DM308_Lecture_4_2021.pdf
Document Details
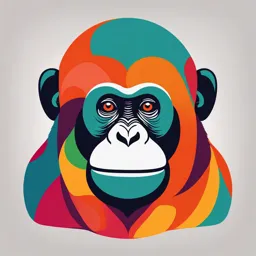
Uploaded by SafeDiscernment
University of Strathclyde
2021
Tags
Related
- Corrosion Section 6 - YILDIZ Technical University
- SERAMIK.pdf
- 01 CERAMICAS-INTRODUCCION_ecda44455a8b7e9b2ecdef8d6d2d25d8.pdf
- Introduction to Material Science and Engineering PDF
- TOPIC 02_Portland Cement, Ceramics, and Glass Industries.pdf
- Dental Ceramics_ Fracture Mechanics and Engineering Design PDF
Full Transcript
DM308 Production Techniques 2 Lecture 4 – Properties and processing of ceramics Dr. Vassili Vorontsov Department of Design, Manufacture and Engineering management, Faculty of Engineering, University of Strathclyde [email protected] What are ceramics? Ceramics are compounds of non-metall...
DM308 Production Techniques 2 Lecture 4 – Properties and processing of ceramics Dr. Vassili Vorontsov Department of Design, Manufacture and Engineering management, Faculty of Engineering, University of Strathclyde [email protected] What are ceramics? Ceramics are compounds of non-metallic elements with other elements (metallic or non-metallic). [keramikos = pottery in Greek] The bonding between atoms is usually either ionic or covalent. Ceramics can be crystalline, semi-crystalline or amorphous. Mohenjo-daro, Indus valley in present day Pakistan. Founded in 2500BC. Brick was the main building material. Fired clay tablet with Babylonian cuneiform script. Ancient Greek pottery. Modern ceramic products Traditional ceramics Structural (Construction) Whiteware Technical ceramics Refractories Structural Functional Major ceramic compounds Oxides Carbides Nitrides Borides Silicides Processing of traditional ceramics Processing of conventional involves (1) powder preparation (2) mixing of powders with water (or other binder) to make wet clay and shaping into a “green” ceramic (3) drying of product to make a “brown” ceramic (4) firing at high temperature to consolidate it into the final product. >>Note the microstructural and volume changes during the production stages. Processing of technical ceramics Powder Material preparation Raw materials Pulverising Mixing Filtering Granulating Plasticising Spray drying Moulding /forming Dry pressing Injection moulding Extrusion Isostatic pressing Green machining Milling Turning Drilling Cutting Sintering Binder removal Sinter firing Finishing Grinding Lapping Polishing Honing Metallising Glazing Assembly Quality control Optical examination Dimensional check Crack detection Strength testing Technical ceramic production relies on the same principles as traditional ceramic production, but is usually more refined and involves a greater number of production stages with a greater degree of control. The quality of the raw material preparation is paramount. Processing of technical ceramics Coarse material preparation Jaw crusher Gyratory crusher Roller crusher Hammer mill Fine powder preparation Ball milling Roller milling Impact grinding Fine ceramic powder can be produced from crushed minerals using one of the above methods. The resulting powders can then be sieved into different size fractions. Ceramic additives There are four main types of additives that can be mixed in with the ceramic powder in order to adjust the properties of the raw: Binder – ensures the cohesion of the ceramic powder in the green parts. Dispersant – decreases the viscosity of the mixture and increases the volume concentration of ceramic particles. Plasticiser – modifies the flow behaviour of the binder and hence the raw ceramic mixture. Lubricant – minimises friction and adhesion between the mixture and the mixture parts. Sintering When ceramics are fired, the powder particles within undergo a process known as sintering, whereby the particles bond and coalesce over time, the porosity decreases and thus density of the ceramic increases. Stages of sintering During sintering necks begin to form between particles that are touching. At this stage the ceramic is still very porous. As sintering progresses the necks thicken to form defined grain boundaries while the network of pores gradually closes. Eventually a dense granular ceramic structure is obtained with some minor distribution of closed pores at the the prior particle boundaries Continuing the sintering process can further reduce this porosity and obtain a very high density ceramic product. Sintering mechanism The free surfaces of the powder particles have an interracial energy and sintering acts to minimise the overall