Diuretics: A Review PDF
Document Details
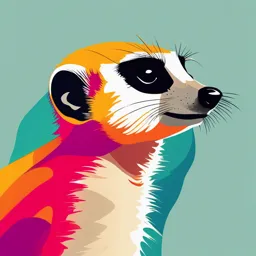
Uploaded by FlawlessRationality
David Wile
Tags
Summary
This review article explores the history and clinical use of diuretics, starting with kidney physiology and progressing through different diuretic classes and their modes of action. It then examines various clinical uses, adverse effects and implications for chronic kidney disease. The article concludes with insights into newer approaches to diuretic therapy.
Full Transcript
Review Article Diuretics: a review David Wile Department of Clinical Biochemistry, University Hospital, Aintree, Longmoor Lane, Liverpool L9 7AL, UK Corresponding author: Dr David Wile. Email: [email protected] Abstract Diuretics, in one form or another, have been around for centuries and t...
Review Article Diuretics: a review David Wile Department of Clinical Biochemistry, University Hospital, Aintree, Longmoor Lane, Liverpool L9 7AL, UK Corresponding author: Dr David Wile. Email: [email protected] Abstract Diuretics, in one form or another, have been around for centuries and this review sets out to chart their development and clinical use. Starting with the physiology of the kidney, it progresses to explain how diuretics actually work, via symports on the inside of the renal tubules. The different classes of diuretics are characterized, along with their mode of action. The clinical use of diuretics in conditions like congestive cardiac failure and hypertension, as well as some rarer, but clinically important, conditions is then examined. An account is given of the adverse effects of diuretics and how they come about. Common adverse effects like hypokalaemia and hyponatraemia are examined in some detail, and other electrolyte disturbances like hypomagnesaemia also gain a mention. Diuretic use in chronic kidney disease is examined and new guidelines that have been introduced are presented. A section on diuretic abuse is included as this is becoming an all too common clinical scenario, and the sometimes tragic consequences of this abuse are emphasized. Diuretics also find a role in the diagnosis of forms of renal tubular acidosis and this role is explored. Finally, a selection of some of the newer approaches to diuretic therapy are presented, often the consequence of the increasing development of molecular biology, and some of the novel compounds – which may be in drug formularies of the future – are revealed. Ann Clin Biochem 2012; 49: 419– 431. DOI: 10.1258/acb.2011.011281 Introduction Historical perspective The word diuretic has a Greek stem, diu (through) oyr1ih (to urinate),1 and a diuretic is defined as any substance that increases urine flow and thereby water excretion.2 Diuretics are among the most commonly used drugs and the majority act by reducing sodium chloride reabsorption at different sites in the nephron (Figure 1), thereby increasing urinary sodium, and consequently, water loss. Paintings found in the ruins of Pompeii have depictions of grapes, ivy, olives and sweet cherry – all of these have diuretic properties described in the writing of Pliny the Elder (23– 79 AD).3 A treatise published in 1788 by Joseph Plenick (1735 – 1807) lists several hundred plants, of which 115 have diuretic properties, including garlic, Chinese lantern, saffron, fennel, liquorice, sassafras and dandelion (Taraxacum officinale).4 The latter derives its name from the French ‘dent de lion’ (tooth of the lion) on account of the shape of its leaves which impart its diuretic property – probably because of that it is commonly called, in French, ‘pissenlit’, literally ‘piss in bed’.5,6 Its diuretic properties are thought to be due to potash ( potassium carbonate, K2CO3).7 From 1919 until the 1960s, the most effective diuretics, used as the mainstay of treatment, were the mercurials, but they are no longer used because of their toxicity.8 Other options during this period were osmotic diuretics like urea, mannitol and sucrose, acidifying salts such as ammonium chloride, xanthine derivatives and digoxin, which has a diuretic effect in addition to its inotropic effect. In 1937, Southworth realized that patients treated with the antibiotic sulphanilamide not only breathed deeply (they developed a mild metabolic acidaemia) but also produced an alkaline urine, with increased sodium and water excretion.9 Sulphanilamide was found to be a carbonic anhydrase inhibitor10 and by 1949, Schwartz11 had successfully treated congestive heart failure patients with sulphanilamide. Karl Beyer, having heard of Schwartz’s clinical success, began searching for and testing a range of sulphanilamide-like agents on animals.12 Substitution of a carboxy group for the aromatic amino group of sulphanilamide generated carboxybenzenesulphonamide (CBS), also a carbonic anhydrase inhibitor that increased sodium and chloride excretion. Introducing a second sulphamoyl group meta- to the first was found to increase potency, and exploration of substituted disulphamoylbenzene analogues finally led to the discovery of 6-chloro-2H-1,2,4-benzothiadiazine-7-sulphonamide-1,1dioxide (chlorothiazide), the first thiazide diuretic.13,14 It was because the original compounds were benzothiadiazine derivatives that this class of diuretics became known as Annals of Clinical Biochemistry 2012; 49: 419– 431 420 Annals of Clinical Biochemistry Volume 49 September 2012 ................................................................................................................................................ Proximal Distal Site IV Site III Site I NaCI Na+ Filtrate More hypotonic CH20 NaCI H20 NaCI tex ulla Med K+H+ Na+ NaCI Hypotonic CH20 Isotonic Cor Aldosterone NaCI ADH TC Isotonic Site II H20 NaCI Ascending limb H20 Water impermeable Collecting duct Hypertonic Figure 1 Diagram of the renal tubule showing principal sites of diuretic action. Reproduced from Lant26 with kind permission from Wolters Kluwer Pharma Solutions thiazide diuretics. Later compounds that were pharmacologically similar to thiazide diuretics but were not thiazides appeared, and acquired the name ‘thiazide-like’ diuretics (Figure 2), many being heterocyclic compounds like metolazone. The British National Formulary (BNF) currently lists 15 individual diuretics available for use in the UK but the original one, chlorothiazide, is not among them, although its derivatives, hydrochlorothiazide and benzothiazide, do feature in combination preparations.8 They are grouped into familiar categories – thiazides, loop diuretics (also known as high ceiling diuretics), potassium-sparing, osmotic and carbonic anhydrase inhibitors (Table 1). This review elaborates on each of these and some miscellaneous compounds (caffeine, alcohol and water), and finally discusses some exciting recent developments. Role of the kidney in water homeostasis Renal tubular reabsorption of filtered water occurs by osmosis, and, since the glomerular filtrate is essentially isoosmotic, depends on sodium reabsorption to create an osmotic gradient.15 After formation of a plasma ultrafiltrate in the glomerulus, the tubular fluid enters the proximal convoluted tubule, where specific transporters reabsorb sodium, chloride, bicarbonate, glucose and amino acids. About 60% of the water and most of the organic solutes are also reabsorbed in the proximal tubule.16 At the boundary between the inner and outer stripes of the outer medulla, the thin descending limb of the loop of Henlé begins. The thick ascending limb of the loop of Henlé actively reabsorbs sodium and chloride from the lumen (about 35% of the filtered sodium), but unlike the proximal tubule and the descending limb, it is virtually impermeable to water.17 Sodium chloride reabsorption in the thick ascending limb effectively dilutes the tubular fluid, so this segment is called the ‘diluting segment.’ The loop of Henlé therefore acts as a countercurrent multiplier producing a gradient of hyperosmolarity in the medullary interstitium. In the distal convoluted tubule, which connects with the diluting segment, around 10% of filtered sodium chloride is reabsorbed. Like the thick ascending limb, the membrane is relatively impermeable to water, so further tubular fluid dilution ensues.18 The final arbiter of urine composition is the collecting duct, where 2 – 5% of sodium chloride reabsorption occurs. Importantly, this is where mineralocorticoids exert their influence, especially aldosterone. Sodium is reabsorbed in exchange for potassium under the influence of aldosterone and it is here that almost all diuretic-induced changes in potassium balance occur. Water is reabsorbed through the action of the posterior pituitary hormone vasopressin (also known as antidiuretic hormone [ADH], although vasopressin is the preferred term) and the final urine to enter the renal pelvis is diluted or concentrated, achieved by the countercurrent mechanism that creates a concentration gradient from 50 mOsm/kg at the outer cortex to 1200 mOsm/kg at the inner medulla.19,20 Wile. Diuretics – a review 421 ................................................................................................................................................ CH3 H N CI CI H2N CO S O2 H2N O2S Hydrochlorothiazide Indapamide 0 CI H N CH3 HN H2N H2N N NH O2S CI NH O2S N O2S O OH CH3 Chlorthalidone Figure 2 Metolazone Structures of some ‘thiazide-like’ diuretics Transporters Absolutely germane to the understanding of diuretic action is the concept of transporters. Those that operate by having to bind more than one substance to the transport protein, then facilitating the transport of these substances, together, across the membrane are called symports (or symporters). If they transport only one substance, they are uniports,21 and others that exchange one substance for another are the antiports. The archetypal antiport is the Naþ – Kþ-ATPase, which moves three Naþ ions out of the cell for each two Kþ ions that it moves into the cell. From the elucidation of these mechanisms, a new and functional classification of diuretics has arisen. How diuretics work Table 1 Diuretics currently licensed for use in the UK Class of diuretic Examples Thiazides and related diuretics Bendroflumethiazide Chlorthalidone Cyclopenthiazide Indapamide Metolazone Xipamide Carbonic anhydrase inhibitors Acetazolamide Loop diuretics Furosemide Bumetanide Torasemide Osmotic diuretics Mannitol Potassium-sparing diuretics Amiloride Triamterine Potassium-sparing diuretics and aldosterone antagonists Spironolactone Eplerenone Potassium-sparing diuretics with other diuretics Co-amilozide Co-amilofuse Co-triamterzide Most diuretics exert their action by decreasing renal tubular sodium reabsorption, thereby reducing the luminal-cellular osmotic gradient, which limits water reabsorption and results in a diuresis. With the sole exception of spironolactone and its analogue, all the transporters that they inhibit are on the luminal surface of the tubule, so the diuretic agents have to actually ‘get there’ in order to block the symport or uniport transporter.22 This means they have to be secreted into the tubular fluid and arrive at their target destination in sufficient concentration to be useful. The process involves facilitated diffusion and in the case of loop diuretics, thiazides and the carbonic anhydrase inhibitor acetazolamide, all of which are acidic, secretion into the tubular fluid, through the organic acid pathway in the proximal tubule. Amiloride and triamterene, being organic bases, enter the tubular lumen via the organic base secretory mechanism, also in the proximal tubule. Spironolactone and other aldosterone antagonists act via a cytosolic receptor and so are delivered to their target area via the blood and the basolateral membrane. If the diuretic is very highly protein bound (.96%), then glomerular filtration is limited. Even in hypoalbuminuria, there is not enough ‘free’ drug at one time to get across. Other considerations apply as well and these will be 422 Annals of Clinical Biochemistry Volume 49 September 2012 ................................................................................................................................................ examined separately, with the diuretic or disease that influences it. This group of diverse chemical agents (e.g. furosemide and bumetanide) have one common property – they are all inhibitors of the NaþKþ 22Cl2 symport which transfers ions from the tubular lumen into the tubular cells.23 The symport is electroneutral and is activated when all four sites are occupied. The Naþ that has entered the tubular cell is pumped out into the systemic circulation by the Naþ 2Kþ-ATPase antiport located in the basolateral membrane.24 By its action, a very favourable electrochemical gradient for Naþ to enter the cell from the lumen is established because the Naþ concentration inside the cell is left low. A separate basolateral chloride channel (called CLCN) provides a basolateral exit conduit for Cl2. The availability of luminal potassium limits activity of the NaþKþ 22Cl2 symport so potassium entering the cell is recycled by the ROMK (renal outer medullary potassium) channel, which is an ATP-dependent potassium channel in the luminal membrane that not only plays an important role in potassium recycling in the thick ascending limb, but also in potassium secretion in the cortical collecting ducts of the nephron. The depolarization of the basolateral membrane sets up a transepithelial voltage difference (10 mV or so) with the lumen positive compared with the interstitial space. Because of the asymmetrical stoichiometry (3 Naþ per 2 Kþ), this voltage repels cations (Naþ, Ca2þ and Mg2þ) towards the interstitial space and drives reabsorption of these cations via paracellular paracellin-1. This region (ascending limb of the loop of Henlé) is virtually impermeable to water because unlike the proximal tubule, it does not have water channels (aquaporins, AQPs) which grossly facilitate the movement of water. Therefore, the actions of the symport remove Naþ and Cl2 but not water, effectively diluting the tubular fluid, to the extent that about 25– 35% of filtered sodium is reabsorbed here. If the Naþ 2Kþ 22Cl2 symport is blocked, then about 25% of filtered sodium would not be reabsorbed, and would remain in the tubular fluid to be presented to the collecting duct, where, under the influence of aldosterone, some Naþ would be retrieved – but at the expense of exchange for Kþ, which would be lost. The other consequence of jamming this symport would be to reduce, if not abolish, the voltage difference whereby calcium and magnesium are reabsorbed. Blocking this symport would lead to a substantial natriuresis, hyponatraemia, possibly some hypokalaemia, with hypocalcaemia and hypomagnesaemia a distinct possibility.25 The mode of action of loop diuretics, e.g. furosemide and bumetanide (Figure 3), is to block the Naþ 2Kþ 22Cl2 symport (site II, Figure 1), and it is thought that these