DR ALIM'S AUTOMATION PDF
Document Details
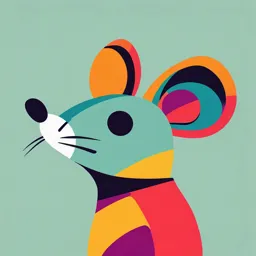
Uploaded by SelfSufficientFlashback
Ahmadu Bello University
Dr. ALIM
Tags
Summary
This document, "DR ALIM'S AUTOMATION" is an introduction to automation, defining it as the process of completing a task with minimal human intervention using specialized equipment, devices, and techniques, to observe, decide, and control the manufacturing processes. It traces the history of automation from its early mechanical beginnings through to its modern computer-controlled forms, along with contrasting mechanization and automation. Key aspects covered include the benefits and social impact of automation, along with lower cost automation solutions.
Full Transcript
CHAPTER 1 AUTOMATION INTRODUCTION The word automation comes from the Greek word “automatos,” meaning self-acting. The word automation was coined in the mid-1940s by the U.S. automobile industry to indicate the automatic handl...
CHAPTER 1 AUTOMATION INTRODUCTION The word automation comes from the Greek word “automatos,” meaning self-acting. The word automation was coined in the mid-1940s by the U.S. automobile industry to indicate the automatic handling of parts between production machines, together with their continuous processing at the machines. The advances in computers and control systems have extended the definition of automation. By the middle of the 20th century, automation had existed for many years on a small scale, using mechanical devices to automate the production of simply shaped items. However the concept only became truly practical with the addition of the computer, whose flexibility allowed it to drive almost any sort of task. DEFINITION OF AUTOMATION Automation can generally be defined as the process of following a predetermined sequence of operations with little or no human labor, using specialized equipment and devices that perform and control manufacturing processes. Automation in its full sense, is achieved through the use of a variety of devices, sensors, actuators, techniques, and equipment that are capable of observing the manufacturing process, making decisions concerning the changes that need to be made in the operation, and controlling all aspects of it. OR Automation is the process in industry where various production operations are converted from a manual process, to an automated or mechanized process. Example: Let’s assume that a worker is operating a metal lathe. The worker collects the stock, already cut to size, from a bin. He then places it in the lathe chuck, and moves the various hand-wheels on the machine to create a component; a bolt could be such an item. When finished the worker begins the process again to make another item. This would be a manual process. If this process were automated, the worker would place long lengths of bar into the feed mechanism of an automatic lathe. The lathe mechanisms feed the material into the chuck, turn the piece to the correct shape and size, and cut it off the bar before beginning another item. This is an example of an automated machine in a manufacturing process. Automation is a step beyond mechanization, where human operators are provided with machinery to help them in their jobs. Industrial robotics are said to be the most visible part of automation. Modern automated processes are mostly controlled by computer programs, which through the action of sensors and actuators, monitor progress and control the sequences of events until the process is complete. Decisions made by the computer ensure that the process is completed accurately and quickly. Many people fear that automation will result in layoffs and unemployment; they believe that its evils considerably outweigh its benefits. Basically, automation does take over jobs performed by workers; but automation does not need to bring about unemployment, as some people fear, for three very positive reasons: First, in terms of the numbers of workers required to produce a product, the reduction is a temporary displacement which can be offset by the demands of a broadening market, as well as the creation of new industries. It still takes many workers to build, service, and operate any automatic machine. Second, automation does not happen overnight; it is an evolutionary process. Manual, direct-labor work will be progressively transformed into work, which will be cleaner, easier, safer, and more rewarding to the worker, who, through the process of automation itself, will be trained for the more skillful accomplishments required in the better jobs of the future. Third, and most important, automation is the necessary solution to a predicted shortage of labor. It is designed to do the work of people who are not there; it is a solution to a problem, not a cause. Automation is a technology dealing with the application of mechatronics and computers for production of goods and services. Manufacturing automation deals with the production of goods. It includes: Automatic machine tools to process parts. Automatic assembly machines. Industrial robots. Automatic material handling. Automated storage and retrieval systems. Automatic inspection systems. Feedback control systems. Computer systems for automatically transforming designs into parts. Computer systems for planning and decision making to support manufacturing. The decision to automate a new or existing facility requires the following considerations to be taken into account: Type of product manufactured. Quantity and the rate of production required. Particular phase of the manufacturing operation to be automated. Level of skill in the available workforce. Reliability and maintenance problems that may be associated with automated systems. Economics. MECHANIZATION VS AUTOMATION Mechanization refers to the use of powered machinery to help a human operator in some task. The use of hand-powered tools is not an example of mechanization. The term is most often used in industry. The addition of powered machine tools; such as the steam powered lathe dramatically reduced the amount of time needed to carry out various tasks, and improves productivity. Today very little construction of any sort is carried out with hand tools. Automation and mechanization are often confused with each other, though it should not be too hard to keep them apart. Mechanization saves the use of human muscles; automation saves the use of human judgment. Mechanization displaces physical labor, whereas automation displaces mental labor. Mechanization is the replacement of human power by machine power. Mechanization often replaces craftwork and creates jobs for unskilled labor. It also only affects one or two industries at a time. Mechanization moves slowly and the job displacement is short-term. Mechanization is what occurred during the industrial revolution. Automation is the replacement of human thinking with computers and machines. Automation tends to create jobs for skilled workers at the expense of unskilled and semi-skilled workers. It affects many industries at the same time, moving rapidly. It also creates longer-term job displacement and has been more characteristic since the 1950s. ADVANTAGES OF AUTOMATION Manufacturing companies in virtually every industry are achieving rapid increases in productivity by taking advantage of automation technologies. When one thinks of automation in manufacturing, robots usually come to mind. The automotive industry was the early adopter of robotics, using these automated machines for material handling, processing operations, and assembly and inspection. Automation can be applied to manufacturing of all types. The advantages of automation are: Increase in productivity. Reduction in production costs. Minimization of human fatigue. Less floor area required. Reduced maintenance requirements. Better working conditions for workers. Effective control over production process. Improvement in quality of products. Reduction in accidents and hence safety for workers. Uniform components are produced. GOALS OF AUTOMATION Automation has certain primary goals as listed below: Integrate various aspects of manufacturing operations so as to improve the product quality and uniformity, minimize cycle times and effort, and thus reduce labor costs. Improve productivity by reducing manufacturing costs through better control of production. Parts are loaded, fed, and unloaded on machines more efficiently. Machines are used more effectively and production is organized more efficiently. Improve quality by employing more repeatable processes. Reduce human involvement, boredom, and possibility of human error. Reduce workpiece damage caused by manual handling of parts. Raise the level of safety for personnel, especially under hazardous working conditions. Economize on floor space in the manufacturing plant by arranging the machines, material movement, and related equipment more efficiently. SOCIAL ISSUES OF AUTOMATION Automation has contributed to modern industry in many ways. Automation raises several important social issues. Among them is automation’s impact on employment/unemployment. Automation leads to fuller employment. When automation was first introduced, it caused widespread fear. It was thought that the displacement of human workers by computerized systems would lead to unemployment (this also happened with mechanization, centuries earlier). In fact the opposite was true, the freeing up of the labor force allowed more people to enter information jobs, which are typically higher paying. One odd side effect of this shift is that “unskilled labor” now pays very well in most industrialized nations, because fewer people are available to fill such jobs leading to supply and demand issues. Some argue the reverse, at least in the long term. First, automation has only just begun and short-term conditions might partially obscure its long- term impact. For instance many manufacturing jobs left the United States during the early 1990s, but a massive up scaling of IT jobs at the same time offset this as a whole. Currently, for manufacturing companies, the purpose of automation has shifted from increasing productivity and reducing costs to increasing quality and flexibility in the manufacturing process. Another important social issue of automation is better working conditions. The automated plants needs controlled temperature, humidity, and dust free environment for proper functioning of automated machines. Thus the workers get a very good environment to work in. Automation leads to safety of workers. By automating the loading and unloading operations, the chances of accidents to the workers get reduced. Workers expect an increase in standard of living with the help of automation. Standards of living go up with the increase in productivity, and automation is the sure method of increasing productivity. The cost of color TVs, washing machines, and stereos has declined, thus enabling a large number of households to buy these products. LOW COST AUTOMATION Low cost automation (LCA) is a technology that creates some degree of automation around the existing equipment, tools, methods, people, etc. by using standard components available in the market with low investment, so that the payback period is short. The benefits of low cost automation are numerous. It not only simplifies the process, but also reduces the manual content without changing the basic set up. Major advantages of low cost automation are low investment, increased labor productivity, smaller batch size, better utilization of the material, and process consistency leading to less rejections. A wide range of activities such as loading, feeding, clamping, machining, welding, forming, gauging, assembly, and packing can be subjected to low cost automation systems adoption. Besides, low cost automation can be very useful for process industries manufacturing chemicals, oils, or pharmaceuticals. Many operations in food processing industries, which need to be carried out under totally hygienic conditions, can also be rendered easy through low cost automation systems. A wide variety of systems (mechanical, hydraulic, pneumatic, electrical, and electronics) are available for deployment in LCA systems. However, each has its own advantages as well as limitations. For uncomplicated situations, one can build a simple LCA device using any of the above systems, through a rapid techno-economic evaluation. However, in most of the practical applications, hybrid systems are used because that can allow use of the advantages of different devices, while simultaneously minimizing individual disadvantages. Issues in Low Cost Automation 1. Assessment of the Current Productivity Level: There are some simple procedures for this. Work sampling (activity sampling) is one of them. It needs no equipment and little time to collect the data. If the data is processed, considerable information will come out about the current productivity level. 