Dissertation (Deepender) - Lung Cancer
Document Details
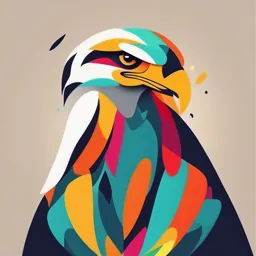
Uploaded by AmenableAzalea7493
Deepender
Tags
Summary
This dissertation investigates lung cancer, detailing its types (SCLC, NSCLC), subtypes (e.g., adenocarcinoma, squamous cell carcinoma), and significant risk factors. It explores the role of smoking, tobacco, cannabis, and air pollution in lung cancer development and progression.
Full Transcript
**INTRODUCTION** A class of disorders known as cancers is defined by the unchecked proliferation and dissemination of aberrant cells. Death may ensue if the metastasis (spreading of tumor cells) is not stopped. In addition to some internal factors (genetic mutations, random mutations, immunological...
**INTRODUCTION** A class of disorders known as cancers is defined by the unchecked proliferation and dissemination of aberrant cells. Death may ensue if the metastasis (spreading of tumor cells) is not stopped. In addition to some internal factors (genetic mutations, random mutations, immunological circumstances, and hormones), cancer is caused due to a variety of external variables (radiation, tobacco, infectious organisms, and chemicals. Several variables, like environmental contaminants, obesity, inactivity, certain infections, and dietary factors, are known to increase cancer risk. Together, these variables may start or encourage tumorigenesis in the human body, making cancer the primary reason for mortality **(Mathur *et al.,* 2015).** Oncogene mutations that result in altered cell growth and tumor development are the main causes of cancer **(Jemal *et al.,* 2010).** Further changes can potentially turn a benign tumor into an invasive carcinoma, characterized by metastasis, invasiveness, and anaplasia (loss of traits unique to a certain cell type) **(Durham *et al.,* 2015)**. Lung cancer (LC), which accounted for nearly one in eight (12.4%) cancer diagnoses and one in five (18.7%) cancer deaths globally in 2022, is the primary cause of cancer morbidity and mortality with nearly 2.5 million new cases and more than 1.8 million casualties worldwide. Male-to-female LC incidence and fatality rates are approximately 2 affecting males more frequently than women. Lung cancer is the most prevalent cancer among men to receive a diagnosis in 33 nations and the major reason for cancer-related deaths in 89 countries. Men\'s incidence rates are the highest in Eastern Asia, then Micronesia/Polynesia, Eastern Europe, and Turkey, which is thought to have the highest national rate among men globally. In 23 nations, including China and the US, LC is the most common cause of cancer-related deaths among women **(Bray *et al.,* 2022).** In India, the total number of new cases of lung cancer in men was 72,510, ranking fourth among all cancers while the total number of fatalities was 66,279, ranking fourth overall. Among deaths, the number of lung cancer cases among females was 26,490 **(Mathur** ***et al.,* 2020).** The estimation of new instances of lung cancer in the US (2018) was 112,350 cases (13% of new cancer cases in women) and 121,680 cases (14% of new cancer cases in men), for a total of 234,030 cases, or 641 cases per day **(Siegel *et al.,* 2018).** After prostate cancer in males and breast cancer in females, LC is the 2^nd^ most prevalent cancer diagnosed by gender **(de Groot *et al.,* 2018).** **TYPES OF LUNG CANCER** Two major types of LC are small cell lung cancer (SCLC) and non-small cell lung cancer (NSCLC) which are often derived from basal epithelial cells **(Zheng, 2016).** NSCLC accounts for 85% and SCLC accounts for 15% of all lung cancers **(Travis *et al.,* 2015).** Squamous cell carcinoma (SqCC), large cell carcinoma, and adenocarcinoma are the three main subtypes of NSCLC (Figure 1) **(Inamura *et al.,* 2017).** Cancers 14 00961 g001 550 **Figure 1.** Different types of lung cancer (**Hoang and Landi, 2022**) **SMALL CELL LUNG CANCER (SCLC)** A very aggressive and lethal kind of LC, SCLC is distinguished by its propensity for fast development, early metastasis, and acquired therapy resistance **(Rudin *et al.,* 2019).** Slightly over 10% of all lung malignancies are SCLCs. Metastatic illness is typically evident when a patient first presents. Within the first two years of starting therapy, the majority of patients relapse, and for metastatic patients, the 2-year survival rate is less than 10%. Typically, SCLC is found in the center of major airway. Compared to other forms of lung cancer, the tumor cells are often less than the diameter of three mature lymphocytes. The chromatin lacks noticeable nucleoli and is coarsely granular **(Lewis *et al.,* 2014).** There is not much cytoplasm, and the cellular boundaries are barely noticeable. There are more than 10 mitoses per 2 mm^2^, indicating a strong mitotic rate. In addition, there\'s often a high incidence of apoptosis and widespread tumor necrosis **(Zheng *et al.,* 2016).** Because of the long-term exposure to carcinogens found in cigarette smoke, SCLC has a very high mutation burden. It is thought that SCLC cells originate from (neuroendocrine cells) NECs or neuroendocrine progenitors (NEPs) in the lung because they have displayed neuroendocrine markers and a high mitotic index **(Karachaliou *et al.,* 2016).** **NON-SMALL CELL LUNG CANCER (NSCLC)** Squamous-cell carcinoma (SqCC), large-cell carcinoma (LCLC), and adenocarcinoma (ADC) are the three further subtypes of NSCLC. Of all instances of LC, 25-30% belong to squamous-cell carcinoma only. **Adenocarcinoma (ADC)** ADC is the main kind of LC, accounting for around 40% of all reported cases. Alveolar cells located in the epithelium of small airway act as the source of adenocarcinoma. It is more likely to be diagnosed earlier in the disease progression as it grows slower than the other forms of lung cancer and ADC is a malignant epithelial tumor having glandular differentiation. It exhibits mucin synthesis that can be identified using pneumocyte marker expressions like napsin A or thyroid transcription factor 1 (TTF-1), or by mucin staining such as mucicarmine **(Rodriguez-Canales *et al.,* 2016).** ADC with a diameter greater than 3 cm is classified as minimally invasive. Exclusion factors include the presence of lymphovascular invasion, perineural invasion, or tumor necrosis. The invasion size is greater than 5 mm, even in cases where the tumor and invasion sizes meet the criteria. Regardless of age, ADC is the main form of LC in both smokers and non-smokers. Peripheral lesions arise from the deeper inhalation of cigarette smoke, which might be because the lungs have filters in them to keep big particles out of the lungs **(Travis *et al.,* 2015).** **Squamous Cell Carcinoma (SqCC)** Between 25% and 30% of all malignancies related to the lung are squamous cell carcinomas (SqCC), which usually start from cells in the major bronchi and airway epithelium, and spread to the carina **(Travis *et al.,* 2013).** Epithelial cells of the bronchial tubes in the center of the lungs and early squamous cells of the airway are the source of this carcinoma type. Studies have observed a high correlation between SqCC and the smoking of cigarettes **(Zappa *et al.,* 2016).** Typically, immunohistochemical markers such as desmoglein-3, p40, CK5, and CK6 are present**.** SqCC often arises in the main or lobar bronchus and appears in a central position. The World Health Organization (WHO) defines SqCC as a malignant epithelial tumor with intercellular bridges and/or keratinization, or one that contains immunohistochemical markers indicative of squamous cell differentiation. Although keratinization is the distinguishing characteristic, many SqCCs may not exhibit these features **(Lemjabbar-Alaoui *et al.,* 2015)**. **Large Cell Carcinoma (LCLC)** LCLC constitute around 5-10% of all lung malignancies. The incidence is lowering as a result of improved immunophenotyping methods that enable more accurately classifying adenocarcinomas and poorly differentiated squamous cell carcinomas. These tumors are usually made of large size cells with a lot of cytoplasms and are poorly differentiated **(Duma *et*** ***al.,* 2019).** LCLC is undifferentiated NSCLC carcinoma and lacks any indication of small-cell, glandular, or squamous cell differentiation in histology or immunohistochemistry **(Rodriguez-Canales *et al.,* 2016).** The center region of the lung is where large cell carcinoma typically starts, however, it can also spread to neighboring lymph nodes, walls of chest, and even far located organs. Smoking has a high correlation with LCLC **(Zappa *et al.,* 2016).** **RISK FACTORS** Globally, lung cancer has been the primary cause of cancer-related deaths **(Liu *et al.,* 2014).** Many risk factors are there that contribute to increasing the chances of LC and out of all the risk factors, cigarette smoking has been found as its key cause **(Xue *et al.,* 2016). The factors that offer a considerable amount of risk to non-smokers are shown in Figure 2.