agents bind the Cl2-binding site that lies within the symporter’s transmembrane domain.26 They owe their designation as loop diuretics to their site of action and they have acquired the synonym ‘high ceiling’ diuretics because progressive increase in dose is accompanied by an increasing diuresis (actually natriuresis because both water and CH2 NH Loop diuretics O 3 1 4 CI 5 H2N 2 COOH 6 O2S Furosemide NH(CH2)3CH3 O COOH H2N O2S Bumetanide Figure 3 Structures of some ‘loop diuretics’ solute [NaCl] are lost) – they have a high ceiling to their effect. As they block the region of the nephron that has the capacity to reabsorb the most sodium, they are the most potent and effective natriuretic compounds and so have claimed yet a third name – that of ‘high efficiency’ diuretics. These names belie the fact that they come from cosmopolitan background chemistry. Some like furosemide are sulphonamide derivatives, one was a sulphonylurea (torasemide) and the other, no longer in common use, a phenoxyacetic acid derivative, ethacrynic acid. This fell from favour because it had particularly marked side-effects of nausea and ototoxicity, although, unlike the others, it was also uricosuric. Ototoxicity is a general feature, usually dose-dependent, of loop diuretics. A transporter with a chloride channel is present in the inner ear, almost identical to the chloride channel that is part of the Naþ 2Kþ 22Cl2 symport, which is also blocked by the same inhibitors. Incidentally, mutations in the gene for the Naþ 2Kþ 22Cl2 co-transporter, called NKCC2, result in the classic form (there are variants) of a disorder named Bartter’s syndrome,27 the clinical features of which are hypokalaemia, hypercalciuria and metabolic alkalosis, similar to those seen in a patient taking a loop diuretic. Wile. Diuretics – a review 423 ................................................................................................................................................ Thiazides and related diuretics The other major group of diuretics is the thiazide group mentioned earlier and this description includes the thiazidelike diuretics (Figure 2), which are not true benzothiazides but are heterocyclic variants (e.g. metolazone) having the same pharmacological action. Their site of action is the cortical portion of the ascending loop of Henlé and the distal convoluted tubule (site III, Figure 1) where they inhibit sodium and chloride reabsorption by inhibiting the electroneutral Naþ 2Cl2 symport located there. They can only act as moderate natriuretics, conspicuously less potent than loop diuretics, because even at maximal doses, they can only inhibit, at most, 3– 5% of the filtered sodium present in the tubular fluid. Thiazides are believed to inhibit the Naþ 2Cl2 symporter by binding competitively to the chloride binding site.28 The Naþ –Cl2 symporter derives its energy from the Naþ 2Kþ-ATPase antiport in the basolateral (blood side) membrane, which maintains a low sodium concentration, in the tubular cell so there is a favourable electrochemical gradient for Naþ reabsorption from the lumen, similar to the situation in the Naþ 2Kþ 22Cl2 symporter (but without the Kþ recycling). The attachment of sodium to its binding site on the Naþ 2Cl2 symporter results in an affinity increase for Cl2 to its site on the symporter. Once the Naþ and Cl2 are both bound, the symporter undergoes a conformational charge that transfers both Naþ and Cl2 across the luminal membrane. Although sodium entry into the cell from the tubular lumen is primarily mediated by the Naþ 2Cl2 symporter, another mechanism is involved – that of the Naþ 2Hþ and Cl2 2HCO2 3 parallel exchangers. With the parallel exchangers, the dynamics differ; intracellular water and þ CO2 combine to form Hþ þHCO2 and 3 ions. The H 2 HCO3 ions are then secreted into the lumen in exchange for Naþ and Cl2, respectively. The secreted Hþ and HCO2 3 ions combine within the lumen to form water and CO2 which can re-enter the cell and repeat the cycle to promote further NaCl reabsorption. Some of the older thiazides (chlorothiazides but not bendroflumethiazide) can slightly impair sodium transport in the proximal tubule as well; not surprising, when one recalls their developmental history from sulphonamide agents with carbonic anhydrase inhibitor activity. This does not have a noticeable effect on diuresis, because any excess fluid leaving the proximal tubule is retrieved later, in the loop of Henlé (thin limb). Thiazides can also increase potassium and hydrogen ion exchange for sodium in the distal convoluted tubule, resulting in increased excretion of potassium and hydrogen ions. Unsurprisingly therefore, mutations in the Naþ 2Cl2 symporter lead to a form of inherited hypokalaemic alkalosis called Gitelman’s syndrome. The distal convoluted tube is also the main site of an effect that is independent of sodium transport – active calcium reabsorption.29 Thiazides, as well as inhibiting sodium reabsorption in this segment, also increase calcium reabsorption; the exact opposite of loop diuretics in their respective operational segment. This effect can be very useful in patients with recurrent nephrolithiasis arising from hypercalciuria, but a note of caution should be sounded because, while thiazides rarely, if ever, actually cause hypercalcaemia, they can unmask it due to other causes, in particular hyperparathyroidism, malignancy or sarcoidosis.23 Potassium-sparing diuretics In the next nephron segment, the late distal tubule and the collecting duct, there is yet another type of sodium transport mechanism, an ion channel, which used to be called the ‘amiloride-inhibitable sodium channel’ and the name itself gives a clue as to the type of diuretic involved. They are now referred to as epithelial sodium channels (ENaCs)30 and are located in the principal cells in the luminal membrane. They are aldosterone-sensitive and are inhibited directly by amiloride and triamterene, but indirectly by spironolactone and eplerenone. The four are known collectively as potassium-sparing diuretics. The principal cells not only have ENaCs, but also ROMK and water channels (AQP2). Another type of cells, the type A intercalated cells, are the primary sites of proton (Hþ) secretion into the lumen, tubular acidification being driven by a proton pump (luminal Hþ-ATPase). The ion channels (ENaCs and ROMKs) exclude anions, so the transport of Naþ or Kþ leads to a net movement of charge across the membrane. Naþ enters the cell down the favourable electrochemical gradient created by the basolaterally sited Naþ – Kþ-ATPase antiport. The driving force for Naþ entry is therefore considerably greater than the exit force for Kþ and the result is the generation of a lumen negative transepithelial voltage difference of some 10– 50 mV. It is this voltage that provides the motive force for Kþ to enter the lumen via the ROMK channels. It is also this voltage difference across the epithelium that ( partly) affects Hþ secretion into the lumen via the proton pump. Thus both Kþ and Hþ can enter the lumen. There is therefore a crucially important relationship between the amount of sodium arriving at the collecting duct and the consequent secretion of potassium destined to leave it in the urine. Any diuretic that acts upstream from the collecting duct (loop diuretic, thiazide or carbonic anhydrase inhibitor) will cause increased (loop most, thiazide moderate, carbonic anhydrase least) delivery of sodium to the collecting duct. If it