DNA Synthesis Handout (PDF)
Document Details
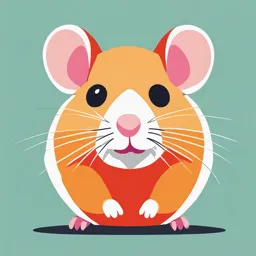
Uploaded by WittyRealism
Dr. Cox
Tags
Summary
This handout details various aspects of DNA replication, including processes like bidirectional replication, semi-conservative replication, the actions of DNA polymerase and replication fork, and Okazaki fragments. It also covers eukaryotic DNA polymerases, telomerase, reverse transcriptase, different DNA repair pathways, and the consequences of human DNA repair defects. The handout highlights the importance of understanding DNA replication and repair processes for various medical and biological applications.
Full Transcript
Learning Objectives: Dr. Cox DNA Replication and DNA repair: Focus your study toward understanding the following points. 1. Describe DNA synthesis as bidirectional, semi-conservative, and requiring factors such as...
Learning Objectives: Dr. Cox DNA Replication and DNA repair: Focus your study toward understanding the following points. 1. Describe DNA synthesis as bidirectional, semi-conservative, and requiring factors such as helicase, gyrase and several others. 2. Describe action of DNA polymerase and events at replication fork. 3. Describe DNA synthesis on the lagging strand as requiring multiple Okazaki fragments that are subsequently joined together. 4. Describe eukaryotic DNA polymerases (α , ß, γ,δ,є), what do they do? 5. Explain the enzymes telomerase and reverse transcriptase- what do they do? 6. Explain the basic DNA repair pathways. 7. Explain nucleotide excision repair mechanism as distinct from base excision repair. 8. Explain mismatch repair. 9. Describe the basic human repair defects, what are the sensitivities of the defects ? 1 Dr. Cox, 2024 DNA Replication and DNA Repair Reading Assignment: Chp 13, Mark’s Basic Medical Biochemistry, 5thed. I. DNA Replication in prokaryotes Bacterial chromosomes are circular DNA molecules with a single origin of replication. A. Bidirectional replication Dna A protein binds to the DNA origin of replication and unwinds a short stretch of single-strand DNA so that DNA synthesis can initiate. With the assistance of helicase,gyrase, and single-strand binding protein, synthesis can occur at two replication forks with DNA polymerase that proceeds bidirectionally. B. Semiconservative replication – all DNA synthesis occurs like this because it requires a template. Each new daughter DNA chromosome contains one parental DNA strand and one newly synthesized complementary strand. C. DNA unwinding Helicases (i.e. DNA B) unwind the double-strand DNA locally to single-strand to be copied. Single-strand binding proteins hold the single strands apart and protect the single-strand DNA from nucleases. Topoisomerases prevent excessive supercoiling due to DNA unwinding. DNA gyrase is a major type of topoisomerase. [Topoisomerase II in eukaryotes is the target molecule for some of the most important anti- cancer drugs such as adriamycin and etoposide. Ciprofloxacin (Cipro) is a widely used antibiotic which is active against gyrase. New antifungal, antiparasitic and antiviral agents are being directed at topoisomerases.] D. DNA polymerase action DNA polymerases are enzymes that catalyze the synthesis of DNA. All DNA polymerases synthesize DNA in the 5’-to-3’ direction. Deoxyribonucleotides (dATP,dGTP,dCTP, and dTTP) are substrates that are added one at a time to growing DNA chains using parental DNA as a template. The free 3’ hydroxyl of the growing chain forms a phosphate ester to the first phosphate of the triphosphate, cleaving off pyrophosphate. Processive synthesis means the polymerase remains attached to the DNA during synthesis. Table 13.1 Functions of Bacterial DNA Polymerases Polymerases Functions Exonuclease Activity Pol I Fill of gap after removal of 5’-to-3’ and RNA primer 3’ –to-5’ DNA repair Removal of RNA primer in conjunction with RNase H Pol II DNA repair 3’ –to-5’ Pol III Replication; synthesis of DNA 3’ –to-5’ E. Base-pairing errors The major replicative enzyme of E.coli is DNA pol III. It has a 3’-5’ exonuclease activity that immediately removes any mistakes incorporated into the growing chain. F. RNA primer requirement DNA polymerases require a pre-existing primer in order to synthesize DNA. In vivo the primer is an oligonucleotide of RNA synthesized by an RNA polymerase named primase. G. Replication Fork DNA synthesis occurs towards the replication fork on the leading strand and discontinuously away from the fork on the lagging strand. On the lagging strand short segments of DNA are synthesized that are known as Okazaki fragments. Okazaki fragments are joined together such that overall DNA synthesis is towards the replication fork on the lagging strand. H. DNA ligase DNA polymerase I removes RNA primers during DNA synthesis with a 5’-to-3’exonuclease. As the primer is removed, DNA pol I synthesizes DNA to fill the gap. DNA ligase is then required to join the DNA stretches together, sealing a phosphodiester bond (nick). II. DNA synthesis in eukaryotes The same processes for DNA synthesis occur in eukaryotes only the enzymes and protein factors are slightly different. Eukaryotic cells have over 1000 times more DNA than prokaryotic cells. In addition, eukaryotic DNA is wrapped onto histone proteins. A. Eukaryotic cell cycle. The G1 or gap 1 phase of the cell cycle is variable in length in most cells. S phase is where all nuclear DNA is replicated and is typically 6-8 hours. After S phase, the cells go through G2 phase where they prepare for cell division or mitosis. Mitosis or M phase is generally rather short in length. B. Origins of replication. Each chromosome