**  **Figure 2. Risk factors of LC in non-smokers (https://www.sciencedirect.com/science/article/pii/S1040842820300330)** **Smoking** The single greatest hazard to public health that the world is now confronting is tobacco smoke. Worldwide, approximately 1.1 billion people smoke, and tobacco smoke alone causes the death of about 8 million people annually **(Carter *et al.,* 2015).** Of these fatalities, \~7 million are related directly to the use of tobacco, and more than 1.2 million are non-smokers which are related to indirect smoke exposure **(Zhou *et al.,* 2019).** **Tobacco** An important contributing cause of the increased rates of lung cancer incidence and mortality is the concurrent increase in the use of tobacco. The greatest significant risk factor for lung cancer development is thought to be tobacco use. In non-smokers with a diagnosis of lung cancer, risk factors other than tobacco use are significant contributors to lung cancer carcinogenesis. Up to 90% of lung cancer cases are related to tobacco use, making it the single biggest risk factor for the disease development. Tobacco is heated to 880°C or even more when smoked. Tobacco and tobacco smoke contain about 8,000 different compounds including nicotine which is a naturally occurring alkaloid that causes addiction **(Hecht *et al.,* 2014)**. It binds to nicotinic acetylcholine receptors (nAChR) in the nervous system and functions as an acetylcholine agonist releasing endorphins, dopamine, serotonin, norepinephrine, and gamma-aminobutyric acid (GABA) into the bloodstream. Although nicotine has not been observed to cause cancer directly, it upregulates nicotinic receptors, affects gene expression, and promotes tobacco dependency. It is also related to the progression of pre-existing lung tumors **(Corrales *et al.,* 2020).** **Cannabis Sativa** Tetrahydrocannabinol (THC), the primary psychoactive component of cannabis, is similar to nicotine in that it causes addiction but is not known to be carcinogenic. A quarter to a half of daily users are thought to be hooked, and up to seventeen percent of youth who start using marijuana will develop dependence. There is evidence that THC has a negative impact on teenage brain development, which is similar to smoking. Over the past 20 years, THC\'s ingredient proportion in cannabis products has been rising. There exists a correlation between youth tobacco usage initiation and marijuana use. Due to the burning of organic material, carcinogenic compounds are released at the time of marijuana smoking. Both the amounts of tar and polyaromatic hydrocarbons in marijuana smoke are significantly higher than in tobacco smoke. Due to the inhalation of marijuana smoke, distal airways get inflamed resulting in the production of cytokines **(Ramo *et al.,* 2012).** It has been proved that marijuana usage can cause pre-cancerous histologic and molecular changes to the bronchial epithelium, similar to those caused due to tobacco use **(de Groot *et al.,* 2018).** **Air Pollution** Particulate matter (PM), organic compounds (like PAHs), gases (like ozone, carbon monoxide, and sulfur oxides), anhydrous sugars, resin acids, microbes, metals (such as lead, vanadium, and nickel), hopanes, and other pollutants are the components of air pollution, also known as haze. These pollutants can originate from both natural and man-made sources **(Ho *et al.,* 2016).** PM2.5 (\< 2.5μm diameter) and PM10 (\< 10μm diameter) make up the majority of PM in the environment **(Zhou *et al.,* 2019).** Lung cancer is facilitated by the complex mixture of mutagenic and carcinogenic substances found in vehicle exhaust fumes. In metropolitan populations, nitrogen oxide and nitrogen dioxide released from automobile exhaust are the main causes of LC **(Kanwal *et al.,* 2017).** **Radon** In 10% of instances, residential radon from soil has been identified as the 2^nd^ most significant risk factor of LC development after cigarette exposure and the primary factor for increasing the LC risk in non-smokers **(Chen *et al.,* 2012).** The radioactive natural gas radon (222Rn) is created during the decay sequence of uranium (238U) from radium (226Ra). It can harm the respiratory epithelium\'s DNA, and radon exposure is thought to be the major cause **(Kim *et al.,* 2016).** **Occupational Exposures** With a correlation observed in 5-10% of cases, workplace exposures of various origins likely represent the third cause of lung cancer globally. There is strong scientific evidence linking arsenic, chromium, and ionizing radiation to other occupational drivers of lung cancer. The most frequent and well-known occupational cause of LC is asbestos exposure. There are two main kinds of naturally occurring fibrous minerals in this class: amphibole (which includes amosite, crocidolite, and tremolites) and serpentine (chrysotile). It is known to induce DNA damage, mutations, a persistent inflammatory response, and the generation of reactive oxygen species (ROS) and reactive nitrogen species, which can lead to carcinogenesis **(Akhtar and Bansal, 2017)**. **Estrogen** The female hormone estrogen has been linked to carcinogenesis caused by polymorphism in estrogen receptors (ER) (ER alpha and beta) and benzo \[alpha\] pyrene employing oxidative stress damage that is found in lung tissues **(Chen *et al.,* 2011).** Compared to normal epithelial cells, lung cancer cells were shown to have higher levels of ER alpha and ER beta expression and activity **(Akhtar & Bansal, 2017).** **Domestic Fuel Smoke** Lung cancer development has been linked to domestic fuel smoke as a risk factor. Worldwide, domestic fuel is used for heating and cooking **(Balmes *et al.,* 2019).** During combustion, smoke is released, increasing indoor air pollution and particulate matter exposure **(Corrales *et al.,* 2020).** Fine particulate matter, made up of airborne particles, is released into the environment due to burning fuel and chemical reactions in the atmosphere. Particulate matter varies in size, and various sizes can have distinct health impacts. Smaller particles can cause greater harm to the lungs since they can penetrate them **(Tomczak *et al.,* 2016).** **Infections** Chronic inflammation brought on by infections has been linked to the development of cancer. The intracellular pathogen-caused pulmonary TB is one type of persistent infection. Regardless of smoking status, Mycobacterium tuberculosis has been repeatedly demonstrated to increase the chances of LC, with a significant latency period **(Brenner *et al.,* 2011).** Lung cancer risk factors also include the human papillomavirus (HPV**) (Corrales *et al.,* 2020).** **Genetic Risk Factor** It includes the genes with strong penetrance and family history. Several registry-based studies reporting a significant familial risk for early-onset lung cancer have identified a positive family history of the disease as a risk factor. Even after a rigorous correction for smoking, higher relative risks were still discovered. High-risk pedigrees\' linkage study revealed a substantial susceptibility locus to chromosome 6q23-25. LC risk becomes high in the context of the Li-Fraumeni syndrome, which is defined by a germline mutation in the p53 gene. Genome-wide association (GWA) studies have been able to discover several genetic polymorphisms underpinning LC risk by using up to a million single-nucleotide polymorphisms (SNP) as tags to detect prevalent genetic variants. The three primary susceptibility loci found are located in the 5p15, 6p21, and 15q25 regions **(Malhotra *et al.,* 2016). Moreover,** lung cancer cytogenetic studies have shown recurring chromosomal changes. It has been linked to a wide range of chromosomal abnormalities, including loss of heterozygosity (LOH), isochromosomes, imbalanced translocation, and allelic loss. In individuals with NSCLC, extensive aneusomy (addition of two or more chromosomes per cell) is the main chromosomal alteration. Chromosome 5p gains are another common aberration found in NSCLC. Also, studies on karyotypes revealed polysomy, in the majority, of chromosome 7 **(Kang *et al.,* 2007).** In addition, lung cancer frequently exhibits extensive regions of chromosomal amplifications in chromosomes 1 and 3q or deletions in chromosomes 3 and 9p **(Kanwal *et al.,* 2017).** **EPIGENETIC REGULATORS** Heritable modifications in gene expression or cellular phenotype that do not affect the underlying DNA sequence are known as epigenetics **(Yao *et al.,* 2019**). Lung cancer develops and advances in large part due to epigenetic control. Lung cancer is among the tumor types for which epigenetic changes, such as disruptions in epigenetic patterns and mutations in epigenetic regulatory pathways, have been linked. Four phenomena are included in the field of epigenetics: RNA modification, non-coding RNAs, DNA methylation, and histone modification. It was originally believed that cancer was only a hereditary condition, but new research indicates that epigenetic changes are equally important in the development of cancer. Epigenetic control takes place at the levels of non-coding RNA, proteins, and DNA as well. The most researched epigenetic mechanism, DNA methylation (DNAm), is the major cause of gene silencing **(Jeltsch *et al.,* 2014).