surface area and hence overall surface energy. Material is transported from convex regions with high vapour pressure to concave neck regions with low vapour pressure where it condenses. Within the particles the opposing diffusion of atoms and vacancies helps close the pores. Plastic flow can also help transport material during sintering, especially if an external stress is applied during the firing of the ceramic. Ceramic forming processes Powder compaction: axial pressing, cold isostatic pressing, hot isostatic pressing Plastic forming: extrusion, injection moulding Casting: slip casting, tape casting, gelcasting, freeze casting, slurry dipping Coating: plasma spraying, physical vapour deposition, chemical vapour deposition Powder compaction Dry and semi-dry axial pressing Comparatively simple shapes can be made simply by pressing a dry or semi-dry ceramic powder to form the green product. This simply involves pressing it into a die or mould with sufficient pressure. Cold isostatic pressing (CIP) During isostatic pressing the ceramic powder is placed into flexible or deformable mould. The mould is then placed in a pressure chamber which is filled with an inert gas (usually argon) at a very high pressure. The very large nearhydrostatic pressure compresses the powder uniformly into a “green” preform. The possible geometries are limited with this process due to the flexible mould required, so additional machining may be necessary. Flexible elastomer moulds Hot isostatic pressing (HIP) During hot isostatic pressing the pressure chamber heated, allowing for simultaneous sintering. This allows for a very high degree of powder consolidation. However the mould material has to withstand the high temperatures and further limits the possible geometries. The requirement for high temperatures and pressures means that particular attention must be paid to ensuring safety. N.B. HIP-ing is a very good method for reducing the porosity of products produced using additive manufacturing techniques. Casting Slip casting In slip casting, a liquid ceramic mixture called “slip” is poured into a plaster mould. The plaster absorbs moisture from the adjacent slip making it more viscous than the original mixture. Pouring out the slip leaves behind the viscous layer which is allowed to dry further to a “leather dry” state. The green ceramic is then removed from the mould for subsequent trimming and firing. Slip casting allows mass production of ceramics with very complex relief. Tape casting Tape casting allows the production of thin ceramic tapes or sheets from a ceramic slurry. The slip flows from a hopper onto a carrier film and the thickness of the ceramic tape is adjusted by the height of the doctor blade. The tape is then dried and spooled up for further shaping and subsequent sintering. Gel-casting In gel-casting the ceramic powder is mixed with water and a monomer binder that undergoes a polymerisation reaction. The polymer forms a gel which binds the ceramic particles. This gel can be used to fill a variety of complex moulds. The gel is then dried into a solid and burned out prior to sintering. Gel-casting Gel-casting is suitable for highly advanced technical ceramic forming and allows a high degree of precision and complex geometries. Sol-gel processing Freeze casting Freeze casting uses a suspension of ceramic particles in water. The water is then frozen in a carefully controlled manner so that the ice grows as regularly spaced lamellae. The suspended ceramic particles settle between and around the ice lamellae. The ice is then allowed to sublimate at low pressure leaving a porous ceramic pre-form behind. The preform then undergoes sintering. Freeze casting allows the production of highly porous ceramics for filters, catalysts and biological/medical applications. Plastic forming Plastic forming Clays and other plastic ceramic mixtures can be extruded, injection moulded and jiggered to form a variety of geometric shapes. Jiggering Extruded ceramics Extrusion Injection moulded part Coating Physical vapour deposition Physical vapour deposition can be used to coat ceramics onto substrate materials. PVD is carried out in a vacuum, whereby the ceramic is heated so that it evaporates and is transported onto the substrate where it condenses. This allows for very thin coatings, several nanometres thin if desired. PVD can also achieve low porosity and a refined surface finish. Plasma sprtaying Plasma spraying can also be used to make ceramic coatings. The ceramic