arrives with an anion that cannot be as easily reabsorbed as chloride (e.g. HCO2 3 ), then the resulting lumen negative potential will be greater, and as a consequence more Kþ secretion will ensue. Add to this mechanism the increased aldosterone secretion from volume depletion activation of the renin – angiotensin – aldosterone system, and we have an explanation for virtually all the diuretic-induced potassium loss, leading to hypokalaemia and its associated metabolic alkalosis. Conversely, inhibition of sodium reabsorption in this nephron segment will inevitably result in hyperkalaemia and metabolic acidosis, the products of coexisting reductions in Kþ and Hþ excretion.29 424 Annals of Clinical Biochemistry Volume 49 September 2012 ................................................................................................................................................ Amiloride, a pyrazinoylguanidine derivative,23 and triamterene, a pteridine (Figure 4), act at site IV (Figure 1) to decrease the number of open ENaCs. Spironolactone, a 17-spirolactone (Figure 4), is a synthetic steroid that is an aldosterone competitive antagonist. Eplerenone is an analogue of spironolactone with improved aldosterone receptor selectivity. The steroid structure of spironolactone means that it has the potential to cause gynaecomastia and hirsutism among other ‘steroid’ effects. The aldosterone receptor is cytosolic and is a member of a superfamily of receptors for steroid hormones, retinoids, vitamin D and thyroid hormones. Epithelial collecting duct cells contain mineralocorticoid receptors with high aldosterone affinity. Very O O H3C H3C importantly, in diuretic terms, aldosterone gets to its receptor from the blood via the basolateral membrane and binds to hormone-sensitive elements (sections of DNA). The complex reaches the nucleus and modulates production of aldosterone-induced proteins (AIPS) and these are believed to activate ‘silent’ ENaCs.23 The effect of spironolactone on aldosterone classifies it as an aldosterone antagonist. The potassium-sparing diuretics are very weak natriuretics indeed, capable of a maximum excretion of 1– 2% of filtered sodium.29 Their main use is in combination with a loop or thiazide diuretic to minimize potassium loss, although the aldosterone antagonists have some special uses in other clinical settings. Although not generally regarded as diuretics, angiotensinconverting enzyme (ACE) inhibitors and angiotensin II receptor antagonists promote renal excretion of sodium and water by blocking the effects of angiotensin II in the kidney and by reducing aldosterone secretion. ENaCs are comprised of three subunits encoded by three different genes.30 The a subunit transports Naþ, while the b and g subunits enhance Naþ transport by the a subunit. Mutations in the b subunit (and less commonly the g subunit) cause them to ‘activate’ and become functional, leading to sodium retention and hypertension in an autosomal dominant disorder, Liddle’s syndrome.31 O Carbonic anhydrase inhibitors O S C CH3 Spironolactone NH2 N N NH2 N N NH2 Triamterene CI N CO NH C NH H2N N NH2 Amiloride Figure 4 Structures of some diuretics acting at the distal tubule NH2 The use of the bacteriostatic agents, sulphonamides, led to the realization that they caused an alkaline diuresis with hyperchloraemic metabolic acidaemia. Vast numbers of sulphonamides were synthesized and tested for their inhibition of carbonic anhydrase and now only one survives in clinical use – acetazolamide. The discovery of carbonic anhydrase in the kidney was made in 1941 by Davenport and Wilhelmi, and it is now known to be a zinc metalloenzyme found in large amounts in the luminal and basolateral membranes of the proximal tubule, either fixed to the membrane by a glycophosphatidylinositol anchor (similar to bone alkaline phosphatase) as the type IV enzyme or free in the cytoplasm as the type II enzyme.32 The reaction between water and CO2 is a slow process, but under the influence of carbonic anhydrase, it is speeded up several thousand-fold. By non-competitively inhibiting carbonic anhydrase (H2O þ CO2 H2CO3 Hþ þ HCO2 3 ) in the proximal tubule (site I in Figure 1), acetazolamide decreases the availability of Hþ for the Naþ 2Hþ exchanger so that not only the Naþ reabsorption is reduced, but there is impaired neutralization of luminal HCO2 and increased Cl2 3 reabsorption resulting in an alkaline diuresis and the development of a hyperchloraemic (normal anion gap) acidaemia.33 The diuresis produced is minimal, partly because tubular fluid leaving the proximal tubule gets reclaimed further down the nephron, and partly because the developing metabolic acidaemia self-curtails the diuretic action. Acetazolamide can be given orally or intravenous and its effect lasts about 12 h, since tolerance is induced by respiratory compensation for the metabolic acidaemia. Wile. Diuretics – a review 425 ................................................................................................................................................ Acetazolamide finds a use in the management of glaucoma by reducing intraocular pressure because aqueous humour is bicarbonate-rich (carbonic anhydrase in the ciliary processes) and acetazolamide inhibits its production. It can alkalinize urine, which aids removal of weak acids like uric acid and cystine, and most importantly can be used to treat oedematous patients with metabolic alkalosis, the urinary excretion of bicarbonate tending to restore acid– base balance. It has also been used to treat familial periodic paralysis and to enhance ventilation in case of altitude sickness. In familial periodic paralysis, there is a sudden drop in serum potassium due to its exchange with sodium in cells, an effect countered by the rise in Hþ in serum generated by acetazolamide, allowing sodium ions to exchange for Hþ rather than potassium ions. Side-effects are few: bone marrow suppression, allergic reactions (rashes) and interstitial nephritis are the main ones.34 secretion inhibited, then a diuresis results. Both ethanol and water are inhibitors of vasopressin secretion and so act as diuretics. Vasopressin acts on either a V1 or a V2 receptor, with action on the latter resulting in rapid activation of the Naþ 2Kþ 22Cl2 symporter in the thick ascending limb of the loop of Henlé, along with rapid insertion of prefabricated AQP2 water channels into the apical membrane of the principal cells of the collecting duct. Both theophylline and caffeine are methylxanthines, caffeine being 1,3,7-trimethylxanthine. They cause an increase in GFR by smooth muscle relaxation in the afferent arteriolar bed of the glomerulus, and probably a direct effect inhibiting salt reabsorption in the proximal tubule.40 Clinical uses of diuretics Which diuretic, for which patient and why Osmotic diuretics Urea, isosorbide, glycerin and mannitol all have been used in the past.35 Mannitol is the only one that remains in current clinical use and appears in the BNF as a 10% or 20% solution for intravenous use. Mannitol is a polyalcohol which is filtered by the glomerulus and not reabsorbed by the nephron. It therefore increases the tubular fluid osmolality, which decreases water reabsorption.36 All osmotic diuretics, including mannitol, exert their effect at nephron sites which have AQP water channels. Mannitol acts at the proximal tubule and thin descending limb of the loop of Henlé, both sites of AQP1 channels. Intravenous administration