** DNA methyltransferase enzymes (DNMTs) covalently add a methyl (-CH~3~) group to the 5^th^ carbon of cytosine base (5mC), which defines DNAm. Mammals prefer to add methyl groups to the genomic areas enriched in contexts of CpG dinucleotides, known as CpG islands (CGIs). CGIs are often located inside or next to gene promoter regions **(Hoang *et al.,* 2022).** Numerous changes may be made to histone proteins, some of which encourage the transcription of genes. Post-translational histone modifications, like DNA methylation, alter the availability of DNA to the transcriptional machinery but do not change the nucleotide sequence of the DNA. Histone phosphorylation is one of the various functions of histone modifications, however, it is mostly known for its role in DNA repair following cell damage. While there are many other kinds of histone modifications, the most significant and well-researched for regulating transcriptional activity and chromatin structure are methylation, acetylation, ubiquitination, and phosphorylation **(Alaskhar Alhamwe *et al.,* 2018).** Furthermore, acknowledged as epigenetic regulators are the non-protein coding RNAs, consisting of long non-coding RNAs (lncRNAs) and micro RNAs (miRNAs) **(Ramazi *et al.,* 2023).** The genome also codes for a class of transcripts called non-coding RNAs (ncRNAs) which are primarily not translated into proteins. Despite not being translated, non-coding RNAs (ncRNAs) play crucial roles in many biological and physiological processes **(Tragante *et al.,* 2014).** Specifically, long non-coding RNAs (ncRNAs longer than 200 nt) are essential modulators of chromatin dynamics, gene expression, development, differentiation, and growth. As reported by the ENCODE project, the human genome is supposed to code more than 28,000 various lncRNAs, many of which are still being searched and have not yet been categorized **(Bhan *et al.,* 2017). M**icroRNAs are non-coding RNA molecules that are \~22 nt long and are highly conserved. As epigenetic regulators, miRNAs change the protein levels of the target mRNA without altering the basic sequence. In addition, epigenetic alterations like DNA methylation, histone modifications, and RNA modification also regulate the activities of miRNAs **(Yao *et al.,* 2019).** Some of the miRNAs are coded by exonic regions. However, most miRNAs are transcribed from the intronic regions of coding or non-coding transcripts. The multiple-step procedure of miRNA maturation initiates in the nucleus and terminates in the cytoplasm. RNA Pol. II transcribes them as massive mono- or polycistronic primary miRNA precursors **(Ha *et al.,* 2014).** Because of this, the 5′ ends of conventional pri-miRNAs have an m^7^G cap, while the 3′ untranslated region has a poly(A) tail **(Romano *et al.,* 2017).** So far, the whole spectrum of RNA types including ribosomal RNAs (rRNAs), transfer RNAs (tRNAs), messenger RNAs (mRNAs), and lncRNAs are modified by more than 170 distinct forms of RNA modifications **(Thapar *et al.,* 2018).** Such RNA tags may be added, removed, and decoded by specific machinery of enzymes, i.e., writers, erasers, and readers, in a manner similar to epigenetic alterations in DNA and histones **(Yue *et al.,* 2015).** The most common internal modification in mRNA is N6-methyladenosine (m^6^A), but mRNAs and non-coding RNAs (ncRNAs) also contain several other significant modifications, such as N1-methyladenosine (m^1^A), 7-methylguanosine (m^7^G), 5-methylcytosine (m^5^C), adenosine-to-inosine (A-to-I), and pseudouridine (Ψ/psi) **(Teng *et al.,* 2021).** **\ ** **MATERIAL AND METHODS** **A thorough search of the literature was done using a variety of books, Google Scholar, PubMed, National Library of Medicine, and other online available resources. Over a hundred research publications on lung cancer, risk factors, and the function of epigenetic regulators were selected. The purpose of this dissertation is** to summarize the existing understanding of how epigenetic mechanisms, specifically DNA methylation, histone modification, and non-coding RNAs contribute to lung cancer development and progression. **It also presents** an overview of studies assessing the potential of some epigenetic regulators as biomarkers for early lung cancer detection and the prognostic value of these regulators in predicting disease progression, response to treatment, and patient outcomes. **REVIEW OF LITERATURE** **DNA METHYLATION** DNA methylation silences the genes that the methyl (-CH~3~) group is linked to but does not alter the DNA sequence. It is a cellular process that is planned and can result in the methylation or demethylation of different genes under different temporal and spatial relevance. In typical circumstances, most of the cytosine-phosphate-guanine (CpG) sites in human body are methylated, while a small fraction of the unmethylated regions constitute CpG islands (CGIs) **(Dor *et al.,* 2018).** Mostly found in the gene\'s promoter region, CpG island is also partially found in its exon region, which is home to many housekeeping, tumor suppressor (TSGs), and DNA repair genes. Gene expression is mostly regulated by the methylation event at the promoter region specifically **(Liang *et al.,* 2021).** Prior research has demonstrated that two prevalent epigenetic characteristics of cancer cells are promoter hypermethylation-associated gene inactivation and generalized loss of DNA methylation termed as hypomethylation **(Heyn *et al.,* 2012).** Transcriptional factors and enzymes are prevented from accessing DNA due to upstream hypermethylation, which eventually suppresses the activity of downstream genes. Numerous DNA methylation changes have been linked to the neoplastic transformation in NSCLC **(Sandoval *et al.,* 2012).** Leading causes of oncogenesis include both hypermethylation and hypomethylation. The former is commonly found and occurs at the CGIs in the promoter region. Conversely, the latter is present genome-wide in different genetic sequences **(Vizoso *et al.,* 2015).** **The landscape of DNA methylation along with active and passive demethylation mechanisms, and methylation levels in normal and cancer tissue** are shown in Figure 3. \(A) 5mC formation is implemented by DNMT proteins, and can be sequentially oxidised by TET proteins to 5-hydroxymethylcytosine (5hmC), 5-formylcytosine (5fC) and 5-carboxymethylcytosine (5caC). 5caC can be excised by Thymine DNA glycosylase (TDG) and replaced with an unmodified cytosine through base excision repair (BER). BER-mediated DNA demethylation pathway is referred to as active demethylation. Alternatively, the oxidation of 5mC to 5hmC can compromise DNMT1-mediated maintenance during replication, resulting in passive demethylation of 5mC DNA methylation. (B) Distribution of 5hmC across genomic elements, showing enhancers, promoters and gene bodies. In normal tissue, levels of 5mC are shown in orange and 5hmC is shown in light blue. In cancer, the levels of 5hmC, shown in dark blue, are reduced. White circle, unmethylated CpG; black circle, methylated CpG. **Figure 3. The landscape of DNA methylation: (A) Methylation process along with active and passive demethylation mechanism, (B) Methylation levels in normal and cancer tissue (https://portlandpress.com/essaysbiochem/article-split/63/6/797/221497/The-DNA-methylation-landscape-in-cancer)** As a tumor develops from a benign proliferating mass to metastatic invasive cancer, there occurs a drop in DNAm throughout the genome. This correlation between the degree of hypomethylation of genomic DNA and the severity of the disease has been widely observed. Hypomethylation of DNA in LC was found to be specifically located at repetitive sequences, such as LINEs (long interspersed nuclear elements), SINEs (short interspersed nuclear elements), segmental duplications in sub-telomeric regions, heterochromatin repeats (such as satellite DNA), and LTR (long terminal repeat) elements according to high-resolution CpG methylation mapping **(Rauch *et al.,* 2008; Mehta *et al.,* 2015**). Numerous investigations have demonstrated that aberrant DNA methylation has a role in carcinogenesis, mostly via localized hypermethylation at certain genomic locations (primarily CpG islands), global hypomethylation, and direct mutagenesis at methylated cytosines **(Hansen *et al.,* 2011).** Furthermore, it is discovered that DNAm changes take place during lung adenocarcinoma development even before the establishment of atypical adenomatoid hyperplasia (AAH) **(Cai *et al.,* 2015).