powder is fed through plasma created by passing an electric arc through an jet of high pressure argon. The particles melt in the plasma and the jet transports them onto the substrate. It is important that the substrate is able to withstand the high temperatures involved. The molten droplets form splats on the substrate surface and solidify. Plasma spraying is faster than PVD. but gives a much rougher and more porous coating. Properties of Ceramics Melting points Technical ceramics have comparatively high melting points which generally surpass most alloys and all polymers. Maximum use temperatures Technical ceramics offer the highest maximum use temperatures, due greater resistance to high-temperature oxidation/corrosion. Stiffness and thermal expansion Most technical ceramics have comparable or greater stiffness and lower thermal expansion than most metal alloys. Hardness and wear resistance Lower is better Most technical ceramics are considerably harder than metal alloys and polymers. They also offer excellent wear resistance. ← Higher is better Strength and fracture toughness Ceramic offer reasonable strength and fracture toughness. However, this often implies compressive loading and high-quality manufacturing. Density Technical ceramics have low density and as a result have the highest specific stiffness and highest specific strength. Note: CFRP is a ceramic-reinforced polymer. Thermal and electrical conductivity Ceramics are mostly electrical insulators. They have a high to moderate thermal conductivity. Properties summary Good: Good high temperature capability High hardness High stiffness Good strength Electrically insulating (some exception exist) Good fracture toughness High wear resistance Bad: Brittle failure mode Notch sensitive (surface defects can lower toughness and tensile/bending strength) Can be difficult to process (due to high temperatures and high hardness) Oxides Alumina - Al2O3 High hardness Moderate strength Electrical insulator Thermal insulator Bioinert Uses: Refractories and crucibles Abrasives and cutting tools Automotive spark plug insulators Bioceramics for orthopaedic implants Ballistic protection (armour plates) High temperature engineering components Zirconia - ZrO2 Moderate hardness High strength High toughness Thermal insulator Bioinert Uses: Crucibles and refractories Heat engine components Dies and punches Dental implants and prostheses Ceramic knives Thermal barrier coatings Zirconia phase transformations Zirconia can have one of three crystallographic structures depending on the temperature. The resulting volume changes can lead to processing problems when manufacturing precision components. It also complicates the use of zirconia at high temperatures. Stabilised zirconia Zirconia can be blended with other oxides to stabilise a particular crystallographic structure. Yttria (Y2O3) is often added to stabilise zirconia in its cubic form. This form is known as yttriastabilised zirconia or YSZ. It is essentially a ceramic alloy. Zr:O ratio in ZrO2 is 1:2 Y:O ratio in Y2O3 is 1:1.5 Adding yttria therefore creates oxygen vacancies in the YSZ. At high temperatures YSZ can conduct oxygen vacancies (=electric current). YSZ is thus used for fuel cells and oxygen sensors. (See lecture 2) Uranium dioxide (UO2) Most nuclear reactors use uranium dioxide as the fuel material rather than metallic uranium. The first reason is that UO2 has a higher melting point than metallic uranium. (i.e. safer to use) Secondly it does not burn since it is already oxidised. (i.e. safer to use) Uranium dioxide is compressed and sintered into ceramic pellets which are packed into neutron-transparent zirconium alloy tubes (fuel pins), which then form the larger fuel assemblies. Carbides Tungsten carbide - WC Very high hardness (2600HV) High melting point (2870°C) Electrically conductive Alloys with cobalt to tune properties Prone to high-temperature oxidation Uses: Cutting tools and dies for machining Mining and foundation drills Walking pole tips and tire inserts Balls for ballpoint pens Surgical equipment Jewellery Silicon carbide - SiC High-temperature strength Forms protective oxide (SiO2) at high temperatures Sublimates rather than melts (2700°C) Good wear resistance Semicoducting Uses: Abrasive and cutting tools Ballistic protection (armour plates) Foundry crucibles High performance brake discs Furnace heating elements LEDs, diodes, transistors Titanium carbide - TiC Extreme hardness (3200HV) High melting point (3160°C) Outstanding