causes water to move from the intracellular to the extracellular compartment, which raises plasma and consequently tubular fluid osmolality.36 The lower gradient in osmolality between the tubular cells and lumen impairs water reabsorption, resulting in water diuresis and hypernatraemia.37 Unlike any of the other diuretics, mannitol produces a water diuresis rather than a natriuresis; water being lost over and above sodium and potassium. In patients with renal failure though, mannitol may be retained and substantially increase the plasma osmolality when water will leave cells, drawn by the osmotic gradient created and cause a dilutional hyponatraemia; the physician needs to be aware of this and treat the hyperosmolality and not the hyponatraemia.38 One of the most common uses of mannitol is its shortterm use to decrease brain bulk and cerebrospinal fluid production as a means of lowering intracranial pressure.39 Following crush injuries, or after cardiac and major vascular surgery, it can be used in an effort to minimize the acute decrease in glomerular filtration rate (GFR) that accompanies acute tubular necrosis predisposing to acute renal failure. Miscellaneous diuretics Both ethanol and water can be counted as diuretics, using the broad definition given earlier. The main function of the posterior pituitary hormone vasopressin (ADH) is water conservation, so if vasopressin is inhibited, or its Diuretics are used in conditions where there is oedema and those which are non-oedematous. A detailed description of the pathogenesis of oedema is not appropriate here, but an overview to be commended is that of Morrison.41 Oedema occurs in heart failure, renal failure, the nephrotic syndrome and with ascites in hepatic cirrhosis. The non-oedematous states include hypertension, nephrolithiasis, hypercalcaemia and diabetes insipidus. The choice of diuretic for each one depends on the combination of factors in the patient (a patient with heart failure may also have chronic kidney disease), the efficacy, the speed of onset of action, the metabolism and side-effects of the agent considered. One cannot rely on chemical grouping as a guide (as one can with oral hypoglycaemic agents), as reference to Figures 2 and 3 helps illustrate. There are three groups of sulphonamide-containing diuretics – with totally dissimilar renal pharmacologies. There is the carbonic anhydrase inhibitor, acetazolamide, the benzothiadiazines and the sulphamoyl benzoates. The prototype of these is furosemide (discovered in 1964), being chemically 4-chloro-N-furfuryl-5sulphamoyl anthranilic acid. Referring to Figure 3, one sees the 4-chloro-5-sulphamoyl substitution of the benzene ring in furosemide as reflecting a thiazide, but the presence of the monocyclic system with a furfuryl substitution at position 2 confers the ‘high ceiling’ activity of a loop diuretic, not a thiazide. The introduction of a phenoxy group at position 4 with a butylamino substitution at position 3 produced bumetanide, an agent that is on a weight basis 40 times more potent than furosemide, with absorption approaching 100% (80 – 100%). On average, the amount of furosemide absorbed is 50%, but this ranges from 10% to 100%, making it difficult to predict in an individual patient how much furosemide will be absorbed. About 50% of a dose of furosemide is excreted, unchanged, in the urine, the other 50% being conjugated to glucuronic acid in the kidney. Thus, in patients with renal insufficiency, the halflife of furosemide is lengthened by a decrease in urinary excretion and also in renal conjugation. Bumetanide and torasemide are mostly hepatically metabolized (50% and 80%, respectively), so in renal 426 Annals of Clinical Biochemistry Volume 49 September 2012 ................................................................................................................................................ insufficiency their half-lives are little altered. Conversely, in liver disease, the half-lives of both drugs will be prolonged and more drugs will be available to the tubular fluid, thereby increasing potency. The kaliuretic potency of bumetanide is 20 times greater than that of furosemide on a weight basis and so is claimed to be more ‘potassium-sparing’. There is also some evidence that it is likely to impair glucose tolerance and lead to retention of urate. It should now become clear that not all diuretics within a classification category are necessarily very similar and that the differences relate to their varied chemistry. Diuretics are commonly used to treat hypertension, but their effectiveness does not always relate to their diuretic effect. For example, thiazides work in hypertension42 by lowering peripheral vascular resistance over time,43 and because of this, they tend to be the preferred choice in this condition. Those patients with mild congestive heart failure, associated with mild oedema, are also suitable candidates for thiazides, but most will require a loop diuretic, although the rate of absorption of loop diuretics is slower in patients with severe heart failure. The maximum response will not occur for four hours or more after the dose.44 Use of diuretics is not devoid of risks, with many sideeffects being well recognized; however, some are less well known. Bendroflumethiazide is known to cause calcium retention, so care should be exercised when bendroflumethiazide is co-prescribed with either vitamin D or calcium supplements, and it is generally recommended that the dose of calcium or vitamin D supplement is reduced by 50%. Inhibition of distal sodium reabsorption may lead to some compensatory increase in proximal tubular reabsorption. This is of relevance in patients receiving lithium therapy since lithium shares the same reabsoptive pathway as sodium in this region of the nephron. Hence, distally acting diuretics (such as thiazides and spironolactone) stimulate proximal tubular reabsorption of lithium and the risk of toxicity.45 Furosemide does not seem to be associated with such enhanced reabsorption. Similarly, furosemide can be used in the treatment of hypercalcaemia as it increases urinary calcium excretion, whereas thiazides cause urinary calcium retention and can therefore cause hypercalcaemia. In the years since diuretics have been used to treat hypertension, it has been recognized that lower doses than originally used can be effective at providing good blood pressure control, with consequently fewer side-effects.46 For example, bendroflumethiazide is used at a dose of 2.5 mg/d, the previously used 5 mg dose affording no better blood pressure control and contributing to reduced glucose tolerance and hyperlipidaemia, especially hypertriglyceridaemia. It has long been acknowledged that thiazides could worsen diabetes in established diabetic patients, but their role in provoking glucose intolerance in previously glucose-tolerant patients remained controversial until, in 1982, Murphy et al. 47 published their findings in the Lancet, demonstrating that stopping the thiazide (after 14 years of treatment) improved glucose tolerance. Hypertension is conventionally thought of as being systemic hypertension, but diuretics, particularly the carbonic anhydrase inhibitor acetazolamide, are used to treat an uncommon form – that of idiopathic intracranial hypertension.48 This is often a chronic condition characterized by symptoms and signs of intracranial hypertension (where the cerebrospinal fluid is normal and no cause is identified by