has many origins of replication. DNA synthesis proceeds bidirectionally simultaneously from all the origins of replication arrayed along a chromosome. C. Eukaryotic DNA polymerases. Fifteen different DNA polymerases have been identified in eukaryotic cells. Most types are involved in DNA repair (see later). Polymerases delta and epsilon are the major replication DNA polymerases. Pol α DNA replication and repair. Pol β DNA repair Pol γ mitochondrial DNA synthesis Pol δ lagging strand DNA synthesis Pol ε leading strand DNA synthesis Pol κ, etc. DNA repair D. Eukaryotic Replication Complex Polymerase (Pol) alpha is a primase (elongates short RNA primers) and begins DNA synthesis on both strands. Pol alpha then falls off and Pol delta continues synthesis on the lagging strand and Pol epsilon on the leading strand. RNase H removes the RNA primers and the gap is filled with DNA Pol delta. PCNA protein (proliferating cell nuclear antigen) serves as a clamp for DNA polymerases and is useful for distinguishing proliferating cells in tissues in the clinic with antibodies to PCNA. E. Replication of chromosome ends The ends of linear chromosomes are called telomeres. A problem arises during replication of chromosomes in that 3’-overhangs are produced due to removal of primers at the 5’ ends. The cell overcomes this problem by extending the telomeres with an enzyme called telomerase. Many copies of a short TTAGGG sequence are added onto the ends of all chromosomes and made double stranded by DNA synthesis. F. Reverse Transcriptase Reverse transcriptase is an enzyme which synthesizes DNA from an RNA template. Retroviruses (HIV-1 is a retrovirus) contain reverse transcriptase and convert their RNA genomes into DNA during their replication cycles. The following figure shows the conversion of RNA to cDNA (complementary DNA) with the use of Reverse Transcriptase. The DNA can then be cloned into a plasmid as a copy of the original RNA. III. DNA Repair A. Actions of mutagens Some chemicals in the environment are known mutagens (can produce mutations in DNA). Mutagens which can produce cancer cells are known as carcinogens. Short wavelength UV- radiation can produce thymine dimers which are mutagenic if not removed from DNA. B. Repair Mechanisms The bases in DNA are usually altered in structure by a mutagen. A region containing the altered base is recognized and clipped out by repair enzymes (nucleases). A new patch of DNA is added by DNA polymerases to be sealed by DNA ligase. 1. Nucleotide Excision Repair 2. Base excision repair This type of repair is initiated when the damage to a base is minor in size, i.e. no bulky distortion to the DNA (thymine to uracil for example). DNA glycosylases remove the damaged base leaving a site that must be repaired. A short region containing the gap is removed and repaired as for nucleotide excision repair. 3. Mismatch repair DNA is methylated periodically on both strands. If mismatches occur during DNA replication, then the unmethylated, newly synthesized strand is repaired using the methylated parental DNA strand as a template. i.e. the two strands can be distinguished by the level of methylation (see next figure). 4. Transcription coupled repair – preferential DNA repair on genes undergoing transcription. Mechanism is not really clear on this type of repair. Human DNA repair defects Human DNA repair defects are very rare,inherited diseases that result in usually cancer at earlier ages. They are very difficult to treat- usually one can try to avoid DNA damaging agent- for example xeroderma pigmentosum patients should only go out at night to avoid solar UV! All require frequent monitoring so that tumors that arise can be caught early and treated. Disease Sensitivity Cancer Symptoms Xeroderma Ultraviolet Skin carcinomas, Skin and eye pigmentosum radiation melanomas photosensitivity Ataxiatelangiectasia Gamma irradiation Lymphomas Ataxia; dilation of blood vessels Fanconi's anemia Crosslinking Leukemias agents Bloom's Syndrome Ultraviolet Leukemias Photosensitivity Cockayne'sSyndrome Ultraviolet Various tumors Neurological defects, dwarfism Lynch syndrome Mismatch repair Colon cancer Learning Objectives: Nucleic Acids/ Chromatin structure Dr. Cox 1. Describe the composition of DNA and RNA. 2. Describe the structure of DNA and account for stability of DNA. 3. Describe major conformations of DNA. 4. Describe products produced by alkali treatment of DNA and RNA. 5. Describe periodicities in DNA - DNA B (Major and Minor groves). 6. Describe chromatin structure, histones and nonhistone role, DNA organization. 7. Describe role of acetylation of histones. Dr. Cox, 2024 Nucleic Acid / Chromatin Structure Reading Assignments: Chp. 12, Marks’ Basic Medical Biochemistry, 5th ed. I. Nucleic Acids: Unit Structure A. Location of DNA -Prokaryotic Chromosome -Viruses -Plasmids -Eukaryotic -Chromosome (nuclear) -Mitochondria -Viruses B. Both DNA and RNA are composed of nucleotides linked in linear chains. The nucleic acids. C. Components of a nucleotide. 1. Nitrogenous base - either a purine or a pyrimidine. 2. Sugar moiety - either ribose or deoxyribose 3. Phosphate D. Differences between DNA and RNA. 1. DNA contains: a. Nitrogenous bases: adenine, guanine, cytosine and thymine. b. Sugar moiety - 2-deoxyribose. c. Phosphate 2. RNA contains: a. Nitrogenous bases: adenine, guanine, cytosine and uracil. b. Sugar moiety - ribose. c. Phosphate 2 3. Differences: a. DNA contains 2-deoxyribose: RNA contains ribose. 