2. PMTS: Predetermined Motion and Time Studies is a very useful tool to check whether an existing manual operation is correctly pasted. If the time taken is more than desirable, PMTS will help in identifying it and improving it. 3. Design for Automation and Assembly: When components are made and assembled manually one may not have thought about the complexity of automation. For example, putting together half a dozen nuts and bolts is very easy in a manual assembly but very complex for an automatic system. TYPES OF AUTOMATION Fixed Automation (Hard Automation) Fixed automation refers to the use of special purpose equipment to automate a fixed sequence of processing or assembly operations. It is typically associated with high production rates and it is relatively difficult to accommodate changes in the product design. This is also called hard automation. For example, GE manufactures approximately 2 billion light bulbs per year and uses fairly specialized, high-speed automation equipment. Fixed automation makes sense only when product designs are stable and product life cycles are long. Machines used in hard-automation applications are usually built on the building block, or modular principle. They are generally called transfer machines, and consist of the following two major components: powerhead production units and transfer mechanisms. Advantages Maximum efficiency. Low unit cost. Automated material handling—fast and efficient movement of parts. Very little waste in production. Disadvantages Large initial investment. Inflexible in accommodating product variety. Programmable Automation In programmable automation, the equipment is designed to accommodate a specific class of product changes and the processing or assembly operations can be changed by modifying the control program. It is particularly suited to “batch production,” or the manufacture of a product in medium lot sizes (generally at regular intervals). The example of this kind of automation is the CNC lathe that produces a specific product in a certain product class according to the “input program.” In programmable automation, reconfiguring the system for a new product is time consuming because it involves reprogramming and set up for the machines, and new fixtures and tools. Examples include numerically controlled machines, industrial robots, etc. Advantages Flexibility to deal with variations and changes in product. Low unit cost for large batches. Disadvantages New product requires long set up time. High unit cost relative to fixed automation. Flexible Automation (Soft Automation) In flexible automation, the equipment is designed to manufacture a variety of products or parts and very little time is spent on changing from one product to another. Thus, a flexible manufacturing system can be used to manufacture various combinations of products according to any specified schedule. With a flexible automation system, it is possible to quickly incorporate changes in the product (which may be redesigned in reaction to changing market conditions and to consumer feedback) or to quickly introduce a new product line. For example, Honda is widely credited with using flexible automation technology to introduce 113 changes to its line of motorcycle products in the 1970s. Flexible automation gives the manufacturer the ability to produce multiple products cheaply in combination than separately. Advantages Flexibility to deal with product design variations. Customized products. Disadvantages Large initial investment. High unit cost relative to fixed or programmable automation. CURRENT EMPHASIS IN AUTOMATION Currently, for manufacturing companies, the purpose of automation has shifted from increasing productivity and reducing costs, to broader issues, such as increasing quality and flexibility in the manufacturing process. The old focus on using automation simply to increase productivity and reduce costs was short-sighted, because it is also necessary to provide a skilled workforce who can make repairs and manage the machinery. Moreover, the initial costs of automation were high and often could not be recovered by the time entirely new manufacturing processes replaced the old. (Japan’s “robot junkyards” were once world famous in the manufacturing industry.) Automation is now often applied primarily to increase quality in the manufacturing process, where automation can increase quality substantially. For example, automobile and truck pistons used to be installed into engines manually. This is rapidly being transitioned to automated machine installation, because the error rate for manual installment was around 1– 1.5%, but is 0.00001% with automation. Hazardous operations, such as oil refining, the manufacturing of industrial chemicals, and all forms of metal working, were always early contenders for automation. Another major shift in automation is the increased emphasis on flexibility and convertibility in the manufacturing process. Manufacturers are increasingly demanding the ability to easily switch from one manufacturing product to other without having to completely rebuild the production lines. REASONS FOR AUTOMATION 1. Shortage of labor 2. High cost of labor 3. Increased productivity: Higher production output per hour of labor input is possible with automation than with manual operations. Productivity is the single most important factor in determining a nation’s standard of living. If the value of output per hour goes up, the overall income levels go up. 4. Competition: The ultimate goal of a company is to increase profits. However, there are other goals that are harder to measure. Automation may result in lower prices, superior products, better labor relations, and a better company image. 5. Safety: Automation allows the employee to assume a supervisory role instead of being directly involved in the manufacturing task. For example, die casting is hot and dangerous and the work pieces are often very heavy. Welding, spray painting, and other operations can be a health hazard. Machines can do these jobs more precisely and achieve better quality products. 6. Reducing manufacturing lead-time: Automation allows the manufacturer to respond quickly to the consumers needs. Second, flexible automation also allows companies to handle frequent design modifications. 7. Lower costs: In addition to cutting labor costs, automation may decrease the scrap rate and thus reduce the cost of raw materials. It also enables just-in-time manufacturing which in turn allows the manufacturer to reduce the in-process inventory. It is possible to improve the quality of the product at lower cost. REASONS FOR NO AUTOMATION 1. Labor resistance: People look at robots and manufacturing automation as a cause of unemployment. In reality, the use of robots increases productivity, makes the firm more competitive, and preserves jobs. But some jobs are lost. For example, Fiat reduced its work force from 138,000 to 72,000 in nine years by investing in robots. GM’s highly automated plant built in collaboration with Toyota in Fremont, California employs 3,100 workers in contrast to 5,100 at a comparable older GM plant. 2. Cost of upgraded labor: The routine monotonous tasks are the easiest to automate. The tasks that are difficult to automate are ones that require skill. Thus, manufacturing labor must be upgraded. 3. Initial investment: Cash flow considerations may make an investment in automation difficult even if the estimated rate of return is high. ISSUES FOR AUTOMATION IN FACTORY OPERATIONS Task is too difficult to automate. Short product lifecycle. Customized product. Fluctuating demand. Reduce risk of product failure. Cheap manual labor. STRATEGIES FOR AUTOMATION Specialization of operations. Combined operations. Simultaneous operations. Integration of operations. Increased flexibility. Improved material handling and storage. CHAPTER 12 TRANSFER DEVICES AND FEEDERS INTRODUCTION Since the beginning of the nineteenth century, the increasing need for finished goods in large quantities has led engineers to search for and to develop new methods of manufacturing or production. As a result of development in the various manufacturing processes, it is now possible to mass-produce high-quality durable goods at low cost. One of the most important manufacturing processes is the assembly process that is required when two or more components parts are to be secured together. The history of assembly process development is closely related to the history of the development of mass-production methods. The pioneers of mass production are also the pioneers of modern assembly techniques. Their ideas and concepts have brought significant improvement in the assembly methods employed in high volume production. However, many aspects of manufacturing engineering, especially the parts fabrication process, have been revolutionized by the application of automation, the technology of the basic assembly process has failed to keep pace. Although, during the last few decades, efforts have been made to reduce assembly costs by the application of high speed automation and more recently by the use of assembly robots, success has been quite limited and many assembly workers are still using the same basic tools as those employed at the time of the industrial revolution. So, in these days, it is necessary that manufacturing engineers and designers must learn about automatic assembly. This in turn provides means to improve design, productivity, and competitiveness. FUNDAMENTALS OF PRODUCTION LINES A production line consists of a series of workstations arranged so that the product moves from one station to the next, and at each location, a portion of the total work is performed on it. Production lines are associated with mass production. If quantities of the product are very high and the work can be divided into separate tasks that can be assigned to individual workstations, then a production line is the most appropriate manufacturing system. In terms of the capacity of a production line to cope with model variations, three types of line can be distinguished: Single-model line produces only one model and there is no variation in the model. The tasks performed at each station are the same on all product units. Batch-model line produces each model in batches. The workstations are set up to produce the desired quantity of the first model, and then the stations are reconfigured to produce the desired quantity of the next model, and so on. Mixed-model line also produces multiple models; however, the models are