imaging), but where vision can be lost from papilloedema; depression and difficult to treat headaches are common. It is said to occur in around 20 per 100,000 obese women and the rational for using acetazolamide is thought to be the reduction of the formation of cerebrospinal fluid.49 A randomized placebo-controlled study to address the role of acetazolamide is currently being undertaken in the USA.48 Carbonic anhydrase is found not only in the proximal tubule but also the central nervous system, and common adverse effects are hyperchloraemic metabolic acidosis (with a normal anion gap) and nephrolithiasis, probably because the metabolic acidosis produces a low urine citrate and so the calcium is in a less soluble form. Acetazolamide also finds a use in periodic paralysis; the acetazolamide-generated rise in serum hydrogen ion concentration offers an alternative cation to potassium with which sodium can exchange.50 The BNF lists the diuretics currently available in the UK along with their licensed indications, contraindications and side-effects, as well as their recommended doses. These are shown in Table 1. Indipamide, xipamide and metolazone are structurally related to furosemide, although they are thiazide diuretics by class, and consequently their actions differ from those of the other thiazide diuretics. Indapamide, for example, in very low doses has little diuretic effect and is a weak diuretic, but reduces vascular tone and lowers blood pressure effectively.51 It has a marked influence on the serum concentrations of potassium, glucose, lipoproteins and, significantly, urate. Xipamide52 is chemically related to salicylic acid, and although it has a powerful diuretic action similar to that of furosemide, its onset of action (about 1 h) and duration of action (often in excess of 12 h) are more akin to hydrochlorthiazide, which is a medium-acting thiazide. Its long duration of action can prove troublesome for elderly recipients, and serum potassium concentrations as low as 2.2 mmol/L have been encountered with its long-term use. Regular serum potassium measurements are therefore needed. Metolazone belongs to the chemical class of quinazoline sulphonamides and can initiate diuresis within an hour of oral administration.53 In patients with renal impairment, it can generate an effective diuresis, and has been used in combination with furosemide when ‘furosemide resistance’ has been encountered. The BNF therefore includes a statement ‘also profound diuresis on concomitant administration with furosemide (monitor patient carefully)’.8 Torasemide differs from furosemide in that it is approximately twice as potent and has a somewhat longer duration of action, which facilitates once daily dose. This effectively does away with the paradoxical antidiuresis that is now and again encountered with furosemide. Additionally, its effect on potassium and calcium excretion is less marked.54 The BNF also includes an unusual (and unlicensed) application for acetazolamide – that of prophylaxis against mountain sickness (although it does stress that it is not a Wile. Diuretics – a review 427 ................................................................................................................................................ substitute for acclimatization).8 High altitude mountain sickness occurs over 3000 m, and is more likely if the ascent has been too rapid. The cause of the symptoms is hypoxia. The normal response to decreasing levels of oxygen tension is hyperventilation, but this is inhibited as alkalosis develops. The acetazolamide-induced metabolic acidosis increases respiratory drive, which helps to maintain the arterial oxygen saturation. This is particularly relevant at night; the time when (undesirable) attacks of apnoea have occurred. In particular, unpleasant (and hazardous) symptoms not only include lassitude and nausea, but also cerebral and pulmonary oedema.50 Fluid retention resulting in peripheral oedema and dyspnoea are cardinal features of the progressive stages of congestive cardiac failure, often referred to as chronic heart failure. Diuretics find their major role in the management of this condition. Peripheral (ankle) oedema is not always associated with chronic heart failure as it occurs in nephrotic syndrome (discussed later). The term heart failure embraces two separate but associated conditions, namely heart failure with preserved ejection fraction and heart failure due to left ventricular systolic dysfunction. It is crucial to distinguish heart failure with low ejection fraction from that with preserved ejection fraction because most high-quality evidence on treatment is for patients with low ejection fraction.55 Heart failure affects around 900,000 people in the UK, and is likely to become even more common as prognosis continues to improve for ischaemic heart disease (the major cause of heart failure) and an ever-increasing ageing population.55 Heart failure symptoms are classified according to the New York Heart Association Classification (NYHA). Fluid retention may be present in patients who have dyspnoea, an increase in weight of more than 2 kg from baseline in less than three days, raised jugular venous pressure, hepatomegaly, crepitations on chest auscultation or signs of peripheral oedema. In 2003, the National Institute for Health and Clinical Excellence (NICE) produced guidelines for the diagnosis and management of heart failure, and other studies have suggested that diuretics should be considered for patients with heart failure who have dyspnoea or ankle or pulmonary oedema. They should be given at the same time, or before ACE inhibitors, and both should precede the start of b-blockers.56 Although there is no obvious evidence that they positively influence mortality, diuretics are used on an individual basis to reduce fluid retention.56 Over-treatment can lead to dehydration as well as renal dysfunction, particularly with loop diuretics. Bumetanide, furosemide and torasemide (all loop diuretics) are all used and if pulmonary or ankle oedema persists, then the addition of a thiazide, metolazone or potassiumsparing diuretic-like spironolactone can be extremely useful. The different diuretic classes, acting as they do on different parts of the nephron, are thought to have an additive effect.56 It is important to monitor electrolytes, especially at the outset of treatment. Elderly patients can find some side-effects (e.g. frequent micturition) distressing and as long as their symptoms remain stable (and on ACE inhibitors and b-blockers), then consideration can be given to lowering the dose of loop diuretic, changing solely to a thiazide, or even stopping diuretics altogether. In patients with heart failure associated with a low ejection fraction classified as having NYHA class III or IV, an aldosterone antagonist like spironolactone can be life-saving.57 Hyperkalaemia should be looked for, especially when starting the drug, and this is more likely if the patient is also on an ACE inhibitor. Doses of spironolactone more than 25 mg/d should be used judiciously. The NICE guidance for heart failure has been updated since 2003, and a summary appeared in the British Medical Journal in August 2010.55 In it, as a new recommendation, an aldosterone antagonist (licensed for heart failure) is recommended if the patient has had a myocardial infarction within the past month. The new guidelines recommend the measurement of serum brain natriuretic peptides (BNP), suggesting