1) DNA - β-2’-deoxyribose 2) RNA - β-D-ribose b. DNA contains thymine ; RNA contains uracil. Note: 5-Fluorouracil is an analogue of thymine that is used in cancer treatment. II. Structure of Nucleic Acids A. Linkage 1. Mononucleotides are joined together by phosphodiester bridges between the 5’-hydroxyl group of one nucleotide and the 3’-hydroxyl group of the adjacent nucleotide. The bases are not directly attached covalently to one another. B. Base Pairs: The bases of one strand form hydrogen bonds with the bases of the other strand such that adenine in one strand always pairs with thymine in the other strand and guanine in one strand always pairs with cytosine of the other strand. Therefore, in any DNA molecule the amounts of adenine and thymine are always the same while the amounts of guanine and cytosine are always the same. A=T; G=C 3 Also, [purine] = [pyrimidines] i.e., purines/pyrimidines = 1. 4 The DNA Double Helix (Basic Features) - 1. In both prokaryotic and eukaryotic cells DNA exists as a double helix in which two DNA strands are wrapped around the same axis. 2. DNA is a right handed double helix (an α-helix). 3. The phosphodiester bonds and the deoxyribose moieties that they link form the backbone of each strand with the nitrogenous bases projecting into the interior of the double helix. a. Polar groups: Phosphates project to the outside where they interact with proteins (ones carrying a positive charge at physiological pH). Negatively charged phosphates (at physiological pH) are complexed with cations such as Na, K and Ca in vivo. This leads to helix stabilization. b. Nonpolar groups: The interior of the molecular is very hydrophobic - water is excluded. 4. The nitrogenous bases are stacked in the interior of the double helix with the planes of the bases being parallel to each other and perpendicular to the long axis of the double helix. Because of the proximity of the stacked bases van der Waals attractive forces contribute greatly to the stability of the helix. a. A=T; G=C Also, [purine] = [pyrimidines] i.e., purines/pyrimidines = 1 b. Complementary Adenine and thymine are said to be complementary bases as are guanine and cytosine. The two strands of a DNA molecule are not identical but are complementary to each other. c. Frequency of A=T versus G≡C. Please note that the amount of A=T pairs does not necessarily equal the G≡C pairs. A section of DNA might have a lot more A=T pairs than G≡C. This point is important because the hydrogen bonding between A=T is weaker than G≡C. Therefore, in sections of DNA rich in A=T pairs the two strands of DNA can be separated more readily. X-ray crystallography of DNA shows two periodicities (repeating units), 3.4 Å and 34Å These correspond to the distance between stacked nitrogenous bases (0.34 nm) and the distance for one complete turn of the double helix (3.4 nm). There are 10 base pairs for each complete turn of the double helix. This is the B form or the most abundant, natural form of DNA. 5 Antiparallel The two strands of a DNA molecule are anti-parallel, that is one strand runs in the 5’→3’ directions while the other strand runs in the 3’→ 5’ direction. Major and minor grooves alternate along the DNA helix due to the bond angle between sugar and bases. Proteins can bind to these grooves. 6 Conformations of DNA- most DNA is in the B-DNA form. DNA Z has 12 pairs per each complete turn of the double helix. DNA Z has been observed in crystals of synthetic hexanucleotide d (CpG)3 and in fibers of the alternating d(GC)n polymers. Z DNA is in the form of a left-handed rather than a right-handed helix Ray BJ et al. PNAS, 2011 D. Properties of Nucleic acids Strong alkali solutions will separate strands of DNA and degrade RNA to nucleotides. 7 II. Structure of Chromosomes A. Size of DNA A human cell contains about 6.5 billion DNA bases organized into chromatin (chromosomes) that fit into a nucleus with a diameter of 3 micrometers ! Haploid human cells contain about 3.2 X 109 base pairs of DNA. Around 25,000 genes are estimated to exist for human cells. Around 10,000 different proteins are made in different types of cells from a very small part of the entire genome (~2%). Each cell in the human body contains 6 picograms of DNA. If all the DNA in a cell was stretched into one linear strand it would stretch over 1meter in length. Chromosomes are DNA- protein associations that allow 10 thousand-fold condensation of DNA in the nucleus. 8 DNA Associated Proteins (Chromatin) 1. General In eukaryotic cells the nuclear DNA is associated with proteins. These proteins are divided into two classes, histones and nonhistones. Histones, the major quantitative ones, package the DNA. Nonhistones are regulatory proteins, enzymes, and other structural proteins. 2. Histones There are five classes of histones proteins; H1, H2A, H2B, H3 and H4. All of the histones are very basic proteins due to the presence of high levels of arginine and lysine. At physiological pH the histones therefore have a large net positive charge and can bind to negatively charged molecules (like the exposed phosphates in DNA). The combination of DNA with histones and nonhistones forms a condensed structure termed chromatin. Highly condensed chromatin is known as heterochromatin and 9 less condensed regions of chromatin are known as euchromatin. Euchromatin has been shown to often be the sites of active RNA synthesis (transcription). Nucleosomes are formed when DNA wraps around histone octamers (1.75 turns per octamer). The nucleosomes can be dissociated with high salt and histones can be dissociated with denaturing agents. 10 Histone H1 binds to the outside of the nucleosome and assembles chromatin into 30nm solenoid structure- heterochromatin. 