intermixed on the same line rather than being produced in batches. While a particular model is being worked on at one station, a different model is being processed at the next station. TYPES OF ASSEMBLY LINES There are two types of assembly lines: Manual assembly lines Automated assembly lines Manual Assembly Lines A manual assembly line consists of multiple workstations arranged sequentially, at which assembly operations are performed by human workers. Because all of the operations are in the control of the worker, so the tools required are simple and less expensive than those used in automated assembly. Manual assembly systems are best suited for low volume products that have high product variety. Processes accomplished on manual assembly lines include mechanical fastening operations, spot welding, hand soldering, and adhesive joining. Automated Assembly Lines The term automated assembly refers to the use of mechanized and automated devices to perform the various assembly tasks in an assembly line. Most automated assembly systems are designed to perform a fixed sequence of assembly steps on a specific product. An automated assembly line consists of automated workstations connected by a parts transfers system whose actuation is coordinated with the stations. In an ideal line, no human workers are on the line, except to perform auxiliary functions such as tool changing, loading and unloading parts, and repair and maintenance activities. Modern automated lines are integrated systems operating under computer control. Automated production lines are applied in processing operations as well as assembly. An automated assembly system performs a sequence of automated assembly operations to combine multiple components into a single entity. The single entity can be a final product or a subassembly in a larger product. In many cases, the assembled entity consists of a base part to which other components are attached. The components are joined one at a time (usually), so the assembly is completed progressively. A typical automated assembly system consists of the following subsystems: One or more workstations at which the assembly steps are accomplished. Parts feeding devices that deliver the individual components to the workstations. A work handling system for the assembled entity. REASONS FOR USING AUTOMATED ASSEMBLY LINES Automated assembly technology should be considered when the following conditions exist: High product demand: Automated assembly systems should be considered for products made in large quantity (in millions). Stable product design: In general, any change in the product design means a change in workstation tooling and possibly the sequence of assembly operations. Such changes can be very costly. The assembly consists of no more than a limited number of components. Automated production lines can be divided into two basics categories: (a) Transfer lines, which consist of a sequence of workstations that performs processing operations, with the automatic transfer of work units between stations. Transfer lines are usually expensive pieces of equipment; they are designed for a job requiring high quantities of parts. (b) Dial indexing machine, is a device used to convey parts for assembly, machining, packaging, finishing, or other manufacturing operations. In a dial indexing machine, the workstations are arranged around a circular worktable called a dial. The worktable is actuated by a mechanism that provides partial rotation of the table on each work cycle. TRANSFER SYSTEMS IN ASSEMBLY LINES The four main types of transfer systems are: Continuous transfer system Intermittent/synchronous transfer system Asynchronous transfer system Stationary transfer system In continuous transfer systems, the work carriers are moving at a constant speed while the work head index moves back and forth. Assembly operations are carried out during the period when the work heads are moving forward, keeping pace with the work carriers. Examples of continuous transfer systems can be seen in bottling operations, manual assembly where the worker can move with the moving line. In intermittent transfer, the work carriers are transferred intermittently and the work heads remain stationary. All the parts are moved at same time, hence, the term synchronous transfer system, which is also used to describe this method of workpiece transport. For example, intermittent transfer systems are used in machining operations, press-working operations, etc. This system is stressful to human workers, but good in automated operations. The asynchronous transfer system allows each work part to advance to the next station when processing at the current station has been completed. Each part moves independent of the other parts, increasing flexibility. This type of system is good for both manual and automated operations. In the stationary system, the part is placed in a fixed location where it remains during the entire assembly process. This system is used when the assembled product is bulky or difficult to handle, e.g., airplanes, ships, etc. AUTOMATIC MACHINES Automatic machines are those machines in which both the workpiece handling and the metal cutting operations are performed automatically. These machines have played an important role in increasing the production rate and have been in use for long time. In automatic machines, operations from feeding of stock to clamping, machining, and even inspection of the workpiece are carried out automatically. TRANSFER DEVICES/MACHINES A transfer machine is an automatic machine capable of performing many operations. It consists of many machine tools properly linked together. These are special purpose machines where the components are automatically transferred from one machining head to another. Operations are performed sequentially. Each machining head carries out one operation until the component reaches the end of the line and all the necessary operations have been performed. Transfer of the workpiece and its fixture from station to station is done automatically. Each station could be considered as simple work head with its own motor mounted on a base. It could also be defined as a combined material processing and material-handling machine. Transfer machines perform a variety of machining, inspecting, and quality control functions. They drill, mill, grind, as well as, control and inspect the operations. Transfer machines range from comparatively small units having only two to three workstations to long straight-line machines with more than 100 workstations. These machines are used primarily in automobile industry. A wide variety of transfer machines with different models, designs, and sizes are available in the market with salient features like automatic reset timer, sliding headstock, increased platen clamping speed, pressure adjustment system, and many more. Some of these machines are flexible, precise, fast, and some are used for high production jobs. They are used in many industrial sectors. There are different types of transfer machines available in the market like the CNC rotary transfer machine, economy precision transfer machine, rotary transfer machines for brass goods, automatic transfer line machine, transfer machines for production of valve bodies, six-station dial rotary transfer machines, and many more suiting different industrial purposes. SELECTION OF TRANSFER DEVICES Selection of a particular transfer device is based upon the following factors: Accuracy required in components. Various forces acting at stations on the components. Physical size of parts and production rate. Number of operations to be performed. Type of drive required, i.e., pneumatic, hydraulic, electric, or a combination of these. TRANSFER MECHANISM IN TRANSFER DEVICES Transfer mechanisms are used to move the workpiece from one station to another in the machine or from one machine to another to enable various operations to be performed on the part. Sensors and other devices usually control transfers of parts from station to station. Tools on transfer machines can be changed easily using tool holders with quick-change features. These machines may be equipped with various automatic gauging and inspection systems. These systems are utilized between operations to ensure that the dimensions of a part produced in one station are within acceptable tolerances before that part is transferred to the next station. The two transfer mechanisms used the are: Linear transfer mechanism Rotary transfer mechanism The goal is the same for both—put a blank, bar, casting, or forging in the first station and get a completely machined part at the other end. LINEAR TRANSFER MECHANISM Linear transfer systems provide a linear motion for workpart transfer in automated production systems. Linear transfer mechanisms are used for inline machines. Some of the commonly used linear transfer mechanisms are: Walking Beam System In this type of linear transfer mechanism (refer to Figure 12.1), the workpants are lifted up from their workstation locations by a transfer bar and moved one position ahead to the next station. The transfer bar then lowers the parts into nests that position them for processing at their stations. The beam then retracts to make ready for the next transfer cycle. A walking beam transfer system is used in transferring cylindrical parts, motor shafts, camshafts, crankshafts, tubing, and piping of all types. The advantages of walking beam machines include a lower machine chassis cost and significant reductions in fixturing costs. This system lends itself well to very high-speed machines. FIGURE 12.1 Walking Beam System. Powered Roller Conveyor System This type of system (refer to Figure 12.2) is used in automated flow lines. The conveyor can be used to move parts or pallets. Roller conveyors are flexible, robust, and highly efficient. FIGURE 12.2 Powered Roller Conveyor System. Chain Drive Conveyor System A chain or steel belt is used to transport the work carriers. The chain drive conveyor system shown in Figure 12.3 can be used for continuous, intermittent, or non-synchronous movement of work parts. FIGURE 12.3 Chain Drive Conveyor System. ROTARY TRANSFER MECHANISM In rotary transfer system, the workpieces are held in fixtures on a continuous rotating table. There are various methods used to index a circular table or dial at various equal angular positions corresponding to workstation locations. Some of the methods are described below: Rack and Pinion The rack and pinion mechanism is not suited for high-speed operation often associated with indexing machines. The device uses a piston to drive the rack, which causes the pinion gear and attached indexing table to rotate. Ratchet and Pawl A ratchet mechanism is based on a wheel that has teeth cut out of it and a pawl that follows as the wheel turns. Looking at Figure 12.4, as the ratchet wheel turns, the pawl falls into the dip between the teeth. The ratchet wheel can only turn in one direction (in this case anticlockwise). Its operation is simple but somewhat reliable, owing to wear and sticking of several of the components. FIGURE 12.4 Ratchet and Pawl System. Geneva Mechanism The Geneva-type mechanism has more general application in assembly machines but its cost is higher than the mechanisms described earlier. A Geneva mechanism as shown in Figure 12.5 is used to provide an intermittent rotational motion of the driven part while the driver wheel rotates continuously. If the driven member has eight slots for an eight-station dial indexing machine, each turn of the driver will cause the table to advance one-eighth of a turn. The driver only causes movement of the table through a portion of its rotation. FIGURE 12.5 Geneva Mechanism. Because