that levels of BNP below 29 pmol/L or N-terminal proBNP below 47 pmol/L make a diagnosis of heart failure unlikely in an untreated patient. They point out, significantly, that treatment with diuretics including aldosterone antagonists may reduce serum natriuretic peptides, as indeed can obesity. In humans, atrial natriuretic peptide (ANP) acts as a diuretic and is cleared by neutral endopeptidase (NEP) in the kidney and elsewhere, as is BNP. Administration of ANP and BNP to patients with congestive cardiac failure produced a beneficial response including diuresis, improved haemodynamic parameters and suppression of the renin – angiotensin– aldosterone system.58 As a result, candoxatril, a neuroendopeptidase inhibitor, was developed but was associated with raised endothelin concentration, the most potent vasoconstrictor peptide (NEP is not specific for natriuretic peptides) and subsequently withdrawn. The concept of ‘nature’s own diuretic’ remains an area of research interest.59 Not surprisingly, in the 21st century, there are examples of molecular genetics starting to impact on the arena of diuretics. A recombinant form of BNP having the same 32 amino acid residue sequence as the endogenous peptide is now available. Called nesiritide, it is used intravenously in acute decompensated heart failure and has a mean terminal elimination half-life of 18 min. Interestingly, it can reduce serum endothelin concentration (which may be elevated in heart failure) from baseline.60 Other conditions in which diuretics are significantly involved in management are nephrotic syndrome, cirrhosis and, somewhat controversially, acute kidney injury. First in nephrotic syndrome, almost by definition, there exists a low serum albumin concentration, which encourages diffusion of the diuretic into the extracellular space, as they are usually bound to albumin. This means that less diuretic enters the renal tubule where it exerts its pharmacological effect (see earlier). To make matters worse, the high concentration of albumin in the tubule then binds free drug that has been secreted, rendering it inactive. Diuretic response, therefore in patients with nephrotic syndrome is suboptimal by about 50%.22 If the hypoalbuminaemia is very marked (below 20 g/L), then infusions of albumin along with furosemide can lead to an increase in sodium excretion, having partly overcome what is a form of ‘diuretic resistance’. 428 Annals of Clinical Biochemistry Volume 49 September 2012 ................................................................................................................................................ Many cirrhotic patients develop oedema, which is mediated through the secondary hyperaldosteronism that develops. The result is sodium and water retention, leading to oedema.56 To combat this, the only useful diuretic is the aldosterone antagonist, spironolactone, which is more effective than loop diuretics partly not only because of its antagonism to the raised aldosterone, but also because it is far less likely to engender hypokalaemia which may precipitate hepatic encephalopathy.61 Spironolactone is not as aggressive a diuretic as furosemide, which in cirrhosis patients is quite helpful since a massive diuresis can easily upset intravascular volume status. If spironolactone alone is not effective, then a thiazide can be added. However, for reasons that are not entirely clear, responses to loop diuretics in cirrhosis are often attenuated.62 Lastly, as described in the physiology section of this review, spironolactone possesses one other special, and useful, property. It does not have to enter the tubular fluid in order to exert its action, as it gains tubular cell entry from the plasma by crossing the basolateral membrane before competing with aldosterone for its cytosolic receptor. The Acute Kidney Injury Network (AKIN) is an interdisciplinary and international consensus panel, which has classified acute kidney injury (AKI) according to the RIFLE criteria (risk, injury, failure, loss and end stage kidney disease) based on changes in baseline serum creatinine or urine output. Logically, one might think of using diuretics to maintain or even increase urine flow. The concept of flushing away debris such as denuded epithelium with the avoidance of tubular obstruction and glomerular filtrate back leak is appealing. However, a systemic review of the literature undertaken by Karajala et al.63 concluded that diuretics do not work in AKI. The review expounds some putative explanations centering around reduction in GFR and loop diuretic-induced renin release, including a decrease in renal perfusion pressure. The upshot is that mannitol can cause more harm, and induce nephropathy, and even nesiritide did not improve renal function in patients with decompensated heart failure and mild chronic renal insufficiency. However, it is acknowledged that nesiritide may be effective in the prevention of AKI when applied in lower doses for a prolonged time in patients with mild to moderate renal insufficiency.63 In conclusion ‘diuretics have been shown to be ineffective in the prevention of AKI or for improving outcome once AKI occurs: at best, diuretics can help decrease symptoms of pulmonary oedema secondary to volume overload’.63 AKI that occurs perioperatively is associated with potentially serious consequences, and current advice on the use of diuretics is that in fluid-overloaded patients, loop diuretics may have a limited role. The decision to deploy them should be undertaken in consultation with a nephrologist because they will not prevent AKI and are, in high doses, associated with a risk of death and impaired renal function that does not recover, as well as possibly causing tinnitus.64 The nonrecovery may relate to more severe renal impairment with consequent resistance to the effects of the loop diuretics since they have to gain entry to the tubular fluid in order to reach their respective symports and deliver their pharmacological benefits. The situation is considerably different when considering chronic kidney disease (CKD). Patients with CKD will frequently need a diuretic as part of the management of their other co-morbidities, hypertension and heart failure. Although a proportion of patients with CKD derive clear benefit from a combination of ACE inhibitors and spironolactone, potentially fatal hyperkalaemia often develops. In advanced CKD, such a combination mandates use with extreme caution.65 Amiloride and triamterene also pose a hyperkalaemic risk and are to be avoided completely in advanced CKD.66 Acetazolamide requires a dose reduction in CKD stages 2 and 3, and should be avoided altogether in CKD stages 4 and 5 because of the high risk of acidosis and its poor efficacy in advanced CKD.67 Thiazides take longer to clear in CKD and are not very effective in advanced CKD (they need to penetrate the nephron lumen) and, as with loop diuretics which also display reduced clearance,68 doses need to be increased. Decreased fractional delivery of drug to the lumen results in resistance especially to loop diuretics.69 There is an additional risk of ototoxicity with loop diuretics in CKD due to the higher doses needed and the reduced clearance. The risk of ototoxicity is likely to be worse if the patient is also in receipt of an aminoglycoside group antibiotic.70 Biochemical disturbances due to diuretics The use of diuretics is not without its metabolic hazards as has been alluded to throughout the preceding