** According to many of the latest research, DNA methylation may be used as a biomarker to help identify lung malignancies at an early stage **(Liang *et al.,* 2019).** Recently, the detection of DNA methylation has become more and more common among clinicians. Patients with many conditions have displayed abnormal methylation **(Guo *et al.,* 2017).** Patients with early-stage LC can be successfully screened by looking at certain gene methylation patterns. Among all, the genes RASSF1A and SHOX2 are frequently used as DNA methylation indicators in lung cancer **(Liang *et al.,* 2021).** Homeobox (HOX) genes form the group of transcription factors that share a similar homeodomain and are arranged into 4 clusters (HOXA-D) on distinct chromosomes **(Booth *et al.,* 2007).** The 3′ regions of HOXA and B are primarily expressed in normal lung tissues in adults, while HOXC and D are usually expressed in disorders like primary pulmonary hypertension and NSCLC, and fetal lungs **(Pradhan *et al.,* 2013).** The HOXA cluster is often methylated in NSCLC, and methylation of HOXA5 and HOXA11 promoters reduces their ability to control tumor growth by transcriptionally silencing them. However, inhibiting HOX activity decreases the development of tumors in vivo, demonstrating the ability of HOX genes to promote tumor growth. Thus, in NSCLC, dysregulated HOX genes may perform in both tumor suppression as well as oncogenesis **(Ooki *et al.,* 2017).** CDKN2A hypermethylation has been seen in pre-cancerous lesions, suggesting that it can transpire in the early development of lung malignancies **(Lin *et al.,* 2009).** Stage I NSCLC has been linked to methylation of promoter regions in APC, RASSF1A, ABCB1, ESR1, HOXC9, and MT1G, indicating that these methylations may also happen quite early in cancer development. In almost all early-stage tumors, CGI methylation of HOX-related genes is prevalent in LC stage I **(Rauch *et al.,* 2007).** On the other hand, advanced-stage NSCLC has been linked to frequently hypermethylated genes like H-Cadherin, hDAB2IP, FBN2, and DAL-1, indicating that these alterations could take place later in the carcinogenic process **(Langevin *et al.,* 2015).** DNAm alterations at cg03636183 in F2RL3 gene and at cg05575921 in AHRR gene were linked to an increased LC risk. These changes were also linked to mediation analyses, which showed preliminary evidence that hypomethylation at these two sites may mediate the effect of tobacco on LC risk and that residual confounding was unlikely to explain the observed associations for cg03636183 and cg05575921 **(Baglietto *et al.,* 2017).** When compared to mutational detection, DNA methylation may have some advantages. Firstly, oncogenic mutations such as EGFR and KRAS are usually biallelic or homozygous (with one allelic copy lost) whereas methylation events, which usually act as tumor suppressors with the lack of expression, are usually heterozygous (no loss of wild-type allele**) (Ward *et al.,* 2008).** Second, as a result of cellular turnover, increasing apoptosis (the primary source of ctDNA) increases the number of circulating nucleosomes. The first evidence that SqCC was preceded by gene promoter hypermethylation came from a study that used a 2-stage nested PCR to identify CDKN2A and MGMT promoter methylation **(Farooq *et al.,* 2020).** A significant number of differently expressed genes in lung cancer correlate with mRNA expression promoter and methylation levels, according to the meta-analysis of mRNA expression and DNA methylation **(Jiang *et al.,* 2017)**. When compared to non-smoking NSCLC patients, seven NSCLC-associated hypermethylation (MGMT, CDKN2A, DAPK, RASSF1, FHIT, RARB, and WIF1) demonstrated statistically significant evidence in smoking NSCLC patients **(Gates *et al.,* 2014).** According to the subgroup meta-analyses by ethnic groups, NSCLC patients shared risk factor for cigarette smoking was discovered to be CDKN2A hypermethylation. It has been demonstrated that cigarette smoking causes DNMT1 accumulation, which results in hypermethylation of the TSGs and may promote carcinogenesis and poor prognosis in NSCLC patients **(Huang *et al.,* 2015).** The hypermethylated genes and promoters with substantial oncogenic effects (S100A7L2, SOD1P3, DCAF4L2, and SNORD114-14) and tumor suppressor effects (LINCMD1 and CELF2-AS1) are found in Programmed death ligand 1 (PD-L1) high-expressing tumors **(Jiang *et al.,* 2020).** Different patterns of methylation in lung ADC with negative and high expression of PD-L1 have been found in an analysis by Hutarew et al. Upon examining the relationship between the pathobiology of genes and promoters and their methylation findings, it was observed that tumors exhibiting high PD-L1 expression are more likely to display oncogenic effects due to hypermethylation. Conversely, tumors expressing negative amounts of PD-L1 exhibit hypomethylation, which results in the loss of suppressor activities. The concurrent activation of powerful oncogenic pathways renders the suppressing activities of hypermethylated promoters and genes ineffective. In both cases, the tumor microenvironment promotes tumor development **(Hutarew *et al.,* 2024).** Although DNAm in the promoter region is thought to limit gene expression, more recent studies have revealed that promoter hypermethylation does not always result in this outcome **(Suzuki *et al.,* 2012).** Studies have shown, for example, that gene transcription and translation can be activated through the hypermethylation of specific promoters **(Nabilsi *et al.,* 2009).** Apart from the transcriptional activation linked to promoter hypermethylation, a non-conventional viewpoint has surfaced, indicating that hypermethylation in gene body areas may also facilitate gene expression **(Gan *et al.,* 2024).** Some of the DNA methylation-related genes have been identified as possible biomarkers with clinical significance for diagnosis, prognosis, and treatment response **as given in Table 1.** **Table 1. DNA methylation-related genes of clinical significance in lung cancer** +-----------+-----------+-----------+-----------+-----------+-----------+ | **Gene** | **Type of | **Functio | **Cell | **Cell | **Referen | | | biopsy** | n** | line/ | type** | ces** | | | | | Tumor | | | | | **(solid/ | | tissue** | | | | | liquid)** | | | | | +===========+===========+===========+===========+===========+===========+ | *P16* | Solid | Cell-cycl | Tumor | NSCLC | **Li *et | | | | e | tissue | | al.,* | | | | control | | | 2020** | +-----------+-----------+-----------+-----------+-----------+-----------+ | *RASSF1A* | Liquid | Ras | Cell line | NSCLC, | **Hu *et | | | | signaling | | SCLC | al.,* | | | | | | | 2019** | +-----------+-----------+-----------+-----------+-----------+-----------+ | *APC* | Liquid | Regulatio | Cell line | NSCLC, | **Feng | | | | n | | SCLC | *et al.,* | | | | of cell | | | 2016** | | | | prolifera | | | | | | | tion, | | | | | | | migration | | | | | | | , | | | | | | | and | | | | | | | adhesion | | | | +-----------+-----------+-----------+-----------+-----------+-----------+ | *RB* | Liquid | Cell-cycl | Cell line | NSCLC, | **Papavas | | | | e | | SCLC | siliou | | | | control | | | *et al.,* | | | | | | | 2024** | +-----------+-----------+-----------+-----------+-----------+-----------+ **HISTONE MODIFICATION IN LUNG CANCER** Gene expression is influenced by epigenetic alterations in the structure of chromatin. Specifically, transcriptional activity is higher in euchromatin, a loosely packed form of DNA, and lower in heterochromatin, a densely packed form of DNA **(Jiang *et al.,* 2009).** The structure of nucleosomes can be changed by chromatin remodeling complexes, changing the accessibility of DNA **(Shanmugam *et al.,* 2018).** Histone tail arginine and lysine residues are methylated via histone methylation **(Bedford *et al.,* 2009).** The places on H3 tails where arginine residues are most heavily mono- or dimethylated at their guanidinium groups active transcription is linked to methylation at these sites. PADI4, or peptidyl arginine deiminase 4, demethylates arginine residues by converting arginine to citrulline, which prevents methylation **(D\'Oto *et al.,* 2016).** Chromatin-modifying enzymes are a broad class of non-histone chromatin-associated proteins that change histone tails. Multicomponent protein complexes including these enzymes are found in cells, where they are usually attracted to chromatin in conjunction with transcription factors bound to DNA **(Audia *et al.,* 2016).** By binding certain co-activators and transcription factors and changing the structural characteristics of chromatin, a large number of covalent post-translational modifications (PTMs) in histones and related areas of DNA perform crucial functions in genomic processes. The four types of chromatin modification enzymes are methylation by histone methyltransferases (HMTs), demethylation by histone demethylases (HDMs), acetylation by histone acetyltransferases (HATs), and deacetylation by histone deacetylases (HDACs) **(Bowman *et al.,* 2014).