100 kilobase loops of the solenoid structures is the next level of compaction to the chromatin. Folding of DNA into chromatin and into chromosomes. Acetylation and phosphorylation of N-terminal amino acids in histones alters the histone charge from + (due to high arginine and lysine) to -. Thus, the affinity of the histone for the negatively charged phosphates of DNA is reduced. In addition, core nucleosomes are partially disassembled prior to transcription. These steps occur by modification of histones by enzymes which acetylate or phosphorylate histone amino acids. Acetylation of histones occurs strongly in expressed regions of chromatin (euchromatin). HAT= histone acetyltransferase HDAC=histone deacetylase 11 Learning Objectives: Transcription -RNA Synthesis 1. Transcription is necessary for gene expression – know types of RNA made and location in cell. 2. Describe promoter sequences- importance. 3. Organization and actions of RNA polymerases. Eukaryotic polymerase types and functions. What is an inhibitor of RNA polymerase II ? 4. Structure of eukaryotic mRNA. (Caps, poly A tail, introns, exons, etc). 5. Describe intron splicing mechanism: 5' and 3' exon/intron consensus sequence, what is alternative splicing? (example: Ig light chain mRNA production) 6. Distinguish prokaryotic and eukaryotic mRNAs 2 Dr. Cox, 2024 Transcription ( RNA Synthesis) Reading Assignment: Marks’ Basic Medical Biochemistry, Chp 14 I. Mechanism of Transcription A. Purpose: To retrieve the information for synthesis of the cell's proteins in the form of messenger RNA (mRNA) and to generate the support systems for this synthesis; i.e., transfer RNA (tRNA) and ribosomal RNA (rRNA). 1. Basic RNA types The three major classes of RNA are transfer RNA (tRNA), ribosomal RNA (rRNA) and messenger RNA (mRNA). All three classes of RNA function in the synthesis of proteins. Messenger RNA carries the information encoded in the DNA to the cytoplasm where it is translated into proteins. Transfer RNAs serve as carriers for amino acids. Ribosomal RNA is a major component of the protein synthesizing machinery, the ribosome. All of these RNAs are synthesized by RNA polymerases in the nucleus. An exception to this is the small percentage of RNA that is synthesized in mitochondria. All three classes of RNAs require processing of the initial product of transcription to yield a mature RNA molecule. These processing events take place in the nucleus. What genes are transcribed? Bacteria contains about 3000 genes and man perhaps 25,000 genes. In a given human tissue (i.e., liver) only a fraction of these genes (say 10,000) are being transcribed at any one time. Some are transcribed essentially at a constant rate all the time (constitutive proteins) others are made only in response to some stimulus (induced proteins). B. Overview of the Sequence of Steps in Transcription 1. Location of the segment of DNA to be transcribed - formation of the open- promoter complex. 2. Synthesis of RNA. 3. Termination of synthesis (not much on this) 4. Processing of the primary transcript (posttranscriptional modifications) 5. prokaryotic vs. eukaryotic As is true for all of molecular biology, much more is known about transcription in prokaryotic cells than eukaryotic. The overall process is basically the same; however, there are some important differences. 1. Promoter C. The Promoter sequence – a specific sequence of DNA that is recognized by RNA polymerase to initiate and synthesize a transcript. The sequences from prokaryotic promoters differ from eukaryotic promoters. Each RNA transcript must have a gene promoter in order for transcription to occur. 2. RNA polymerase RNA polymerase is required for all transcription. RNA polymerase separates DNA to single strand form and initiates transcription at a start site downstream of a promoter sequence. RNA polymerase is a very large enzyme complex. Eukaryotic RNA polymerase is composed of 10 protein subunits with a molecular weight of 500,000 daltons. RNA polymerase adds one ribonucleotide at a time, copying the template DNA. Promoters have specific, short, conserved DNA sequences upstream of the transcription start site. This is a eukaryotic transcription unit. A general picture of a eukayotic promoter. Most eukaryotic genes have enhancer sequences- enhancers do not exist in prokaryotic cells. We will discuss enhancers more in the gene expression notes. Basal RNA transcription factors and RNA polymerase. These factors activate RNA polymerase. TBP is the TATA box binding protein. TFs are transcription factors, proteins required for transcription. With the above proteins and RNA polymerase one would expect basal transcription only from a promoter. There are 3 major RNA polymerases in eukaryotic cells, RNA pol I,II,III. RNA polymerase I, nucleolus, transcribes ribosomal RNA. RNA polymerase II, nucleoplasm, transcribes hnRNA (precursor RNA for messenger RNA) RNA polymerase III, nucleoplasm, small RNAs (tRNAs, snRNA etc.) RNA polymerase inhibitor This is the structure of a-amanitin, a selective RNA polymerase II inhibitor from Death’s Head Mushrooms (Aminita sp.) It is very toxic, as small quantities will shut down protein synthesis in the liver, which leads to death in a couple of days. Alpha -amanitin There are 3 basic modifications to most eukaryotic messenger RNAs. 1. Capping. The following is a 5’- Cap structure found on all mRNA transcripts. Note the methylguanosine which is added on as a triphosphate to the first ribonucleotide of the mRNA transcript. 5’Caps confer stability to the 5’ end of the message. They also function in translation of the message. Some caps differ in their degree of methylation. Note the methyl group is added in the form of S- adenosylmethionine. (SAM). 