the driven wheel in a Geneva motion is always under full control of the driver, there is no problem with overrunning. Impact is still a problem unless the slots of the driven wheel are accurately made and the driving pin enters these slots at the proper angle. The main characteristic of the Geneva mechanism is its restriction on the number of stops per revolution. The smaller the number of stops, the greater the adverse mechanical advantage between the driver and the driven members. This results in a high indexing velocity at the center of the indexing movement. CLASSIFICATION OF TRANSFER DEVICES Transfer devices/machines can be classified as: Inline transfer machines Rotary transfer machines The goal is the same for all, i.e., put a blank, bar, casting, or forging in the first station and get a completely machined part at the other end. Inline Transfer Machines The inline assembly machine consists of a series of automatic workstations located along an inline transfer system (refer to Figure 12.6). Inline transfer machines have a load station at one end and an unload station at the other end. The parts feeders, workstations, and inspection stations are arranged along the workflow. The components are transferred automatically from one machining station to another either by pulling supporting rails by means of an endless chain conveyor or by pushing along the continuous rails by air or hydraulic pressure. The components are loaded manually or automatically onto the central bed. All types of machining operations are carried out at various stations and the chips produced are removed so that these do not foul the working parts. FIGURE 12.6 Inline Transfer Machine. These machines are generally suitable for operations involving large workpieces and for those in which large workstations are required. Inline machines have the advantages of an unlimited number of workstations and efficient operator loading. In comparison with rotary transfer systems, the manufacturing costs of linear transfer systems are generally 10 to 20% higher according to type. Space requirements are greater as compared to the rotary transfer system. Inline transfer machines can be broadly classified as: Pallet type transfer machines Plain type transfer machines In pallet type transfer machines, the workpieces are transferred from stations by holding them in fixtures called pallets. In plain type transfer machines, the parts move in an unclamped position. Pallet type transfer machines are used for producing components requiring a high degree of accuracy. Rotary Transfer Machines In rotary transfer machines, the workpieces are located and clamped in pallet type fixtures that are indexed in a circular path as shown in Figure 12.7. The table rotates about a vertical axis and its movement could be continuous or intermittent. Rotary transfer machines automatically feed multiple workstations from a rotating turret. This combines an automated part feed with multiple simultaneous operations, streamlining the machining process significantly. The rotary transfer technology indexes a workpiece from station to station via a rotary table, with operations performed at each station. The number of stations allow for easier balance between long and short operation cycles. Because the tools rather than the workpiece are rotating, it is possible to insert machine stock of virtually any shape. This type of machining method permits the workpiece to be loaded and unloaded at a single location without interrupting the machining. FIGURE 12.7 Rotary Transfer Machine. Machining operations are typically hole-making operations (drilling, cross drilling, tapping, boring, counter boring, etc.) but can also include milling, turning, cutoff, broaching, crimping, threading, tapping, broachving, and other secondary machining and assembly operations. Although the rotary transfer machine is best suited for multi-million part runs, its flexibility also makes it effective for family of parts production. These machines are used when few machining stations need to be employed. Rotary arrangement saves floor space and presents a more compact arrangement. Rotary Index Table Rotary indexing tables are used to index/transfer parts and components in defined, angular increments so that they can be machined, worked, or assembled in multiple operations. Tables consist of a circular steel plate, one or more spindles, a drive system, and pins that hold parts and components in place. A rotary indexing table as shown in Figure 12.8 has either fixed or adjustable indexing angles. During each revolution, the table stops for a specified period of time so that an operation can be performed at each station. Rotary indexing tables are powered by pneumatic and electric motors, hydraulic drives, and manual actuation. Drive mechanisms can be located above, below, behind, or to the side of the table surface. Pneumatic rotary indexing tables are suitable for small and medium loads. Electrically powered tables are generally faster than pneumatic devices and can handle heavier loads. Tables that are powered by hydraulic drives use a pressurized fluid that transfers rotational kinetic energy. Manually actuated rotary indexing tables often include a hand crank or are loosened, turned, and adjusted by hand. FIGURE 12.8 Rotary Indexing Table. Besides boosting output, indexing tables improve safety. Hazardous operations can be placed on the opposite side of the table from the operator. A light curtain or safety glass installed above the table, along its diameter, will ensure that the hazardous operation and the operator remain separated. Applications for rotary indexing tables include assembly and equipment positioning as well as various automation, inspection, and machining applications. ADVANTAGES AND DISADVANTAGES OF TRANSFER MACHINES Advantages Transfer machines offer a number of advantages: Complex shaped components can be easily machined. Optimum utilization of floor space with these machines. Increased productivity. Components produced are of high quality, accurate, and cheap. Elimination of worker’s fatigue. Loading and unloading time is eliminated. Disadvantages High initial investment. These machines are limited to high production industries. A breakdown of one machine means stoppage of whole production line. Overhauling and maintenance costs are high. CONVEYOR SYSTEMS USED IN TRANSFER DEVICES A conveyor system is a common piece of material handling equipment that moves materials from one location to another. Conveyors work by two methods: through manual operation or through a power source. Conveyors are especially useful in applications involving the transportation of heavy or bulky materials. Conveyor systems allow quick and efficient transportation for a wide variety of materials, which makes them very popular in the material handling and packaging industries. Many kinds of conveying systems are available, and are used according to the various needs of different industries. By laying the conveyors along the line of production machines, the integration of material movement with the production processes is facilitated. The various types of conveyors used are: Roller conveyor Wheel conveyor Chute conveyor Belt conveyor Chain conveyor Magnetic belt conveyor Bucket conveyor (a) Roller Conveyor: Roller conveyors form an important means of conveying unit material in the industry. Usually, the load should possess a flat base, or should be mounted on a flat-bottomed pallet/container, so that it can roll on top of straight cylindrical rollers. These may be powered (or live) or non-powered (or gravity) roller conveyors. Gravity conveyors do not require a motor, but use wheels, rollers, and the pull of gravity to move materials along a conveyor. Power conveyors, unlike gravity conveyors, require a pneumatic or an electrical power source. Power is transmitted from the drive system to a drive pulley, which is fastened to the drive shaft. The drive pulley then transmits the power to the conveyor belt, which moves the conveyor bed, upon which the materials rest. Roller conveyors are shown in Figure 12.9. FIGURE 12.9 Roller Conveyor. (b) Wheel Conveyor: The wheel conveyor as shown in Figure 12.10 uses a series of skate wheels mounted on a shaft (or axle), where the spacing of the wheels is dependent on the load being transported. Slope for gravity movement depends on load weight. These are more economical than the roller conveyor. FIGURE 12.10 Wheel Conveyor. (c) Chute Conveyor: An inexpensive chute conveyor is used to link two handling devices. These are used to provide accumulation in shipping areas and to convey items between floors. These are inexpensive but have the limitation of difficulty to control position of the items. (Refer to Figure 12.11.) FIGURE 12.11 Chute Conveyor. (d) Belt Conveyor: Belt conveyors as shown in Figure 12.12 are used for the controlled movement of a large variety of both regular and irregular shaped products. They can move light, fragile to heavy, rugged unit loads on a horizontal, inclined, or declined path within the limits of product stability and the conveyor component capacities. The items being conveyed are carried by the top surface of the belt. FIGURE 12.12 Belt Conveyor. (e) Chain Conveyor: These conveyors offer high flexibility of layout and conditions of work. (Refer to Figure 12.13.) FIGURE 12.13 Chain Conveyor. (f) Magnetic Belt Conveyor: A magnetic belt conveyor is used to transport ferrous materials vertically, upside down, and around corners. (Refer to Figure 12.14.) FIGURE 12.14 Magnetic Conveyor. (g) Bucket Conveyor: Bucket conveyors are used to move bulk materials in a vertical or inclined path. Buckets are attached to a cable, chain, or belt. Buckets are automatically unloaded at the end of the conveyor run. (Refer to Figure 12.15.) FIGURE 12.15 Bucket Conveyor. FEEDERS A feeder is an extremely important element in a bulk material handling system, because it is the means by which the rate of solids flow from a hopper or bin is controlled. When a feeder stops, solids flow should cease. When a feeder is turned on, there should be a close correlation between its speed of operation and the rate of discharge of the bulk solid. Feeders feed material to conveyors, processors, and other equipment at a controlled rate to maximize efficiency and production. In essence, they are short conveyors that come in multiple shapes and sizes. Feeders are also used in recycling applications. Feeders differ from conveyors in that the latter are only capable of transporting material, not modulating the rate of flow. Dischargers are not feeders. Such devices are sometimes used to encourage material to flow from a bin, but they cannot control the rate at which material flows. This requires a feeder. CLASSIFICATION OF FEEDERS There are two basic types of feeders used in industrial plants: Volumetric feeders Gravimetric feeders As the name implies, a volumetric feeder modulates and controls the volumetric rate of discharge from a bin (e.g., cu.ft./hr.). The four most common types of such feeders are screw, belt, rotary valve, and vibrating pan. A gravimetric feeder, on the other hand, modulates the mass flow rate. This can be done either on a continuous basis (the feeder modulates the mass per unit time of material discharge) or on a batch basis (a certain mass of material is discharged and then the feeder shuts off). The two most common types of gravimetric feeders are loss-in-weight and weight belt. Gravimetric feeders should be used whenever there is a requirement for close control of material discharge. A gravimetric feeder should also be used when the bulk density of the material varies. CRITERIA FOR FEEDER SELECTION The feeder used (whether volumetric or gravimetric) should provide the following: Reliable and uninterrupted flow of material from some upstream device (typically a bin or hopper). The desired degree of control of discharge rate over the necessary range. Uniform withdrawal of material through the outlet of the upstream device. Interface with the upstream device such that load acting on the feeder from the upstream device is minimal. This minimizes the power required to operate the feeder, particle attrition, and abrasive wear of the feeder components. PARTS FEEDING DEVICES Parts feeding devices are the devices that deliver the individual components to the workstations. Some of these parts feeding devices are explained as follows: Hopper Chute Magazines Separator Parts feeder Feed track Escapement and placement device Selectors and orientor Ejectors and pushers Reel Cradle Bowls Pallets (a) Hopper: Hoppers are receptacles for the temporary storage of material. They are designed so that stored material can be dumped easily. This means that the parts are initially randomly oriented in the hopper. Hoppers can be made out of aluminum or steel for heavy-duty use, and plastic for light-duty applications. Hoppers are generally one of two types, bottom or tilt. Bottom hoppers are designed so that stored material can be dumped from the bottom of the hopper. Live bottom hoppers have hydraulically or mechanically driven screws to aid in discharging the material. They are typically used to aid in the discharge of sluggish or viscous materials. Some hoppers have a base that allows them to be lifted by forklifts for dumping. Important parameters to consider when specifying hoppers are the weight capacity, volume capacity, height, and weight of the hopper when empty. The weight capacity is the maximum weight the hopper is designed to hold. The volume capacity is the maximum volume of material the hopper is designed to hold. The height of the hopper is necessary to consider for applications where overhead space is limited. Common features for hoppers include agitation, perforations, self-dumping, side dumping, stackable, and wheels. A hopper will use agitation to dislodge material while dumping. Hoppers with agitation may also be called vibratory hoppers. Perforations in the hopper allow for the drainage of water or other liquids. Self- dumping hoppers have a mechanism to allow them to self-dump the material. Side dumping hoppers dump their contents from the side rather than the front. Stackable hoppers allow for greater economy of space, most are only to be stacked when empty, not when loaded. Some hoppers will come equipped with wheels for easy moving. (b) Chute: Chutes are smooth surfaced, inclined troughs that allow materials to move down the chute under the force of gravity. Chutes can be made of steel, plastic, or wood. Chutes may be straight or spiral. Chutes are designed to move materials without damage and convey objects from high-to-low levels under the force of gravity. (c) Magazines: Magazines are loading devices adopted to hold a pile of work of various shapes and sizes, for further loading into the machine. Magazines can be subdivided into flat (palettes) and chute magazines. Three different types of magazines are shown in Figure 12.16. Figure 12.16 A shows a flat magazine. Figure 12.16 B shows a chute magazine in zigzag form for the magazining of symmetrical cylindrical parts. Figure 12.16 C shows a chute magazine for plate parts. FIGURE 12.16 Types of Magazines. (d) Separator: Many times workpieces are to be fed into the machine at set intervals of time. So workpieces are separated by interrupting the flow of parts to machine. This can be done with the help of separators. (e) Parts Feeders: It is very costly to maintain part order throughout the manufacture cycle. For example, instead of keeping parts in pallets, they are often delivered in bags or boxes, where they must be picked out and sorted. A parts feeder is a machine that orients such parts before they are fed to an assembly station (refer to Figure 12.17). The most common type of parts feeder is the vibratory bowl feeder, where parts in a bowl are vibrated using a rotary motion, so that they climb a helical track. As they climb, a sequence of baffles and cutouts in the track create a mechanical “filter” that causes parts in all but one orientation to fall back into the bowl for another attempt at running the gauntlet. Other common devices use centrifugal forces, reciprocating forks, or belts to push parts through filters. These devices have the disadvantage that design and setup for new parts requires manual trial and error, which is slow and error-prone. Parts feeders provide a cost-effective alternative to manual labor, saving manufacturers valuable time and labor costs. One operator can oversee a number of automated machines, as opposed to one worker hand loading one machine. Hand selecting and inspecting is also time consuming and labor intensive. The tediousness of the process can subject the workers to repetitive motion injuries. Using vibratory feeders typically results in a better product, as well. When selecting a parts feeder, several factors must be taken into account, including the industry, application, material properties, and product volume. FIGURE 12.17 A Parts Feeder Orients Parts as They Arrive on the Left-hand Conveyor Belt. (f) Feed Track: A feed track is used to transfer the components from the hopper and part feeder to the exact location of the assembly workhead, maintaining proper orientation of the parts during the transfer. Feed tracks can be powered or gravity type. (g) Escapement and Placement Devices: The purpose of an escapement device is to remove components from the feed track at timed intervals that are consistent with the cycle time of the assembly workhead. The placement device physically places the component in the correct location at the workstations for the assembly operation by the worker. (Refer to Figure 12.18.) FIGURE 12.18 Escapement Device. (h) Selector and Orientor: The purpose of the selector and/or orientor is to establish the proper orientation of the components for the assembly workhead. A selector is a device that acts as a filter, which allows only those parts to pass through which are in correct orientation. Improperly oriented components are rejected back into the hopper. An orientor is a device that allows properly oriented parts to pass through but provides a reorientation of components that are not properly oriented initially. (Refer to Figure 12.19.) FIGURE 12.19 Selector and Orientor. TYPES OF FEEDERS Apron feeders Belt feeders Vibratory feeders Rotary feeders Reciprocating feeders Disc feeders Screw feeders Centrifugal feeders Flexible feeders Some of the commonly used feeders are described below: APRON FEEDERS Apron feeders are useful for feeding large tonnages of bulk solids being particularly relevant to heavy, abrasive, ore-type bulk solids and materials requiring feeding at elevated temperatures. They are also able to sustain extreme impact loading. Apron feeders consist of a continuous steel belt that is made up of overlapping flights or pans that are connected to and supported by steel chains or bars. The underside of these flights is reinforced and designed to withstand impact and pressure. An endless conveyor travelling over rollers is created. Some feeders are made of extra-heavy structural steel and are particularly suited to handling coarse, abrasive material. Apron feeders provide a positive material flow and with variable speed drives can provide close control of the feed rate to the crusher. These feeders have the limitation of high cost and limited length. (Refer to Figure 12.20.) FIGURE 12.20 Apron Feeders. RECIPROCATING FEEDERS (PLATE FEEDERS) Plate feeders, also known as reciprocating feeders (refer to Figure 12.21), are in widespread use, usually at the tail end of a conveyor or elevator to relieve pressure and drag. Designed for feeding at a fixed rate, a plate is driven reciprocally under a head of bulk material. Size varies and the feed rate can be controlled easily. These feeders use rollers to support the belt and are engineered for maximum uptime. They often are used in wet applications, and products ranging from sand and gravel to crushed stone that pass over belt feeders. FIGURE 12.21 Reciprocating Feeders. Advantages Low cost. Ability to handle a wide range of miscellaneous materials. Disadvantages Not self-cleaning. Not recommended for highly abrasive materials. RECIPROCATING-TUBE HOPPER FEEDER A reciprocating-tube hopper feeder (refer to Figure 12.22) consists of a conical hopper with a hole in the center through which the delivery tube passes. Relative vertical motion between the hopper and the tube is achieved by reciprocating either the tube or the hopper. During the period when the top of the tube is below the level of parts, some parts will fall into the delivery tube. FIGURE 12.22 Reciprocating Tube Hopper Feeder. RECIPROCATING PLATE FEEDER A reciprocating plate feeder consists of a plate mounted on four wheels and forms the bottom of the hopper. (Refer to Figure 12.23.) When the plate is moved forward, it carries the material with it; when moved back, the plate is withdrawn from under the material, allowing it to fall into the chute. The plate is moved by connecting rods from cranks or eccentrics. The length and number of strokes, width of plate, and location of the adjustable gate determine the capacity of this feeder. FIGURE 12.23 Reciprocating Plate Feeder. VIBRATORY BOWL FEEDER Vibratory parts feeding is a technology used to orient (proper position), singulate (proper quantity), and differentiate (separate/sort) and move parts to a desired location. Vibratory bowl feeders are used for feeding in oriented form, a wide range of components such as steel balls, nuts, belts, washers, rivets, nails, caps, plugs, spoons droppers, rings, and various other components having very odd shapes. The vibratory bowl feeder is the oldest and still most common approach to the automated feeding (orienting) of industrial parts. These feeders are very useful for automatic feeding of components to various machines and on automatic assembly lines such as presses, grinders, threading machines, wadding machines, knurling machines, etc. The size of the feeders varies from 200 mm–1000 mm diameters depending upon size, shape, and weight of components. There are no moving parts in the equipment and thus no wear and tear and needs no maintenance. Vibratory Feeder Terminology (a) Hopper (Storage Hopper): The storage hopper is the storage area provided to backlog bulk parts prior to entering the feeder bowl. This hopper eliminates overloading or insufficient loads of parts, causing the bowl not to function as required. Feed rate from the hopper to a bowl is metered by a level control switch. (b) Basic Bowl: Basic bowls are not off-the-shelf standard items. They are individually designed and can be supplied for any profile of part up to approximately 5” long. (c) Feeder Bowl: The feeder bowl is the actual orienting and feeding device that orients the part according to machine requirements and is the heart of the system. The feeder bowl is always custom tooled to a specific part configuration. Feeder bowls are almost always round and constructed of stainless steel for long life. (d) Rate (or Feed Rate): Feed rate is the number of parts per minute, or per hour, which meets the production requirements. (e) Selector: An area of the system designed and custom fit to profile only the properly positioned part. Parts entering a selector, which are not in the proper position, are diverted out of the feed line. (f) Confinement: The fabrication installed