** **The scheme of the histone methylation/demethylation and histone acetylation/deacetylation is shown in Figure 4.** These groups are categorized based on their respective roles. Together with the tumor suppressor genes (TSGs) linked to lung cancer, the produced PTMs may work together or independently to support inhibition or activation of chromatin-mediated gene expression of inflammatory cytokines, apoptosis, cell cycle arrest, senescence, antioxidants, and growth factors **(Bajbouj *et al.,* 2021).** Chromatin condensation is lessened by histone acetylation. The balance between histone acetylation and histone deacetylation in proper cell activity is regulated by the enzymes HATs and HDACs. HATs catalyze the movement of the -vely charged acetyl group from acetyl-CoA to the amino-terminal tail of lysine, thereby neutralizing the +ve charge on histones. It creates space between nucleosomes and DNA histones, which gives transcription factors a chance to access, bind, and promote DNA transcription **(Min *et al.,* 2012).** Conversely, histone deacetylation goes back to its initial condensation: HDACs clear the acetyl group, restoring the positive charge, and allowing negatively charged DNA to bind with histone proteins **(Giaginis *et al.,* 2014).** This condensation of chromatin structure is linked to the repression of genes **(Chrun *et al.,* 2017).**  **Figure 4. Scheme of histone acetylation/deacetylation and histone methylation/demethylation (https://www.sciencedirect.com/science/article/pii/S1044579X20301760)** Writers and erasers are the enzymes that add and delete histone PTMs from histones, respectively. Examples of "writers" that add acetyl, methyl, and phosphoryl groups to histones are HATs, HMTs, and histone kinases respectively **(Baek *et al.,* 2011).** Examples of "erasers" that remove acetyl, methyl, and phosphoryl groups on histones are HDACs, HDMs, and histone phosphatases respectively. It is also known that chromatin-related proteins and histone-modifying enzymes interact, affecting important cellular functions including transcription, replication, and repair **(Khan *et al.,* 2015).** Histone H4 modification patterns in lung cancer cells were found abnormal, showing loss of H4K20 trimethylation, hypoacetylation of H4K12/H4K16, and hyperacetylation of H4K5/H4K8. Their results underscore the significance of histone H4 alterations and point to H4K20me3 as a promising biomarker for LC therapy and early diagnosis. Higher expression of HDAC1 and HDAC3 genes appears to be correlated with a poor prognosis in pulmonary ADC patients, suggesting that HDAC1 gene expression may be linked to the advancement of LC. Target protein identification using antibody microarrays revealed that, in 92% of tumors with SqCC histology, HDAC3 levels were likewise raised **(Ansari *et al.,* 2016). *I****n vitro* analyses have shown cigarette smoke condensate (CSC) to upregulate mesenchymal markers in LC cell lines and to encourage the depletion of epithelial markers like E-cadherin. Slug and T-cell factor-1 (TCF-1) are expressed more when CSC loses its E-cadherin expression, which is mediated by HDAC-1 **(Nagathihalli *et al.,* 2012).** On the other hand, via acetylation of H3 and H4 histones, the HDAC inhibitor Entinostat increases the E-cadherin expression and suppresses cell motility and metastasis. By deacetylating the non-histone protein STAT3, high production of HDAC-7 renders it inactive. In lung cancer, this stimulates cell division and prevents apoptosis. In NSCLC cells, a pro-metastatic phenotype is induced both *in vivo* and *in vitro* by acetylating K185 and K201 lysine residues of the C1 member of the aldo-keto reductase 1 family (AKR1C1) (**Contreras-Sanzón *et al.,* 2022).** The findings of the meta-analysis revealed a strong relationship between the differentiation grade of lung cancer and HDAC1 expression. Furthermore, a meta-analysis was conducted to ascertain the distinction in HDAC1 expression between ADC and SqCC **(Yu *et al.,* 2015).** The findings indicated that squamous cell carcinoma had a greater level of HDAC1 expression than adenocarcinoma. Lastly, it was noted that compared to the patients with high HDAC1 expression, individuals with reduced HDAC1 expression had a superior overall survival rate **(Cao *et al.,* 2017).** In NSCLC cell lines, vorinostat and trichostatin A (TSA)-induced HDAC inhibition showed potent anti-tumor action **(Miyanaga *et al.,* 2008).** TSA treatment caused dose-dependent apoptosis in H157 cells of LC by activating the intrinsic mitochondrial and extrinsic/Fas/FasL system death pathways **(Li *et al.,* 2014).** In NSCLC cells NCI-H460 and NCI-H520, vorinostat caused overexpression of the cyclin-dependent kinase inhibitor p21, cell cycle arrest at G0-G1, and a reduction in the levels of bcl-2 **and** C-myc **(Mamdani *et al.,* 2020).** In a study of SCLC data collection, mutations were also discovered in other chromatin-modifying enzymes, such as PBRM1 (a tumor suppressor candidate), which codes for BAF-180 and is often mutated in renal cell carcinoma and cholangiocarcinoma. PBRM1 is found to be located at 3p21, a region that is frequently deleted in human SCLC **(Varela *et al.,* 2011).** It is a specificity-determining element of the PBAF SWI/SNF complex. To change the nucleosome placement, Adenosine triphosphate (ATP) is used by PBRM1 **(Jiao *et al.,* 2013).** Compared to tumors, truncating KMT2D mutations were more common in SCLC cell lines. Mutations in phosphatase and tensin homolog gene (PTEN) and phosphatidylinositol-4,5-bisphosphate 3-kinase catalytic subunit alpha gene (PIK3CA) are also more common in SCLC cell lines than in actual tumors **(Augert *et al.,* 2017).** **Histone Methylation** Histone methylation is the most well-researched type of histone modifications, may either stimulate or suppress transcription at various gene loci, and as a result, it has a complicated role in lung cancer. This essential epigenetic event is involved in many biological processes, including RNA splicing, transcription regulation, and carcinogenesis **(Cheng *et al.,* 2014).** The methylation of histone tail arginine (R) and lysine (K) residues is thought to have a major role in chromatin structure and, therefore, biological effects. Histone methylation is also a dynamic process controlled by a number of \"writer\" and \"eraser\" enzymes **(Audia *et al.,* 2016).** Certain methyl markers that are essential for gene expression, cell destiny, and genomic integrity are added or removed by methylation \"writers,\" and \"erasers\" respectively **(Kimura *et al.,* 2013).** Histone lysine methyltransferases/demethylases are the enzymes that act as methyltransferase \"writers\" and corresponding demethylase \"erasers\" for histone lysine residue **(Chen *et al.,* 2018**). Histone methyltransferases (HMTs) and histone demethylases (HDMs) dynamically catalyze histone methylation at the lysine and arginine sites in the H3 and H4 N-terminal tail. The lysine residues can be mono-, di-, or trimethylated whereas the arginine residues might be mono-, di-, or asymmetrically demethylated. Three functional enzyme families include HMTs: arginine methyltransferase PRMT family, non-SET domain DOT1L lysine methyltransferase PRDM family, and SET domain-containing lysine methyltransferases. HDMs are responsible for regulating the methylation level of histones. They belong to two major families: histone demethylases and lysine-specific demethylases (LSD) which include the JMJC structural domain **(Ye *et al.,* 2022).** Catalyzed by EZH2, H3K27me3 is associated with transcriptional repression, necessary for cancer chemoresistance, and acts as a lung cancer prognostic factor. Numerous investigations have demonstrated that HOX antisense intergenic RNA (HOTAIR) and EZH2 pair mediate cell invasion and metastasis. By controlling the lncRNA HOTAIR, H3K27me3 influences the methylation of HOXA1 DNA, resulting in multidrug resistance in SCLC. Furthermore, H3K27me3 uses a negative feedback mechanism to modify HOTAIR expression. These results contribute to knowledge of the mechanism behind SCLC chemoresistance and suggest that H3K27me3 can serve as a useful target for therapeutic intervention **(Fang *et al.,* 2018).** **Histone Acetylation** Lysine acetylation is one of the most common PTMs on histones. It is essential for controlling the accessibility of the underlying DNA and chromatin dynamics in eukaryotes **(Kouzarides *et al.,* 2007).** Histone acetyltransferases (HATs) and histone deacetylases (HDACs) are two families of counteracting enzymes that regulate acetylation on histone lysine residues. Acetylation is often linked to the active transcription. The large acetyl groups can act as docking sites for reader proteins by neutralizing the +ve charge on the lysine residues' side chain. The reader proteins identify this particular alteration and transduce the chemical signals to produce a range of downstream biological effects **(Ali *et al.,* 2012; Mi *et al.,* 2017).