2. Polyadenylation or poly A tails occurs for most mRNAs and is important for message stability. Over time the polyA tail will get shorter in the cell due to degradation from the 3’-end of the message. 3. Splicing. This is the structure of a typical hnRNA showing the conserved sequences at the exon-intron junction. The introns are exactly spliced out by molecular complexes known as spliceosomes. The following figure shows the general mechanism of intron splicing. Know that small nuclear RNAs are involved in splicing- the U1-U6 are different small nuclear RNAs associated with ribonuclear proteins (brown spheres). A typical processing and splicing of a mRNA. All introns must be removed before the spliced message can leave the nucleus. Example of alternative splicing- Antibody messenger RNAs. The kappa light chain messages are alternatively spliced to result in a specific J (join) fragment in a given messenger RNA. Organization of a “typical” chromosome- note highly and moderately repetitive sequences. The genes specifying unique messenger RNAs (mRNAs) are located randomly along the chromosomes with sometimes huge regions of non-coding DNA sequences. HIV life cycle involves both spliced and unspliced messenger RNA. The unspliced messages code for structural proteins and enzymes of the virus. The spliced messages are for regulatory viral proteins. Differences between prokaryotic and eukaryotic messenger RNAs Messenger RNA - Function in prokaryotes - Function in eukaryotes -Polycistronic message -Monocistronic -Message mainly protein -Introns and exons coding -mRNA must be processed -Message is short-lived -Long lived -Message is immediately translated. 1 Learning Objectives Dr. Cox Translation (Protein synthesis): Understand and be familiar with the following topics. 1. Structure and function of tRNA. a. Attachment of amino acids. b. Significance of tRNA domains. c. Antiparallel binding to mRNA. d. Isoacceptors. 2. Amino acid acyl tRNA synthetases. 3. Ribosome structure: prokaryotes and eukaryotes. 4. Mechanism of initiation: factors, recognition of start codons: AUG methionine.,P-site. 5. Elongation: GTP, EF-TU and EF-G (prokaryotic) ; EF1, EF2 (eukaryotic), peptide bond formation. 6. Termination mechanism. Releasing factor. 7. Differences between prokaryotes and eukaryotes message translation. 8. Signal peptide and stop transfer sequences in eukaryotes and their significance. 9. Inhibitors of protein synthesis, mechanisms (eukaryotic vs, prokaryotic) 2 Dr. Cox, 2024 Protein Synthesis Reading Assignment: Marks’ Chp 18. I. Protein Synthesis A. Overview: The following are the main participants of protein biosynthesis: mRNA, tRNA ribosomes, and GTP. Three groups of assistants are also required: initiation factors, elongation factors and termination factors. 1. The overall process in prokaryotes and eukaryotes is similar: some differences will be discussed. 2. The messenger RNA is translated from the 5′ end to the 3′ end. 3. The protein is synthesized from the amino terminal to the carboxyl terminal. 4. A sequence of three nucleotides in the mRNA codes for one amino acid. 5. Each ribosome contain a P (peptidyl) site and an A (acceptor) site. 6. There are five stages of protein synthesis. a. Activation b. Initiation c. Elongation d. Termination e. Posttranslational modifications 7. The process in brief: Processed mRNA enters the cytoplasm where it associates with a ribosome (a particle composed of protein and RNA which is organized into 2 major subunits). The mRNA is aligned on the ribosome so that the first codon of the mRNA will be “read” first. A transfer RNA molecule (bearing the amino acid coded for in the first codon) binds to this codon in the mRNA and tRNA bearing the amino acid coded for the next amino acid binds to the second codon. The two amino acids are joined by a peptide bond. The mRNA codons continue to be read and the correct amino acids joined and the proteins grows from the amino to the carboxyl terminus. Releasing factors help to terminate protein synthesis. For secreted proteins, endoplasmic reticulum targeting is determined by the presence of an N-terminal signal peptide. 3 B. Structure and Functions of tRNA- tRNAs are cloverleaf in structure with 2d folding and about 80 nucleotides in length. Amino acids are attached to the 3’end of the tRNAs. The 3d structure of the tRNA molecule is L-shaped with side-arms folded over. Codon-anticodon hydrogen bonding is how the specificity is achieved for the correct amino acid placement during protein synthesis. 4 C. The Genetic Code is the language of life which is nearly universal for all forms of life. In about half of the cases for the codons, the first two letters of the codon specify the amino acid. 5 D. Ribosomes- large and small subunits. The ribosomes are comprised of small and large subunits, each of which contain both rRNA and many proteins. The importance of this for protein synthesis is that small and large subunits dissociate following protein translation of an mRNA. 6 E. Aminoacyl tRNA synthetases (20 different)- one for each amino acid. The synthetases require the equivalent of 2 ATPs because ATP is converted to AMP in attaching 1 amino acid to the 3’end of the tRNA. F. Reading the genetic code. Three reading frames but always start with AUG. 7 Four different codons for alanine- 3rd base is wobble position. More than one codon can be read by most tRNAs because base pairing is imperfect at the 3rd base of the codon. G. Initiation requires several initiation factors in addition to Met-tRNA and mRNA on the small ribosomal subunit. The large ribosomal subunit joins to complete initiation. 