wear resistance Currently only used as coatings or cermet composites, i.e. no bulk form Uses: Hard surface coatings for tools Coatings for watch parts TiC Cermet composites E.g. rock crusher hammers on the left. Nitrides Boron nitride - BN Has multiple crystalline forms: cubic (c-BN), hexagonal (hBN) and others. c-BN has second-highest hardness after diamond (7750HV) Good heat conductor Uses: h-BN high-temperature/vacuum lubricants h-BN crucibles heatsinks/heat spreaders c-BN grinding wheels and abrasives Boron nitride nanotubes Boron nitride can be synthesised into nanotubes (BNNTs) with a similar structure to carbon nanotubes (CNT). Unlike CNTs, BNNTs do not burn in air. BNNTs also exhibit some interesting defect healing properties. Thus BNNTs open some interesting possibilities for novel composites. Silicon nitride - Si3N4 Si3N4 offers moderate properties at a moderate cost, which can often be the deciding factor for selecting it for a given application over other ceramics and/or metals. Hardness (1600HV) Decomposes above 1900ºC Uses: Bearings High-temperature structural parts ^(e.g. automobile/rocket parts) Bioceramics for orthopaedic implants (e.g. spinal fusion) Metalworking cutting tools (cheaper than WC) Titanium nitride - TiN High hardness (2100HV) Low friction properties Non-toxic / Biocompatible Nice gold colour Combinable with other nitrides Uses: Wear-resistant tool coatings ^(good edge retention and low wear) Surgical tool and bone pin coatings Motorcycle suspension forks Aesthetic coatings in plumbing Ceramic alloys Clays Clays have been used by people for millennia for buildings, pottery and objects of art. Clays are very fine-grained rock material that typically combine a range of minerals and some organic matter. A commonly occurring clay mineral is Kaolinite - Al2(OH)4Si2O5 though several others exist. Clays also contain some amounts of quartz (SiO2) and other metal oxides. (E.g. terracotta contains red iron oxide Fe2O3) When clay is fired, the clay minerals decompose into silica Al2O3 and alumina SiO2, which can form a range of alloys. (See phase diagram on next slide). Open pit clay mine Kaolinite Alumina-silica phase diagram Liquid Temperature (°C) L+Mullite L+SiO2 L+Al2O3 Liquid+Mullite Mullite+Al2O3 SiO2+Mullite Mullite Composition (wt.% Al2O3) 3Al2O3·2SiO2 Porcelain microstructure The variability of clay composition can produce a wide variety of microstructures after firing. Note the three mullite morphologies below. Mullite, porcelain and mullite ceramics Mullite is also known as porcelanite and is largely responsible for the properties of porcelain. Kaolinite clays form mullite when fired. If the processing and composition are right, the mullite will form a microstructure of interlocking needles, which imparts considerable strength to the porcelain. Mullite-containing ceramics don’t have to be made from clay, but can be produced using pure silica and alumina. Typical products include fire bricks and other refractories. Porcelain is also used as the outer layer on zirconia dental implants. AlON AlON ? AlON High hardness (2100HV) High melting point (2150ºC) Transparent when polished Polishing improves fracture toughness considerably Uses: Currently ALON ceramics are used predominantly in military applications such as transparent armour, military aircraft sensor windows and missile domes. Perhaps future uses can be found in the civil sector too? 1.6 inches of AlON offers more protection than 3.7 inches of glass/polymer laminate armour. This allows considerable weight savings. SiAlONs High hardness (1700HV) Maximum use temperature (1200ºC) High chemical stability and resistance to thermal shock Phosphorescent in UV light when doped Uses: Tubes and ladles for non-ferrous metal processing Cutting tools / lathe inserts Tube forming rollers Coloured phosphors Hydrocyclones for filtering out dust/sand from oil and gas Summary Ceramics are an important family of materials with some outstanding properties: specifically high hardness and good hightemperature properties. Processing of ceramics usually involves: powder preparation, forming/shaping, drying, firing/sintering and finishing. Ceramic forming processes are divided into: powder compaction, plastic forming, casting and coating. Ceramics can be used both in their pure form as well as used to make alloys. Typical applications include: refractories and crucibles, machining tools and dies, abrasives, high temperature structural components, biomedical implants.