** HDACs catalyze the removal of acetyl groups from histone lysine residues to control the remodeling of chromatin. This heterochromatin state happens when the chromatin is densely packed and inhibits the gene transcription in this situation **(Li *et al.,* 2020).** The four classes of human HDAC enzymes consist of the following 18 enzymes: HDAC 1-3, and 8 are the members of Class I HDAC; Class II is sub-divided into two sub-groups, IIa (HDAC 4, 5, 7, and 9) and IIb (HDAC 6 and 10); Class III is referred to as Sirtuins (SIRTs) and it consists of SIRT 1-7 and Class IV which only contains HDAC-11. All of the class I members are typically found in the nucleus excluding HDAC 3 which moves to the cytoplasm **(Seto *et al.,* 2014).** Shuttles between the cytoplasm and nucleus are possible for class IIa and IIb HDACs. Class III SIRT 1 and 2 are found in both the cytoplasm and the nucleus, SIRT 3-5 are located in the mitochondria while SIRT 6 and 7 are found in the nucleus. Class IV HDAC is limited to the nucleus of cells **(Contreras-Sanzón *et al.,* 2022).** HDAC1 is an essential epigenetic factor that opposes the acetylation state of both histone and non-histone proteins. It has a well-established correlation with the onset and spread of cancer. For instance, HDAC1 overexpression increased cell proliferation, suggesting that HDAC1 promotes the development of cancer cells, whereas silencing of HDAC1 using siRNA led to the arrest of cell cycle and cell growth suppression **(Aghdassi *et al.,* 2012).** Furthermore, it was shown that HDAC1 was attracted to the CDH1 promoter in order to suppress the production of the cell adhesion protein E-cadherin, which in turn prevented the migration of cancer cells in pancreatic cancer. A correlation was found between the differentiation grade of lung carcinoma and HDAC1 expression. Furthermore, squamous cell carcinoma had greater levels of HDAC1 expression than adenocarcinoma. Also, a negative correlation was observed between the overall survival rate **of** LC patients and HDAC1 expression **(Cao *et al.,* 2017).** Histone acetylation of H3 and H4 at several lysine residues is catalyzed by a single HAT family member called HBO1, often referred to as KAT7 or MYST2 **(Iizuka *et al.,* 2009).** Both histone acetylation and acetyl-CoA binding need HBO1. Multiple important physiological behaviors and activities are regulated by HBO1 through its interaction with native subunits and cofactors. It is crucial for the development of the immune system, cell self-renewal, and transcription and expression of genes. The BRPF scaffold proteins and HBO1 interaction are essential for histone H3K14 acetylation (H3K14ac) **(Lan *et al.,* 2020).** In NSCLC, dysregulation of HATs is frequently seen. NSCLC has an overexpression of one HAT hMOF, which is critical for the carcinogenesis and development of NSCLC. HAT inhibitor CPTH6 specifically reduced the proliferation and viability of LC stem cells **(Chen *et al.,* 2014).** In NSCLC cells, another HAT inhibitor A485 was found to increase TRAIL sensitivity. NSCLC cells were made radiosensitive by the p300 HAT inhibitor C646 through the facilitation of mitotic catastrophe **(Chen *et al.,* 2022).** DNA-targeting anti-cancer medications can more easily access the chromatin structure when HDAC inhibitors (HDACIs) enhance the acetylation of key histone proteins (**Eckschlager *et al.,* 2017).** Nevertheless, in order to mediate their anti-cancer impact, HDACIs also have pleiotropic cellular actions and increase the expression of pro-apoptotic and cell cycle-regulating genes **(Suraweera *et al.,* 2018).** **Histone Phosphorylation** Histone phosphorylation at the 10^th^ and 28^th^ serine residues on the H3 histone subunit (H3S10ph and H3S28ph, respectively) has been linked to stimulus-dependent gene expression. The inability to modify histone phosphorylation at various loci within native chromatin settings has made it difficult to define the causative role of endogenous H3S28ph and H3S10ph **(Li *et al.,* 2021).** Serine phosphorylation at position 139 (S139) in H2AX has been extensively researched and is a crucial step in the identification and handling of DNA damage **(Gagou *et al.,* 2010).** It has been observed that the very first stage of DNA damage response (DDR) is the phosphorylation of histone H2AX at S139, which results in the formation of γ-H2AX **(Ji *et al.,* 2017).** This signal is crucial for the recruitment and retention of DDR complexes at the damage site **(Moeglin *et al.,* 2021).** Prototypic RTKs, including the EGF receptor (EGFR), initiate the MAPK signaling cascade. RAS proteins, including KRAS, NRAS, and HRAS, are transphosphorylated and activated by RTKs. These trigger the activation of different RAF proteins (ARAF, BRAF, CRAF). Similarly, RAF proteins trigger MEK1/2, which in turn triggers ERK1/2 **(Dhillon *et al.,* 2007).** Eventually, a number of genes controlling apoptosis, differentiation, and proliferation are activated by ERK1/2. Repeated somatic mutations of dominant oncogenes like EGFR, HER2, KRAS, or BRAF in lung cancer can result in long-term MAPK/ERK pathway activation (Reissig *et al.,* 2020). **NON-CODING RNA** Housekeeping ncRNAs, such as rRNAs, tRNAs, telomerase RNA, and RNase P RNAs are constitutively expressed and necessary for normal cellular function and homeostasis. On the other hand, regulatory ncRNAs exhibit particular temporal and spatial expression patterns **(Prasanth *et al.,* 2007).** Regulatory non-coding RNAs are further categorized based on their length: small non-coding RNAs (\200 nt long, mostly polyadenylated, and are involved in subcellular transport, splicing, imprinting, and epigenetic regulation **(Ricciuti *et al.,* 2016).** **MicroRNAs in Lung Cancer** A family of tiny non-coding RNAs of 21-25 nucleotides, known as microRNAs (miRNAs), can block the translation of messenger RNA (mRNA) and promote its destruction by base pairing with complementary sites on target mRNAs. MiRNAs modify post-transcriptional changes in gene expression via this mechanism. In *Caenorhabditis elegans*, the first ncRNA, lin-4, was discovered to be miRNA in 1993 **(Wu *et al.,* 2019).** MicroRNAs are known to play roles in the EMT (epithelial-mesenchymal transition) and lung inflammation mechanisms, which in turn affect the development of LC and how well a treatment works **(Wang *et al.,* 2014).** Interest in this field of study has grown as a result of the potential uses of microRNAs as therapeutic targets and in the diagnosis as well as prognosis of cancer. The microRNAs that are now being researched the most include miR-196, let-7, miR-21, miR-125b, miR-155, miR-135b, and miR-210 **(Castro *et al.,* 2017).** MiR-143-5p is one of the miRNAs that directly targets the expression of glycolytic enzymes. It has been demonstrated that the hyperactivation of mTOR signaling in lung cancer downregulates the expression of the glycolytic enzyme Hexokinase 2 (HK2) **(Fang *et al.,* 2012).** In contrast, the re-expression of miR-143-5p suppressed the expression of HK2 and impacted the glucose metabolism of LC cells **(Iqbal *et al.,* 2019).** By using exosomal miRNAs to transmit genetic information across cells in the tumor environment, exosomes encourage cell growth in LC. On the other hand, exosomal miR-512 inhibited cell growth by focusing on TEA domain family member 4 (TEAD4), suggesting it plays a tumor-suppressing part in LC **(Aylon *et al.,* 2016).** Furthermore, it was shown that exosomal miR-208a derived from NSCLC cells A549 functioned as a transfer messenger, specifically targeting p21 and activating mTOR pathway in the process, hence impeding the proliferation of NSCLC cells **(Hu *et al.,* 2020).** In lung cancer, there is a negative correlation between poor post-operative survival and decreased expression of the miRNA let-7. This was the first study to draw attention to the connection between changed miRNA expression and lung cancer biology and clinical outcomes **(Takamizawa *et al.,* 2004).** Additional research has characterized the tumor suppressor functions of the let-7 family in lung cancer, where it has been demonstrated that over-expression of the let-7 family in the A549 lung adenocarcinoma cell line reduces cell-cycle progression and inhibits cell growth **(MacDonagh *et al.,* 2015).** **Long non-coding RNAs (lncRNA) in lung cancer** Numerous lncRNAs have been linked to the advancement of cancer. These include HOTAIR, metastasis-associated lung adenocarcinoma transcript 1 (MALAT1), and antisense non-coding RNA in the INK4 locus (ANRIL) **(Tano *et al.,* 2012).** LncRNA nuclear-enriched abundant transcript 2 (NEAT2), another name for MALAT1, is highly conserved in mammals **(Shen *et al.,* 2015).