8 H. Three major events happen during elongation- 1) delivery of tRNA by eEF2, 2) peptide bond formation, and 3) translocation of the ribosome one codon towards the 3’end of the message. Two of these events require GTP hydrolysis for the elongation factors to work. Elongation (here with prokaryotic factors)- note: Two GTPs are hydrolysed for each amino acid added. 9 Peptide bond formation during elongation. Note: No energy is required for this step however a catalytic group required for the reaction is provided by a specific Adenine base of the ribosomal RNA! 10 I. Termination occurs at stop codons where release factor (RF) causes hydrolysis of peptide chain and ribosome disassembles. J. Multiple ribosomes can simultaneously translate the same mRNA- called polysomes. Note: the ribosome disassembles following reaching a stop codon so the process can begin again. 11 K. Secreted proteins have signal peptides which bind to signal recognition particle (SRP) and dock to a receptor on the ER membrane. The secreted protein is translated through a pore in the membrane and enters into the ER lumen. Signal peptidase removes the signal peptide. Membrane resident proteins have one or more stop transfer sequences that are hydrophobic for the membrane stretch and anchor the protein into the membrane. 12 L. Some antibiotics are protein synthesis inhibitors that selectively inhibit prokaryotic ribosomes. They are derived from fungi and other microorganisms. END 13 14. The Biochemistry of Cellular Organelles Dr.James Cox 2024 Learning objectives : 1. Describe the structure of the ER and what are its functions. 2. Describe the proteins involved in endocytosis. 3. Describe the SNARE proteins in vesicle fusion. What is the function of NSF/SNAP in fusion? 4. Describe structure and function of lysosomes. What are the lysosomal storage diseases? 5. Describe autophagosome formation. How is it regulated and what disease is linked to it? Outline: 1. Endoplasmic reticulum 2. Endocytosis 3. Vesicular trafficking 4. Lysosomes 5. Autophagy 1. Endoplasmic reticulum A. Structure : - a. Complex network of tubules and sheet-like regions - - b. Structure of the ER is maintained by proteins including reticulons, REEPs (receptor expression enhancing proteins), and atlastins to regulate morphology - - c. Kinesin 1 and dynein are microtubule motor proteins which bind to the ER to drive formation of new tubules and remodel network. Fig. 1 The endoplasmic reticulum. Note that the ER begins at the nuclear membrane and continues out with sacs lined with ribosomes and proceeds into smooth ER with many tubules and some sheets. Fig 2. The rough ER is shown on the right with ribosomes lining the sacs or cisternae. The shape of the many tubules is produced by the insertion of proteins into the outer layer of the ER membrane producing membrane curvature of the tubule. Fig 3. The REEP proteins help bring about vesicle membrane curvature by partially inserting into the outer membrane leaflet. (B). Fig 4. ER tubules (and also transported vesicles) tether to kinesins and dyneins to move along microtubules to create a dynamic structure. The Golgi complex Vesicles which bud from the ER carry proteins to the Golgi complex. The Golgi complex is a series of flattened sacs or cisternae surrounded by spherical vesicles. It is divided into cis, medial and trans compartments, each of which contain different enzymes for protein modifications. Vesicles containing mature proteins are split off from the Golgi and destined for secretion from the cell by the process of exocytosis. Fig. 5. The Golgi Complex. The cis- face of the Golgi is towards the nucleus and the trans- face is towards the plasma membrane. Transport vesicles which leave the Golgi fuse with the plasma membrane so secretion of the vesicle contents can occur. B. Function Three main functions of the ER are: 1. Secreted and membrane protein site of synthesis with some post-translational modifications. 2. Calcium storage and signaling. 3. Lipid biosynthesis compartment. 2. Endocytosis Endocytosis is involved in nutrient uptake, cell adhesion and migration, signaling,pathogen entry,synaptic transmission,receptor downregulation , antigen presentation,mitosis, and growth and differentiation. Fig. 6 General view endocytosis from the membrane of a cell. Endocytosis is mainly to deliver extracellular material to the lysosome. Fig. 7 Endocytic pathway. Fig 8 Clathrin (A), dynamin and AP2 (B). Fig 9. Dynamin action- vesicle pinching off plasma membrane. Formation of the clathrin “basket” also requires an intermediate protein complex, AP2 (Adaptor Protein 2) which links clathrin to receptor proteins aggregated in the vesicle. Fig. 10 Two views of clathrin mediated endocytosis. Fig 11 Side view of clathrin- mediated endocytosis. 3. Vesicle trafficking and fusion After uptake into cells, vesicles must fuse with various other membrane compartments such as early endosomes. Both vesicles that fuse have proteins on their surface called SNAREs. There are two major families of SNAREs- v and t (vesicular and target SNAREs. Vesicle fusion requires formation of trans SNARE complex – v SNARE on one membrane and t SNARE on another membrane. Binding of the SNARE complexes drives membrane fusion of the two membranes. NSF (N-ethylmaleimide-sensitive factor) with ATPase activity is also required for membrane fusion. SNAP (soluble NSF attachment protein) regulates ATPase activity of NSF. NSF/SNAP complexes binds to SNAP receptors (SNAREs). The role of NSF (ATPase)/ SNAP is to disassemble SNARE complexes for new rounds of vesicular transport. Fig 12 (A) Binding of SNAREs between vesicle and target membrane. (B) Rab GTPases Another layer of regulation is by the Rab GTPases. Rab GTPases cause transport vesicles to bind to proper target membranes. For example, Rab 5 is required for early endosome fusion. PI 3-kinases are also essential for endocytosis and endocytic recycling. EEA1 (early endosome antigen 1), a Rab effector molecule, is also required for fusion of membranes. It interacts with v- and t- SNAREs. So, membrane fusion in the early endocytic pathway is carried out by Rab 5 GTPase and 3-phosphoinositide. EEA1 mediates membrane tethering as fusion occurs with SNARE and NSF/SNAP docking. Fig 13. Tethering of v to t vesicles by EEA1. Rab5 is involved in fusion. 