to ensure 100% control of the part after a selector ejects improperly positioned parts. (g) Return Pan: An extra pan-like area welded to the outside of the bowl, which catches excess and rejected parts falling from the track. The pan guides these parts back into the interior of the bowl for recirculation. (h) Discharge of the Feeder Bowl: This is the last section of the bowl. In most cases, it is a straight exit that confines the parts after they are oriented. (i) Track Switch: A switch of any type, which turns the feeder off whenever, the tracking to the machine becomes full. The track switch also helps to eliminate wear, noise, and jams within the feeder. Air, photoelectric, proximity, and electro-mechanical track switches can be used. Construction and Working of Vibratory Bowl Feeders Vibratory bowl feeders are commonly used for aligning and feeding small parts. A typical vibratory bowl feeder consists of a bowl mounted on a base by three or four inclined leaf springs. The springs constrain the bowl so that, as it travels vertically, it also twists about a vertical axis. As parts move up an inclined track along the edge of the bowl, tooling in the bowl orients parts into the proper orientation or rejects misaligned parts into the center of the bowl where they begin their travel up the track again. An electromagnet, mounted between the base and the bowl, generates the force to drive the bowl feeder. The feeder base rests on rubber feet, which serve to isolate the vibration of the feeder from the surrounding environment. The drive unit, equipped with a variable-amplitude controller, vibrates the bowl, forcing the parts to move up a circular, inclined track. The track is designed to sort and orient the parts in consistent, repeatable positions, according to certain requirements. The length, width, and depth of the bed of the vibratory feeder can be adjusted, and special bed liners can be installed if the material to be handled is abrasive. Dust-proof outlet covers can be attached to the inlet and discharged to reduce dusting caused by dusty material. A part feeder takes in a stream of identical parts in arbitrary orientations and outputs them in a uniform orientation. It consists of a bowl filled with parts surrounded by a helical metal track. The bowl and track undergo an asymmetric helical vibration that causes parts to move up the track, where they encounter a sequence of mechanical devices such as wiper blades, grooves, and traps. Most of these devices are filters, that serve to reject (force back to the bottom of the bowl) parts in all orientations except for the desired one. Thus, a stream of oriented parts emerges at the top after successfully running the gauntlet. A picture of a section of the vibratory bowl feeder track is given in Figure 12.24. The parts move from the right to the left on the feeder track. Parts in undesired orientations fall back into the bowl, other orientations remain supported. FIGURE 12.24 Vibratory Bowl Feeder Track. Figure 12.25 shows a typical vibratory bowl feeder used to sort small parts for an automated assembly system. Large numbers of unoriented parts are placed into the device and move up the spiral track on the bowl’s interior by means of vibratory motion. Parts reach the top of the track in single file in one of a finite number of stable orientations where they interact with a series of features built into the track and bowl wall. FIGURE 12.25 Vibratory Bowl Feeder. Controls for Vibratory Feeders There are a variety of controls available for the different types of vibratory feeders. Pneumatic feeder controls include a quick-acting valve, an airline filter, a pressure regulator gauge, a lubricator, and a long air hose. A transformer-type device, available for electromagnetic vibratory feeders, adjusts the intensity of vibration by varying the applied voltage. Electromechanical feeders have a wall-mounted control box with an on/off button or switch and overload protection. Special controls for remote operation include two speed, maximum-to-minimum material flow controls for batch weighing, and panel board controls for multiple feeder installation. An accelerometer can be attached to the drive unit to monitor the amplitude and to apply a correction to the feeder, which tends to vibrate more quickly as the material level drops. Applications of Vibratory Feeders Vibratory feeders are utilized in the pharmaceutical, automotive, chemical, and mining industries. Other industries include steel, glass, foundry, concrete, recycling, bakery, railroad unloading, and plastics. Chemical plants typically use vibratory feeders to control the flow of ingredients to the mixing tanks. Foundries use them to add binders and carbons to sand reprocessing systems. The pulp and paper industry uses vibratory feeders for chemical additive feeding in the bleaching process, while the metal working industry uses them for feeding metal parts to beat treating furnaces. Water and sewage treatment plants also use vibratory feeders in chemical additive handling. Other materials that are separated by vibratory feeders include powder, plastic pellets, dry chemicals, coal, metals, ore, minerals, aluminum, mining and aggregates, grains, seed, dry detergents, ceramics, textiles, rubber, fibers, wood chips, salt, sugar, and many more. SCREW FEEDERS Screw feeders are among the most widely used types of bulk-solids- handling equipment in the chemical industry. These are well suited for use with bins having elongated outlets. A screw feeder is shown in Figure 12.26. Screw feeders can be used to deliver bulk solids at tremendous range of feed rates. Bulk solid materials are usually metered into production processes from storage containers, such as hoppers and bins. These feeders have an advantage over belt feeders that in these feeders there is no return element to spill solids. Because a screw is totally enclosed, it is excellent for use with fine, dusty materials. Screw feeders require less maintenance than belt feeders. All screw feeders are volumetric devices. This means that they are intended to deliver a certain volume of bulk material per revolution of the screw. The volume delivered depends on the screw outside and inside (shaft) diameter and the pitch of the screw. Commercial feeders often use a variety of agitation devices to ensure that the solids enter the screw in a highly flowable state. These devices may stir, massage, or vibrate the solids in order to fill the screws as completely, or at least as uniformly, as possible. FIGURE 12.26 Screw Feeder. BELT FEEDERS Belt feeders are used to provide a controlled volumetric flow of bulk solids from storage bins and bunkers (refer to Figure 12.27). Like screw feeders, belt feeders can be an excellent choice when there is a need to feed material from an elongated hopper outlet. Belt feeders generally can handle a higher flow rate than screw feeders. They generally consist of a flat belt supported by closely spaced idlers and driven by end pulleys. These types of feeders have more moving parts and, therefore, generally require more attention and maintenance than a well-designed screw or vibrating bowl feeder. They usually also require more installation space than screw feeders. Some particular features of belt feeders include: Suitable for withdrawal of material along slotted hopper outlets when correctly designed. Can sustain high-impact loads from large particles. Flat belt surfaces can be cleaned quite readily allowing the feeding of cohesive materials. Suitable for abrasive bulk solids. Capable of providing a low initial cost feeder. FIGURE 12.27 Belt Feeder. ROTARY PLOW FEEDERS Rotary plow feeders are often used under large stockpiles because of lower capital and operating costs, as well as greater ease of maintenance. This system can be used to move minerals ranging from coal to iron ore stored at mine sites, processing facilities, and power plants. The mechanism by which a rotary plow moves material is as follows: When a rotary plow begins operating, it loosens material in a narrow vertical channel above it. If twin plows are used and both are operating, two channels will form independent of each other. The pressures exerted on the material adjacent to the channels are generally low and proportional to the size of the flow channel. If a plow does not traverse under the stockpile, and if the material has sufficient cohesive strength, the channel eventually empties out, forming a rat hole. However, as a plow traverses, the narrow plow channel lengthens. Whether material on either side of it remains stationary or slides depends on the wall friction angle along the sloping wall, the wall angle, and the head of the material. If the material slides, it does so for only a short distance, because, as the material in the flow channel is compressed, the pressure it exerts on the adjacent material increases, resulting in a stable mass. If the side material does not slide, the level of material in the flow channel drops and material sloughs off the top surface. ROTARY TABLE FEEDERS The rotary table feeder can be considered as an inverse of the plough feeder. It consists of a power-driven circular plate rotating directly below the bin opening, combined with an adjustable feed collar which determines the volume of bulk material to be delivered. The aim is to permit equal quantities of bulk material to flow from the complete bin outlet and spread out evenly over the table as it revolves. The material is then ploughed off in a steady stream into a discharge chute. This feeder is suitable for handling cohesive materials, which require large hopper outlets, at flow rates between 5 and 125 metric tons per hour. Feed rates to some extent are dependent on the degree to which the material will spread out over the table. This is influenced by the angle of repose of the material, which varies with moisture content, size distribution, and consolidation. These variations prevent high feed accuracy from being obtained. Rotary table feeders are suitable for bin outlets up to 2.5 m in diameter; the table diameter is usually 50 to 60% larger than the hopper outlet diameter. With some materials, a significant dead region can build up at the center of the table. This can sometimes be kept from becoming excessive by incorporating a scraping bar across the hopper outlet. It is important to ensure that the bulk material does not skid on the surface of the plate, severely curtailing or preventing removal of the bulk material. CENTRIFUGAL HOPPER FEEDER A centrifugal hopper feeder shown in Figure 12.28 is a feeder system, with a central flat or conical turntable, which drives the working materials via a rotary action. The resulting centrifugal force causes workpieces to separate out of the heap and move towards the edge of the drum. Here, they meet the delivery ring and slide onto the ramp. The speeds of the turntable and the delivery ring can be adjusted separately. Separated out workpieces can be aligned by orienting devices and, thus, proceed to the pick-up point correctly orientated. Incorrectly orientated workpieces pass back into the hopper. Complex workpieces can be fed by means of a conveyor belt. Any excess conveyed workpieces fall back into the heap in the hopper. FIGURE 12.28 Centrifugal Hopper Feeder. CENTERBOARD HOPPER FEEDER In this feeder, a blade made of hardened steel, with a shaped top is oscillated up and down (by a crank mechanism) through a mass of parts. Properly oriented parts are picked by the blade and discharged by gravity into the track. This type of feeder is suitable for parts having simple shape like balls, cylinders, nuts and bolts, rivets, etc. These are very robust and have a long working life. The