** Significant contributions to the lncRNA-mediated regulation of gene expression are made by chromatin-modifying complexes. According to recent research, one class of lncRNAs, which makes up 24% of all lncRNAs, is expressed in different cell types and bound by polycomb repressive complex 2 (PRC2) made up of SUZ12, EED, and EZH2. PRC2 also serves as a molecular scaffold for complexes that modify histones **(Khalil *et al.,* 2009).** These lncRNAs bind PRC2 to the target gene promoter and catalyze the trimethylation of histone 3 lysine residue 27 (H3K27me3**) (Wan *et al.,* 2016). Different mechanisms of long non-coding RNA action are shown in Figure 5.** figure 1 **Figure 5. Different mechanisms of long non-coding RNA action** **(****)** Certain long non-coding RNAs function as suppressors to impede the growth and spread of cancer. Pvt1b is an isoform of lncRNA that is reliant on p53 and inhibits the LC progression by downregulating the production of c-Myc. Within the lncRNA protein family, lncRNA CPS1 intronic transcript 1 (CPS1-IT1) is a newly discovered tumor suppressor. Heat shock protein 90 (HSP90) was involved in the reduction of tumor development and metastasis caused by the overexpression of CPS1-IT1. It is unclear, nonetheless, if this lncRNA functions in any way in other solid tumors **(Xiaoguang *et al.,* 2017). On the other hand,** the role of snoRNAs in lung cancer is significant **(Mei *et al.,* 2012).** The oncogenic function of SNORD42 is established as it promotes the carcinogenesis of the lungs. As reported by a TCGA data-based bioinformatic study, the U28, U30, U51, U59B, U60, U63, U104, HBI-100, HBII-142, and HBII-419 snoRNAs were up-regulated in LC, but HBII-420 was found down-regulated **(Nogueira Jorge *et al.,* 2017).** Furthermore, SNORD15A is substantially down-regulated in the tissues of non-smokers as compared to the tissues of smokers. Additionally, the research demonstrated that non-smokers exhibited uniform distribution of the snoRNAs pattern that are differentially expressed in malignant vs normal tissue. Lung cancer cells show elevated SNORD78 expression, with enhanced growth of malignant cells, enhanced epithelial-mesenchymal transition, and ultimately greater invasion potential **(Braicu *et al.,* 2019).** Recently discovered ncRNAs, circRNAs, function as either oncogenes or tumor suppressors. For example, circCDYL is underexpressed and favorably linked with patient survival in triple-negative breast cancer, colon cancer, and bladder cancer. It is now increasingly being reported and ensuring that new biomarkers for NSCLC diagnosis and prognosis can be derived from lncRNAs **(Olivero *et al.,* 2020; Yan and Bu, 2021).** **MALAT1** In 1997, research identifying numerous transcripts derived from the genomic locus generating multiple endocrine neoplasia (MEN) type 1 designated the transcript of the MALAT1 gene as an \"alpha\" transcript **(Ulitsky *et al.,* 2011).** A transcript linked to metastasis in patients with early-stage NSCLC was discovered in 2003. This transcript was identified as lncRNA MALAT1 and it was found to increase in cancer cells. The tRNA maturation route forms the 3′ end of MALAT1 RNA, which does not have a poly(A) tail. RNase P and RNase Z, tRNA processing endonucleases break the tRNA-like structure present at the 3′ end of the initial transcript **(Wilusz *et al.,* 2012).** It results in the formation of smaller MALAT1-associated small cytoplasmic RNA (mascRNA) via processing of the MALAT1 transcript as shown in Figure 6 **(Yoshimoto *et al.,* 2016).**  **Figure 6. Processing of the MALAT1 transcript and mascRNA formation** (https://www.sciencedirect.com/science/article/pii/S1874939915002060**)** MALAT1 is a predictor of survival for patients with squamous cell carcinoma or stage I lung adenocarcinoma. It has a close relationship with the histology and stage of metastasis in NSCLC patients. Nowadays, it has been discovered that the rs3200401 C\>T variation of the lncRNA MALAT1 can act as a viable prognostic biomarker for the survival prediction of advanced LC patients. This was achieved by genotyping the gene using the single nucleotide polymorphism (SNP) rs3200401. Using a gene-targeting technique based on zinc finger nucleases (ZFNs), MALAT1 was knocked down in A549 cells, revealing its role as a regulator of the metastatic phenotype of LC cells **(Ye *et al.,* 2020**). **H19** Located on chromosome 11p15.5, H19 is a 2.3 kb intergenic lncRNA that is maternally expressed. It is also one of the earliest lncRNAs known to imprint. H19 is a significant player in the development of tumors and embryos and consists of five exons and four introns **(Zhao *et al.,* 2019).** Numerous malignancies have been linked to H19, including neuroblastoma (NB), pancreatic cancer (PC), bladder cancer (BC), ovarian cancer (OC), gastric cancer (GC), colorectal cancer (CRC), and lung cancer (LC). Research findings indicate a considerable increase in H19 expression in LC tissues relative to neighboring tissues **(Guo *et al.,* 2019).** Poor prognosis, reduced disease-free survival (DFS), and transition from early stages to metastasis are all linked to H19 overexpression in LC **(Chi *et al.,* 2019).** LncRNA H19 is up-regulated in hypoxic stress and is important for the formation of human tumors. It was also shown to be upregulated and to have significant involvement in the carcinogenesis of stomach cancer. The growth of stomach cancer cells is aided by its overexpression. H19 can promote bladder cancer metastasis by blocking the production of E-cadherin and interacting with EZH2 **(Cui *et al.,* 2015).** In LC cells, it is known that c-Myc directly regulates H19. Furthermore, it was revealed that the mineral dust-induced gene (mdig) demethylated H3K9me3 on the histone H3 peptide, which is an essential regulator and epigenetic marker of heterochromatin **(Cui *et al.,* 2015).** H19 rs2107425 was significantly linked to LC susceptibility in people under 50 years of age, and H19 rs2839698 showed a correlation with the response to platinum-based chemotherapy in SCLC, according to a statistical analysis of lncRNA genetic polymorphisms **(Yoshimura *et al.,* 2018).** It has been established that H19 may bind to miR-625-5p which may accelerate the development of NSCLC. This lncRNA may modulate miR-625-5p to impact CPSF7 expression during the lung cancer process **(Wang *et al.,* 2021).** **CASC** Numerous cancer-related CASCs that influence cell proliferation, migration, invasion, and apoptosis can either promote or impede tumor growth. These CASCs are members of the cancer susceptibility candidate family of lncRNAs **(Han *et al.,* 2024**). CASC2 increases the sensitivity to chemotherapeutics by acting as a tumor suppressor for several malignancies. It has been demonstrated to induce apoptosis and necrosis in a variety of cancer chemotherapy regimens **(Liu *et al.,* 2019).** However, not much is known regarding the chemotherapy sensitization mediated by CASC2 and its upstream transcription factor in NSCLC. *In vitro* cisplatin-resistant cell line proliferation and *in vivo* tumor development are both inhibited by the overexpression of CASC2 in NSCLC cells. Interferon regulatory factor 2 (IRF2), which is regulated by miRNA, allows CASC2 to change the biological behavior of tumor cells **(Ando *et al.,* 2016).** Furthermore, the upstream regulatory component of CASC2 has been predicted and confirmed to be ELF1 **(Xiao *et al.,* 2020).** It was discovered through multivariate studies that the expression of CASC2 functions as an independent predictor of overall survival in NSCLC patients. Moreover, the formation of xenograft NSCLC tumors in mice or *in vitro* proliferation of human NSCLC cell lines were both markedly suppressed by CASC2 overexpression. The results indicated that CASC2 has a role in the onset and progression of NSCLC and that it might be used as a novel therapeutic target and a possible diagnostic tool for NSCLC patients **(Palmieri *et al.,* 2017). O**n chromosome 8, the 1,316 kb long lncRNA CASC9.5 is located. A recent investigation using second-generation sequencing revealed that CASC9.5 is implicated in the development of lung ADC and acts as a non-coding proto-oncogene **(Thiery *et al.,* 2009).** According to the latest research, lung adenocarcinoma tissues had a much greater expression level of CASC9.5 than para-cancerous tissues. A correlation between the CASC9.5 expression levels and tumor size, tumor TNM (tumor, node, and metastasis) stage, and lymph node metastasis was investigated. Additionally, CASC9.5 may control cell division and metastasis by controlling cyclin D1, β-catenin, N-cadherin, and E-cadherin **(Zhou *et al.,* 2018).** **X inactivated-specific transcript (XIST)** XIST is significantly overexpressed in NSCLC cell lines and tissues. It can promote cell migration, invasion, and proliferation by epigenetically suppressing KLF2, a member of the KLF family with zinc-finger domains Cys2/His2 **(Zhang *et al.,* 2015).