4. Lysosomes Lysosomes are composed of an acid lumen and a layer membrane formed by a phospholipid bilayer. The acidic lumen contains over 60 hydrolytic enzymes. These enzymes are synthesized in the ER and modified in the Golgi where a mannose-6-phosphate is added to target proteins to lysosomes. On the membrane of lysosomes is a H+ATPase (v-ATPase) that hydrolyses ATP to transport protons into lysosomes to decrease pH. Also in the membrane are lysosome-associated membrane proteins (LAMPs) which are involved in phagocytosis, autophagy, and lipid transport. There are also membrane bound proteins and peptides that are involved in trafficking and fusion of lysosomes to other vesicles. Fig 14. Lysosome structure Lysosomal storage diseases These are a group of diseases that involve the enzymatic breakdown of glycosphingolipids in the lysosome. Failure to break down (catabolize) certain glycosphingolipids leads to swelling and damage to lysosomes which leads to massive damage to certain organs depending on the severity of the enzyme depletion and the exact enzyme which is deficient. As an example, Tay-Sachs disease leads to psychomotor impairment, delayed mental development, hepatosplenomegaly, and death within the first few years of life. There are also a number of sphingolipidoses - diseases which involve other lysosomal enzymes but also often with severe outcomes. Table Lysosomal storage diseases. Be familiar with names of different diseases, you need not know the enzymes associated with the diseases. 5. Autophagy Conserved catabolic process that involves formation of double-membrane vesicles called autophagosomes. Autophagosomes engulf cellular proteins and organelles for delivery to the lysosomes for breakdown of contents. Autophagy is induced by diverse signals and cellular stresses such as amino acid starvation. Formation and turnover of the autophagosomes involves autophagy related (ATG) genes. The process can be broken down into stages : initiation, nucleation and expansion of membrane, closure and fusion with lysosome. Autophagy Stage I Initiation begins with activation of ULK complex. Stage II PI3Kinase complex plus ULK complex promotes extension of an ER membrane to form a phagophore.. Stage III Phagophore elongation with recruitment of LC3II protein (required for binding of target to be degraded to autophagosome membrane) Stage IV –target to be degraded is bound inside autophagosome. Stage V- lysosome fusion to autophagosome. Fig 15 Autophagy Overview of autophagosome formation. Focus on ULK complex, PI3K complex and phagophore formation. Also, LC3 is bound to the interior of phagophore membrane. Regulation of Autophagy Upon insufficient ATP in the cell, AMP-activated protein kinase (AMPK) is activated to induce autophagy. However, target of rapamycin (mTOR) negatively is involved in autophagy regulation as well. Activation of mTOR inhibits autophagy and under the lack of growth factors and/or amino acids, autophagy is triggered due to the inhibition of mTOR. Autophagosomes stained with MDC monodansylcadaverine in 24 hr. serum starved B16F10 melanoma cells. Diseases involving autophagy There are actually a number of important disease that involve autophagy. We are going to focus only on Alzheimer’s type dementia. Alzheimer’s disease (AD) is the most common type of senile dementia. It involves the formation of senile plaques (β- amyloid ) and neurofibrulary tangles (Tau protein). The role of β amyloid in AD pathogenesis has not been established yet. β- amyloid is formed by proteolytic cleavage of the β-amyloid precursor protein (APP). Autophagy is involved in APP degradation in vesicles. Autophagy impairment in AD is associated with the accumulation of large numbers of immature autophagosomes. (see Fig 16) Fig 16. Ultrastructure of autophagic vesicles in AD brain. The dark staining regions are actually electron-dense contents of autophagolysosomes. Cross-section of a dystrophic neurite. Fig.17 Diagram of what the cross-section in Fig 16 is illustrating. Dystrophic nerve cell. EXTRACELLULAR MATRIX Dr. James Cox 2024 Textbook: Mark’s Basic Medical Biochemistry, 5th Chap. 47 Learning Objectives 1. Explain structure of collagen (Gly,X,Y, proline rich) as well as the synthesis and post-translational modifications. 2. Describe basic types of collagen. Describe collagen IV as non-fibrillar collagen, rich in basement membranes. 3. Describe the structure and biosynthesis of proteoglycans. Know the diseases and product buildup of mucopolysaccharidoses. 4. Describe fibrillin mutations as the cause of Marfan’s syndrome. 5. Describe an integrin 6. Describe fibronectin protein and laminin as extracellular matrix adhesion proteins. 7. Describe matrix metalloproteinases (MMPs). 