sections. Table 2 lists the common metabolic complications of diuretic use. Hyponatraemia is common, made worse by patients who drink copious volumes of water as a result of being on the diuretic in the first place. Distinguishing hyponatraemia in patients without oedema from those with oedema is an important clinical distinction. In those with heart failure, nephrotic syndrome and cirrhosis, salt and water intake should be restricted as they are more likely to have expansion of their extracellular fluid volume. The mechanism was described earlier. Although the potassium-sparing diuretics can cause hyperkalaemia, this is more likely if used in conjunction with an ACE inhibitor or angiotensin II receptor antagonist. The same can apply to the concomitant use of potassium supplements and non-steroidal anti-inflammatory drugs (NSAIDs). These latter two are particularly important, Table 2 Metabolic complications of diuretics Hyponatraemia (especially with thiazides) Hypokalaemia Hyperkalaemia Volume depletion Metabolic alkalosis Hypomagnesaemia Hyperuricaemia Increased urea and creatinine Hyperkalaemia and metabolic acidosis with potassium-sparing diuretics Wile. Diuretics – a review 429 ................................................................................................................................................ since they can be purchased ‘over the counter’ (e.g. ‘Lo-Salt’ and ibuprofen) and unless physicians specifically enquire, then they may be unaware that the patient is taking them. Hypokalaemia occurs with both thiazide and loop diuretics and the physician needs to be aware that additional factors can, sometimes suddenly, worsen a mild hypokalaemia and cause the patient to develop symptoms which may include cardiac dysrhythmia. Such conditions include diarrhoea, vomiting and a small bowel fistula. Drugs include corticosteroids, amphotericin and theophylline in particular, which, if used together with diuretics, can exacerbate hypokalaemia.50 Both thiazide and loop diuretics can cause urinary magnesium loss, and if both are used together, such loss can be substantial.22 If diuretics are used too enthusiastically, a fall in intravascular volume occurs which produces orthostatic hypotension, which can be a particular problem in elderly patients taking thiazides. Thiazides can be associated with hypochloraemic metabolic alkalosis (as well as hyponatraemia, hypokalaemia and hypomagnesaemia). They worsen NSAID-induced nephrotoxicity and the hypokalaemia can precipitate digoxin toxicity. Being sulphonamiderelated drugs, allergic reactions come as no surprise and they potentiate non-depolarizing, neuromuscular-blocking agents (a fact of which anaesthetists need to be aware of).71 Thiazides have another property: they can cause hyperuricaemia and may lead to the clinical onset of gout. Around 50% of patients on long-term thiazides develop hyperuricaemia, but only 2% develop clinical gout.72 Two mechanisms probably operate. First, uric acid and diuretics are organic acids and likely compete with one another for the transport mechanisms that deliver them from the blood into the tubular fluid.50 Second, diuretic-induced depletion of the extracellular fluid volume can lead to reduced glomerular filtration and increased absorption of most solutes, including urate, in the proximal tubule.73 Loop diuretics also potentiate the effects of non-depolarizing neuromuscular blocking agents as well as aminoglycosides and cephalosporin antibiotics, in addition to the common electrolyte disturbances which they share with thiazides.71 Further information on adverse effects can readily be found in large clinical pharmacology textbooks.74 Patients with porphyria present a special circumstance and advice on ‘unsafe’ drugs including diuretics is available, and regularly updated, on a number of websites.75 Diuretic abuse Sadly, individuals can take it upon themselves to take diuretics inappropriately, in situations where no real clinical indication exists.55 Usually associated with an eating disorder (anorexia nervosa or bulimia), the hypokalaemia which can develop may prove fatal. Diuretic abuse is also encountered in sport and diuretics have been included on The World Anti-Doping Agency’s (WADA) list of prohibited substances.76 The use of diuretics is banned both in and out of competition and diuretics are routinely screened for by antidoping laboratories.76 Diuretics in diagnosis Diuretics are most often regarded as therapeutic agents, but furosemide has found a particular role in the diagnosis of distal renal tubular acidosis (RTA), also known as type I RTA.77 The furosemide-fludrocortisone test offers an alternative to the ammonium chloride urinary acidification test and is quicker to perform, as well as being a more palatable approach to test urinary acidification. One of the inherent problems with the ammonium chloride loading test is that it very often causes vomiting such that the test has to be abandoned. In the report by Walsh et al.,77 none of the subjects experienced adverse effects and the simultaneous administration of furosemide and the mineralocorticoid fludrocortisone was well tolerated. The authors reasoned that the furosemide increases distal tubule sodium delivery and the consequent enhancement of distal sodium absorption increases the lumen-negative transepithelial voltage, thereby indirectly stimulating proton secretion. The fludrocortisone given simultaneously enhances both principal cell sodium reabsorption and, because it increases the activity of the Hþ-ATPase, a-intercalated cell hydrogen ion secretion.78 The combination, they proposed, should provide sufficient and consistent stimulus to elucidate an acidification defect in type I RTA, without recourse to the use of ammonium chloride which causes a systemic acid load to be excreted.77 Future developments A new class of diuretics has recently been discovered and provides an exciting challenge for medical science. They are called AQP modulators, and are likely to be commercially exploited since a patent application for the first in its class, AqB013, has recently been submitted.79 Physiologists had long pondered the existence of ‘gates’ that could allow rapid reabsorption of water by renal tubular cells. Diffusion will only permit a mere trickle of water across what is effectively a hydrophobic barrier – the lipid bilayer of the cell membrane first proposed in 1935 as the Danielli –Davson model.80 With the characterization in the early 1990s of water channels called AQPs81 came the explanation of how water can pass through a membrane at around the rate of three billion water molecules per second per AQP channel.82 Several members of the AQP family also allow glycerol and urea permeability. AQP1 predominates in the proximal tubule and descending thin limb of the loop of Henlé, while AQP2 is present in the principal cells of the collecting duct, where, in response to vasopressin, it shuttles between intracellular vesicles and the apical membrane.83 Increased activity of AQP2 is a contributory factor in the pathophysiology of cirrhosis, heart failure and nephrotic syndrome (all conditions where diuretics form part of the treatment/ management strategy). Mutations in the AQP2 gene cause nephrogenic diabetes insipidus.83 Mouse knockout models have been developed to explore the possibilities of modulating AQP function and expression. Verkmann’s review concludes that mouse phenotype data suggest that modulators of AQP expression/