s) + e− Ag(s) + Cl−(aq) The potential of Ag-AgCl electrode is 0.2224V at 25oC (1M KCl) The potential of Ag-AgCl electrode is 0.199V at 25oC (saturated KCl) Ion selective electrodes: The electrodes that are able to respond to certain specific ions only and develop a potential while ignoring the other ions in a solution are called ion selective electrodes. e.g., Glass electrode Glass electrode: Glass electrode is an ion selective electrode which responds to hydrogen ions in a solution and develop potential while ignoring the other ions. One of the most advances in the recent years in connection with the determination of pH is the use of glass electrode. 11 Engg. Chemistry, I/II Sem Dr. H.S. Lalithamba Pt wire Ag / AgCl 0.1M HCl The glass electrode consists of a glass tube having a small bulb at its bottom. The bulb is made up of a special type of thin walled glass of relatively low melting point and high electrical conductivity. The bulb contains a solution of a buffer or 0.1M hydrochloric acid solution. Electrical connection is made by a platinum wire coated with Ag-AgCl and is dipped in a buffer solution or hydrochloric acid solution. Glass electrode can be represented as, Ag | AgCl(s), HCl(0.1M) | glass The reaction that occurs at glass electrode is not clearly understood. The probable reaction is, NaG + H+ HG + Na+ (on the surface ( in solution) of glass membrane) Limitations of glass electrode: 1) Glass electrode cannot give satisfactory results with the solutions having pH greater than 9. As the pH increases, alkalinity of the solution increases leading to increase in salt effects. 2) In very acidic solutions of pH