1 Extracellular Matrix Classes of ECM Proteins The extracellular matrix contains three major classes of biomolecules: 1. structural proteins (collagen, elastin and fibrillin) 2. proteoglycans 3. specialized adhesion proteins like fibronectin, and laminin Figure 1: Interaction of proteoglycans with other extracellular matrix proteins. A. Fibrous proteins 1. Collagen a. Collagen forms a family of fibrous proteins produced by a number of cell types, one major type being fibroblasts. There are 28 types of collagen known. b. Collagen type I is the most abundant protein in the body. c. Collagen (I) is made of 33%glycine, 21% proline and hydroxyproline as well as a number of other different amino acids. Collagen Structure a. Collagens have a helical structure with (Gly-X-Y) repeats. 2 b. Fibrillar collagens have a triple helical structure throughout most of the molecule. c. Other collagen types have only part of their structure as triple helical. Figure 2. Helical structure of collagen. Triple helical on the right. Types of Collagen a. Fibrillar – Types I,II,III (90% of collagen is fibrillar) b. Non-fibrillar – Types IV,VIII,X Type IV(non-fibrillar) is a major component of basement membranes (see later). c. Others. Synthesis of Collagen a. Collagen is synthesized as a preproprotein which means the pre- part is a signal peptide which destines the protein for the ER and secretion. b. The pro- form contains short N and C terminal extensions that are removed during collagen processing. c. Post- translational modifications to collagen include hydroxylation of some prolines and lysines. (Note: this is the only source of hydroxyproline in the body). This step requires vitamin C. 3 d. Crosslinking of fibrils occurs between certain lysines. This adds great strength to collagen as it assembles into fibers. e. Collagen fibrils are arranged into fibers in the extracellular matrix. Figure 3. Collagen synthesis Scurvy - clinical Scurvy manifests as bleeding gums as well as poor wound healing. If untreated it can result in tooth loss. Scurvy is due to a deficiency in vitamin C which is required for proline hydroxylation during collagen synthesis. Reduced proline hydroxylation weakens collagen structure. Osteogenesis imperfecta - clinical a. Genetic disease with a defect in collagen production. b. Two different types are known with the second type forming an unstable collagen due to a missense mutation. 4 c. Osteogenesis imperfecta can be treated with bisphosphonates which inhibit osteoclast activity. 2. Elastin a. Elastin is high molecular weight protein which has elastic properties. b. Lysines crosslink (2, 3, or 4) between the elastin monomers to create a fibrillar molecule. c. Elastin is a very stable molecule with a half-life of 70 years. 3. Fibrillin a. Fibrillin is a large glycoprotein (350 kDa). b. Fibrillin is a structural component of microfibrils, 10-12nm fibers found in many tissues. c. Fibrillin is secreted into the extracellular matrix. Marfan Syndrome - clinical a. Due to mutations in the fibrillin gene. b. Most patients are tall and gangly and exhibit long digits (arachnodactyly- spider-like fingers) c. Hyperextensibility: Joints; cardiovascular system leading to dilation of the ascending aorta). B. Proteoglycans a. Core protein. b. Covalently linked glycosaminoglycan chains- many per core protein. c. Glycosaminoglycans are repeating disaccharides- hexosamine and uronic acid. 5 Figure 4. Basic structure of a proteoglycan Proteoglycans a. Proteoglycans are found in: i. synovial fluid of joints ii. vitreous humor of the eye iii. arterial walls iv. bone and cartilage b. Major component of the ECM which forms a gel. Types of Proteoglycans : There are seven major types of glycosaminoglycans which differ in their repeating disaccharide units: Hyaluronic acid Chondroitin sulfate Dermatan sulfate Heparin Heparan sulfate Keratan sulfate I and II 6 Figure 5. Hyaluronic acid structure. Synthesis of Proteoglycans a. Once core protein enters the lumen of the ER, synthesis starts with the attachment of a sugar to a serine residue. b. UDP-sugars are the substrate for proteoglycan synthesis. c. Specific UDP-sugar glycosyltransferases carry out the sequential transfer of specific monosaccharides. Figure 6. 7 Sulfation of Proteoglycans a. Sulfate groups are attached through N- or O- sulfation. This occurs after the sugars have been added. b. 3’ –phosphoadenosine 5’-phosphate (PAPS) provides the sulfate group. Figure 7. 8 Breakdown of Proteoglycans Clinical disorders of proteoglycan breakdown are known as mucopolysaccharidoses. II. Integrins a. Cell membrane anchored adhesion receptors to the extracellular matrix. b. 24 different integrins are known which are comprised of alpha/beta dimers. c. Activation of integrins allows them to participate in cell migration and adhesion. III. Adhesion Proteins in the Matrix Fibronectin a. Soluble glycoprotein found in large amount in the extracellular matrix. b. Consists of two peptide chains (each 230 kDa) joined by disulfide bonds near their C-terminus. c. Contains seven domains binding to heparin, fibrin, collagen, DNA, and cell surfaces. 9 d. Cell fibronectin receptor belongs to the family of transmembrane integrin receptors. Figure 8. Structure of fibronectin. Laminin a. Consists of three distinct elongated polypeptide chains (A, B1, and B2) (850 kDa) linked together to form a cruciform shape. b. Has a binding site for type IV collagen, heparin, and integrin. c. Basal lamina have three proteins – laminin, entactin, and type IV collagen. 10 Laminin Structure : Figure 9. Laminin. IV. Matrix Metalloproteinases a. MMPs are proteolytic enzymes which can degrade proteins in the extracellular matrix. b. More than 20 MMPs are known c. MMPs are tightly regulated in the ECM and are involved in protein turnover of the matrix. 11 12 13 14