capacity of a centerboard hopper is large. The disadvantage of these is that they cannot be used for fragile components and the degree to which they can orient is rather limited. FIGURE 12.29 Centerboard Hopper Feeder. FLEXIBLE FEEDERS A crucial component of any assembly system is the parts feeders. Unfortunately, parts feeders are also one of the most specialized components of such systems. While a modular approach could be employed which would allow specialized feeders to be quickly brought into the work cell, there are several difficulties, which present themselves using this approach. First, a feeding system tends to be large and bulky in comparison to other specialized components, such as a robot’s gripper. Size alone could make storing all the specialized feeders difficult. Second, a feeding system is generally more expensive than other specialized components. It might be difficult to economically justify building several new feeders for each new product being assembled. Third, the lead-time to build and adjust most current feeding systems is rather long. This diminishes the ability of the work cell to be rapidly adapted to new products. Therefore, simply placing CHAPTER 17 APPLICATIONS OF ROBOTS INTRODUCTION The present-day applications for robots are much broader than most people realize. While the emphasis in robot development is on industrial robots, factories are not the only place where robots are used. Small, medium, and large companies in just about every industry are taking a fresh look at robots to see how this powerful technology can help them solve manufacturing challenges. In business offices and elsewhere, robots serve as mail delivery carts, promotional or show robots, laboratory assistants, hospital orderlies, and window washers. In general, industrial robots are best used for jobs that are dirty, dull, dangerous, or difficult, the types of jobs that humans do most poorly. Robots are used in a wide range of industrial applications. The first commercial application of an industrial robot took place in 1961, when a robot was installed to load and unload a die-casting machine. This was an unpleasant task for human operators. Many robot applications took place in areas where a high degree of hazard or discomfort to humans existed, such as in welding, painting, and foundry operations. The earliest applications were in materials handling, spot welding, and spray painting. Robots were initially applied to jobs that were hot, heavy, and hazardous such as die-casting, forging, and spot welding. The reasons for using robots are: 1. Reduced costs—robots can perform tasks more economically than humans. 2. Improved productivity—robots are not only less expensive than human labor, but also have higher rates of output. This increase in productivity is due to robot’s slightly faster work pace but much is the result of the robot’s ability to work almost continually, without lunch breaks and rest periods. 3. Better quality—robots have a distinct advantage of being able to perform repetitive tasks with a higher degree of consistency, which in turn leads to improved product quality. 4. Elimination of hazardous tasks. ROBOT CAPABILITIES The three important capabilities of robots that make them useful for applications are: Transport Manipulation Sensing Transport Material handling is one of the basic operations, which is performed on an object as it passes through the manufacturing process. The object is moved from one location to another to be stored, machined, assembled, or packaged. The robot’s capability to acquire an object, move it through space, and release it makes it ideal for transport operations. Simple tasks such as part transfer from one conveyor to another may only require one-or two- dimensional movements, which are often performed by non-servo robots. Other parts handling operations such as machine loading and unloading and packaging may be more complicated and require varying degrees of manipulative capability. Servo-controlled robots perform these operations. Manipulation Another basic operation performed on an object as it is transformed from raw material to a finished product is processing, which generally requires some types of manipulation, i.e., workpieces are inserted, oriented, or twisted to be in proper position for machining, assembly, or some other operation. A robot’s capability to manipulate both parts and tooling make it very suitable for processing applications. Examples in this regard include spot and arc welding, and spray painting. Sensing A robot’s ability to react to its environment by means of sensory feedback is also important, particularly in applications like assembly and inspection. These sensory inputs may come from a variety of sensor types, including proximity switches, force sensors, and machine vision systems. In each application, one or more of the robot’s capabilities of transport, manipulation, or sensing is employed. These applications make a robot ideal for many applications now performed manually. APPLICATIONS OF ROBOTS Manufacturing Applications Arc and spot-welding Spray painting Machine loading and unloading Machining Die casting Forging Investment casting Parts transferring Plastics molding Finishing Assembly Inspection Materials Handling Transport goods Pick and place Palletizing Space Industry Robot arms used as manipulator to handle bulky telescopes Mounted on space shuttle or repair craft Military Remote bomb detonation Smart bombs Medical Applications Intelligent wheelchairs Robot arms used to manipulate and handle patients MANUFACTURING APPLICATIONS Welding Perhaps the most popular applications of robots are in industrial welding. The repeatability, uniformity quality, and speed of robotic welding are unmatched. A robot performing a welding operation is shown in Figure 17.1. The two basic types of welding are spot welding and arc welding, although laser welding is done. Some environmental requirements should be considered for a successful operation. The automotive industry is a major user of robotic spot welders. The other major welding task performed by robots is arc or seam welding. In this application, two adjacent parts are joined together by fusing them, thereby creating a seam. FIGURE 17.1 Robot Performing Welding Operation. Why should robots be used for welding? A welding process that contains repetitive tasks on similar pieces might be suitable for automation. The number of items of any type to be welded determines how difficult automating a process will be or not. If parts normally need adjustment to fit together correctly, or if joints to be welded are too wide or in different positions from piece to piece, automating the procedure will be difficult or impossible. Robots work well for repetitive tasks or similar pieces that involve welds in more than one axis. The most prominent advantages of automated welding are precision and productivity. Robot welding improves weld repeatability. Once programmed correctly, robots will give precisely the same welds every time on workpieces of the same dimensions and specifications. Arc Welding Arc or fusion welding is considered a major growth area for the application of robotics (Refer to Figure 17.2). The process is very hostile to the operator, generating noise, fumes, and intense light. Automation produces high quality welds with greater consistency and at a faster rate. In general, equipment for automatic arc welding is designed differently from that used for manual arc welding. Automatic arc welding normally involves high-duty cycles, and the welding equipment must be able to operate under those conditions. In addition, the equipment components must have the necessary features and controls to interface with the main control system. FIGURE 17.2 Robots for Arc Welding. A special kind of electrical power is required to make an arc weld. A welding machine, also known as a power source, provides the special power. All arc-welding processes use an arc welding gun or torch to transmit the welding current from a welding cable to the electrode. They also provide for shielding the weld area from the atmosphere. The nozzle of the torch is close to the arc and will gradually pick up spatter. A torch cleaner (normally automatic) is often used in robot arc welding systems to remove the spatter. All of the continuous electrode wire arc processes require an electrode feeder to feed the consumable electrode wire into the arc. The process is applied to automobile subassemblies mainly for reasons of strength, low distortion, high-speed applications where one-sided access is required, and sealing. Spot Welding Automatic welding imposes specific demands on resistance welding equipment. Often, equipment must be specially designed and welding procedures developed to meet robot-welding requirements. The spot welding robot (Refer to Figure 17.3) is the most important component of a robotized spot welding installation. Welding robots are available in various sizes, rated by payload capacity and reach. Robots are also classified by the number of axes. Spot welding involves applying a welding tool to some object, such as a car body, at specified discrete locations. A spot welding gun applies appropriate pressure and current to the sheets to be welded. This requires the robot to move its hand (end effector) to a sequence of positions with sufficient accuracy to perform the task properly. It is desirable to move at a high speed to reduce cycle time, while avoiding collisions and excessive wear or damage to the robot. FIGURE 17.3 Spot-welding Gun. There are different types of welding guns, used for different applications, available. An automatic weld-timer initiates and times the duration of current. A robot can repeatedly move the welding gun to each weld location and position it perpendicular to the weld seam. It can also replay programmed welding schedules. A manual-welding operator is less likely to perform as well because of the weight of the gun and monotony of the task. Spot welding robots should have six or more axes of motion and be capable of approaching points in the work envelope from any angle. This permits the robot to be flexible in positioning a welding gun to weld an assembly. A robot easily performs some movements that are awkward for an operator, such as positioning the welding gun upside down. Typical components of an integrated robotic spot welding cell are: Spot welding robot Spot welding gun Weld timer Electrode tip dresser Spot welding swivel Electron Beam Welding Electron beam welding (EBW) is a fusion joining process that produces a weld by impinging a beam of high-energy electrons to heat the weld joint. Electrons are elementary atomic particles characterized by a negative charge and an extremely small mass. An electron beam-welding gun uses a high intensity electron beam to target a weld joint. The weld joint converts the electron beam to the heat input required to make a fusion weld. The electron beam is always generated in a high vacuum. The use of specially designed orifices separated a series of chambers at various levels of vacuum permits welding in medium and non-vacuum conditions. Although, high vacuum welding will provide maximum purity and high depth to width ratio welds. An electron beam robot welding system benefits the customer with a low contamination vacuum, narrow weld zone, uses low filler metal, and has low distortion. MIG Welding Gas metal arc welding (GMAW) is frequently referred to as MIG welding. MIG welding is a commonly used high deposition rate welding process. Wire is continuously fed from a spool. MIG welding is therefore referred to as a semiautomatic welding process. Robotic systems are integrated towards MIG welding applications on a consistent basis. With advances in technology, and the benefits of a GMAW robotic cell, factories and job shops large and small are investing in a robot. Return on investment of a robotic system is possible after just a few years. M I G welding robots, or GMAW cells have many benefits for customers. Robots are all position capable, have higher deposit rates than SMAW, need less operator skill, can perform longer welds without stopping, and have minimal post weld cleaning. TIG Welding Gas tungsten arc welding (GTAW) is frequently referred to as TIG welding. TIG welding is a commonly used high-quality welding process. TIG welding has become a popular choice of welding processes when high quality, precision welding is required. In TIG welding, an arc is formed between a non-consumable tungsten electrode and the metal being welded. Gas is fed through the torch to shield the electrode and molten weld pool. If filler wire is used, it is added to the weld pool separately. A TIG welding robot system has many benefits to customers. A robot produces high-quality welds, welds can be made with or without filler metal, variable precise controls, low distortion, and free of spatter. Spray Painting Another popular and efficient use for robots is in the field of spray painting. The consistency and repeatability of a robot’s motion have enabled near perfect quality while at the same time wasting no paint. The spray painting applications seems to epitomize the proper applications of robotics, relieving the human operator from a hazardous, albeit skillful job, while at the same time increasing work quality, uniformity, and cutting costs. In spraying applications, the robot manipulates a spray gun, which is used to apply some material such as paint, stain, or plastic powder, to either a stationary or moving part. These coatings are applied to a wide variety of parts, including automotive body panels, appliances, and furniture. The spray-painting environment constitutes a fire hazard and is also dangerous to human worker’s respiration. An early paint-spraying machine was built by Pollard in the 1930s. Today, this machine would be called an industrial robot. Robots perform painting, coating, and dispensing jobs in many industries today. Companies making products such as motorcycles, bicycles, boats, jet skis, and cars are using painting automation to their advantage. Painting robots are generally equipped with six axes, three for the base motions and three for applicator orientation. Some units incorporate machine vision for guidance or to check application quality. Typically, these painting robots are electrically driven, rather than hydraulically or pneumatically powered. Advantages of using spray-painting robots (Figure 17.4) include: FIGURE 17.4 Robots for Spray Painting. 1. Humans are removed from a hostile environment. 2. Less energy is needed for fresh air requirements and the need for protective clothing is reduced. 3. The quality of the painting is improved, reducing works and warranty costs. 4. Less paint and other materials are used. 5. Direct labor costs are reduced. Machine Tending Machine loading and unloading is a more complex application than basic material handling; for this application, the robot provides both manipulative and transport capabilities (Refer to Figure 17.5). Robots can be used to grasp a workpiece from a supply point (e.g., a conveyor belt), transport it to a machine, orient it, and then insert it into the machine work holder. This may require that the robot signal the machine tool when the workpiece is in the correct position, so that the part can be secured in the work holder. The robot then releases the part and withdraws the arm so that machining can begin. Upon completion of the machining, the robot unloads the workpiece and transfers it to another machine or conveyor. In a robotic cell, a single robot can service several machines. The single robot may be used to perform other operations while the machines are performing their primary functions. This may require that the robot be able to exchange end- effectors. Examples of machine tending functions include the following: FIGURE 17.5 Machine Tending Operation. Exchanging machine tools, such as lathe and machining centers. Stamping press loading and unloading. Tending plastic injection molding machines. Holding a part for a spot welding operation. Loading hot billets into forging presses. Loading auto parts for grinding. Loading gears onto CNC milling machines. Forging Robots have been applied in many different types of forging applications such as hammer forge operations, upsetter operations, roll forges, hot forming presses, and draw bench applications. In some cases, a robot acts as a forging machine operator or as a role of forge helper. Forge hammers: Forging hammers are either hydraulic, steam hydraulic, or air driven. One-half of the forming die is on the anvil, and the other half of the die is on a ram that moves up and down, either under force by air, steam, or gravity. Under the control of an operator, the hammer is allowed to strike the part that is lying between the two dies a certain number of times. Depending on the observation of the forging operator, the operator determines when to take a part out of one die and moves it into the next. The function of the robot in this application can be to act as a forge helper. When working heavier parts, the robot can be used to load and unload furnaces and process the billets to the forging bed, where the operator can take over and process the parts through the various forming cycles. The robot then can maneuver the finished product to a trim operation. Die Casting Die casting involves forcing nonferrous metals into dies under high pressure to form parts of a desired shape. A typical die-casting task involves unloading a part from the die-casting machine, quenching the part, and then disposing of the part on a conveyor belt or into a bin. A possible robot task cycles in die-casting could include any of the following: 1. Alternately loading two or more die casting machines. 2. Unloading, quenching, trimming, and disposing of the part. 3. Unloading the die casting machine and preparing the dye for the next casting cycle. (This would require another gripper or attachment to spray the die.) 4. Loading an insert into the die casting machine and unloading the finished part. Plastic Molding The plastic molding process is typically used for thermoplastic materials. The material to be molded is supplied in a granule form and moved from a hopper to a cylinder, from which a plunger forces the granules through a heat chamber into the mold. Then, the mold half opens, and the products are withdrawn. Many automotive parts are injection molded today, as well as many parts utilized in household appliances. The robot is typically employed to remove the part from the mold either by grasping a sprue and runner assembly. A robot is typically used at an injection molding machine workcell where parts must not be dropped because of fragility, or where runs are so short that it is not economic to build a totally automatic mold to drop the part through the bottom of the machine. Assembly This is the process of robot manipulation of components, resulting in a finished assembled product. Assembly (Refer to Figure 17.6) is the process of fitting and holding together parts and assemblies; generally performed by means of nuts, bolts, screws, fasteners, or snap-fit joints. Examples of assembly operations include: FIGURE 17.6 Robot Doing Assembly Operation. Assembly of computer hard drives. Insertion of lamps into instrument panels. Insertion and placement of components onto printed circuit boards. Automated assembly of small electric motors. Furniture assembly. Sealing/Dispensing For dispensing applications, the robot manipulates a dispenser or gun to apply a material such as paint, adhesive, sealant, or washing solution to a stationary or moving part. Additional equipment used to complete a dispensing system may include material containers, pumps, and regulators. It is very important that, for operations that involve the application of material that produces flammable or explosive fumes, the robot be sealed and have a system that purges the robot’s internal cavities. If the robot is not sealed and does not have a purging system, the possibility of the ignition of flammable or explosive fumes by the arcing of the robot’s internal electrical components (i.e., motors, electronic components, and electrical connections) exists. In applications where the part is on a conveyor line, the robot’s motion is coordinated with the motion of the conveyor. The manipulative capability of the robot is the primary function that makes a robot especially well suited for dispensing applications. The major benefit of using a robot (Figure 17.7) for dispensing applications is that a robot provides uniform application of material (repeatability). Other benefits include a reduction of labor costs, coating material waste, and exposure of workers to hazardous materials. FIGURE 17.7 Robot Performing Sealing Operation. Examples of dispensing applications include: Painting parts on an automated line. Application of adhesive and sealant to car bodies. Application of thermal material to rockets. Washing. Inspection and Measurement With a growing interest in product quality, the focus has been on zero defects. However, the human inspection system has somehow failed to achieve its objectives. Robot applications of vision systems have provided services in part location, completeness and correctness of assembly products, and collision detection during navigation. Machine vision applications require the ability to control both position and appearance in order to become a productive component of an automated system. Material Removal Robotic material removal is a new application that has many uses in industrial automation. The robot can grind, roll, and file metal parts to precision. The robotic system can remove material with quality every single time it is being used. The robot can work long hours and produce more throughputs. Robotics has become more affordable and many factories are looking to buy a robotic deburring cell for their operation. With expert engineering, any material removal application can bring your company a good return on investment (ROI). A material removal robot can be very beneficial to an operation because of the robot’s precision and quality. With a material removal robot system technology can be as precise as removing spots on jewellery. Material removal can be described as a deburring process, and can be integrated to the most precise applications. Companies can automate a material removal process and gain many benefits. Deburring Robotic deburring is the use of a robot to remove burrs, sharp edges, or fins from metal parts. The robot can grind, roll, and file metal parts to precision (Refer to Figure 17.8). The robotic system can produce a deburring application, i.e., quality every single time it is being used. The robot can work long hours and produce more throughputs. Robotics has become more affordable and many factories are looking to buy a robotic deburring cell for their operation. A robotic deburring cell can work long hours without fatigue removing rough edges. The quality of the process, as well as the long hours of a robotic cell is unmatched. FIGURE 17.8 Robot Performing Deburring Operation. Grinding Manual grinding is tough, dirty, and noisy work. The metal dust produced by grinding is harmful to employee’s eyes and lungs. Grinding robots remove excess material from the surface of machined parts/products quickly and efficiently. A robo