** KLF2 is down-regulated in a variety of cancer types with tumor-suppressive characteristics due to its inhibitory effects on cell proliferation expression in NSCLC cells **(Fang *et al.,* 2016).** The expression of XIST was elevated in NSCLC tissues and cell lines. On the other hand, XIST knockdown supressed tumor development *in vivo* and decreased NSCLC invasion and proliferation *in vitro*. Ultimately, it was discovered that miR-186-5p inhibited XIST\'s carcinogenic properties in NSCLC cells. As a result, XIST can serve as a unique target for the management of NSCLC **(Wang *et al.,* 2017**). In a study, the levels of XIST and HIF1A-AS1 were found much higher in the serum and tumor tissues, respectively, as compared to the control group. Also, a greater positive diagnosis rate of NSCLC was observed in the combination of serum XIST and HIF1A-AS1 than in each biomarker alone. Therefore, elevated serum levels of HIF1A-AS1 and XIST might be utilised as a predictive screening biomarker in NSCLC cases. Because of its strong expression in NSCLC tissues and serum, XIST may act as a diagnostic marker for the disease **(Tantai *et al.,* 2015**). By lowering the nuclear transfer of SMAD2, XIST can increase proliferation and mediate DDP resistance in NSCLC. This, in turn, inhibits pyroptosis and apoptosis by preventing the transcription of NLRP3 and p53, respectively. These results may direct future efforts to prevent DDP resistance in NSCLC and maybe other types of tumor. They also offer possible novel biomarkers to evaluate the effectiveness of DDP treatment **(Xu *et al.,* 2020).** Through the ability to sponge different microRNAs, XIST can act as a tumor suppressor or oncogene. By binding to miR-449a competitively, XIST has been shown to control the E-cadherin and Bcl-2 expression, which can control the development of NSCLC cells **(Chang *et al.,* 2017).** Furthermore, by overexpression of E-cadherin and by controlling the XIST/miR-181a/PTEN pathway, XIST has been shown to suppress the proliferation of cells and metastasis in HCC. It was demonstrated that XIST caused carcinogenesis by directly interacting with EZH2 to silence KLF2 expression. This finding raised the chances that XIST may act as a useful diagnostic and therapeutic target for NSCLC **(Zhou *et al.,* 2018**). It has been shown that XIST can target miR-367/141, miR-139, and miR-186-5p and acts as the sponge for miR-152, miR-92b, and miR-497 **(Li *et al.,* 2018). In another study,** it has been observed that XIST may considerably suppress miR-16 expression which further suppresses NSCLC invasion, proliferation, and EMT **(Zhou *et al.,* 2019).** **MEG3** Maternally expressed and imprinted lncRNA MEG3 is a component of the DLK1-MEG3 locus on human chromosome 14q32 **(Lu *et al.,* 2013).** It has been found that increased MEG3 expression controls p53 activation, which in turn controls NSCLC cell proliferation and apoptosis**.** Tumor suppressor MEG3 is expressed at reduced levels in a variety of malignancies, including gastric, colorectal, prostate, and NSCLC **(Liu *et al.,* 2015)**. It has been discovered that miR-205 is up-regulated in NSCLC and promotes the emergence of malignant phenotypes **(Yin *et al.,* 2015).** Additionally, a bioinformatics investigation suggested that MEG3 and miR-205-5p might interact. In human NSCLC tissues and cells, miR-205-5p expression was higher and MEG3 expression was lower **(Wang *et al.,* 2017).** **RNA MODIFICATION** Post-transcriptional RNA modifications, also known as the \"epitranscriptomic modifications,\" are the epigenetic modifications at the RNA level. These modifications can be regulated dynamically and reversibly by some specific enzymes known as \"writer\" (installer), \"reader\" (translator), and \"eraser\" (demodifier) **(Ontiveros *et al.,* 2019).** Because these enzymes can modulate RNA functions, they represent a class of potential drug targets. All types of RNAs have some main RNA modification which may be found in many different forms, such as N1-methyladenosine (m^1^A), 5-methylcytidine (m^5^C), N6-methyladenosine (m^6^A), N7-methylguanidine (m^7^G), and 2-O-methylation (Nm). It is interesting to note that different RNA species may have distinct set of methyltransferases and demethylases acting as their writers and erasers even when they target the same sort of methylation **(Haruehanroengra *et al.,* 2020). Figure 7 shows the reversibility of RNA methylation along with different epitranscriptomic regulators.** Fig. 1 **Figure 7. A schematic diagram showing the reversibility of RNA methylation along with different epitranscriptomic regulators (https://www.sciencedirect.com/science/article/pii/S0888754321002305\#bb0060)** In eukaryotic mRNAs, m^6^A modification is the most prevalent modification. The physiological relevance of the m^6^A alteration was not clear until recently, despite its discovery in the 1970s **(Rahnamoun *et al.,* 2019).** Since m^6^A is crucial for mRNA stability, splicing, translation efficiency, and other processes, the homeostasis of an mRNA molecule\'s methylation level is strictly controlled. Previous reports have indicated that Methyltransferase Like Protein 3 (METTL3), is the primary element of the m^6^A methyltransferase complex **(Li *et al.,* 2021).** On particular RNA types, distinct methyl transferases carry out the installation of m^6^A. On mRNAs, the majority of m^6^A modification is carried out by METTL3, and less so by METTL16, according to loss-of-function tests conducted on a variety of enzymes **(Geula *et al.,* 2015).** Furthermore, it has been discovered that A1832 of 18S rRNA, A4220 of 28S rRNA, and U6SnRNA are methylated by METTL5, ZCCHC4 (Zinc Finger CCHC-Type Containing 4), and METTL16, respectively. S-adenosylmethionine (SAM) provides the --CH~3~ group needed for the methylation processes that METTL3 and METTL16 carry out **(Khan *et al.,* 2021).** WTAP forms a complex with METTL3/14 that is attached to the nucleus and catalyzes m^6^A methyltransferase. The enzyme m^6^A demethylase, also known as m^6^A \"erasers,\" which contains fat mass, obesity-associated protein (FTO), and alkB homologue 5 (ALKBH5), has the ability to reverse the dynamic methylation of m^6^A **(Ping *et al.,* 2014).** FTO is related to increased fat mass and shares motifs with Fe (II)- and 2-oxoglutarate-dependent oxygenases. FTO has a strong oxidative demethylation activity that lowers mRNA m^6^A levels **(Yi *et al.,* 2020).** The tissues of LC patients with advanced stages of the disease had greater amounts of METTL3. In H1299 cells, METTL3 promotes the translation of target mRNAs by attracting eIF, the eukaryotic translation initiation factor, to the translation initiation complex **(Chen *et al.,* 2020).** Certain elements of the multi-subunit eIF3 complex are directly interacting with it. The oncogenic transformation of A549 cells, the production of densely packed polyribosomes, and the promotion of translation all depend on the METTL3-eIF3H interaction **(Choe *et al.,* 2018).** When the METTL3-eIF3H connection is disrupted, METTL3 is no longer able to alter polysome conformation, increase oncogenic transformation, or promote translation **(Wang *et al.,* 2021).** The m^5^C modification is also a common RNA modification and is found in mRNA as well as ncRNAs such as tRNA, rRNA, lncRNA, snRNA, miRNA, and enhancer RNA (eRNA). It is created when an active --CH~3~ from the donor, typically S-adenosyl-methionine (SAM), is added to the 5^th^ carbon of the cytosine base in RNA **(Song *et al.,* 2022).** A number of important mediator proteins, termed \"writers\" \[NOP2/Sun RNA methyltransferase (NSUN1-7), and tRNA aspartic acid methyltransferase 1 (TRDMT1)\], \"readers\" \[Y-box binding protein 1 (YBX1) and Aly/REF export factor (ALYREF)\] and \"erasers\" \[tetmethylcytosine dioxygenase (TET1-3)\] can catalyze the m^5^C RNA methylation in a dynamic manner **(Hussain *et al.,* 2013).** Human disorders, including cancer, have been linked to m^5^C dysregulation and dysfunction **(Liu *et al.,* 2022).** A prognostic risk model for seven m^6^A/m^5^C genes (IGF2BP1, NPLOC4, METTL3, NSUN3, NSUN7, RBM15, and YTHDF1) was developed to accurately stratify patients with early-stage lung ADC. The high-risk score indicated a poor prognosis in case of early-stage lung ADC, which could be effectively utilized in clinical evaluation and treatment plans. In early-stage lung ADC, m^6^A/m^5^C modification may control the expression of critical prognostic genes and oncogene changes. The m^6^A/m^5^C regulated genes may be implicated in signaling pathways, including mismatch repair and DNA replication, and the development of T cell fatigue, which may further worsen the prognosis and advance early-stage LUAD (**Tian *et al.,* 2023).** **CONCLUSION** In summary, there are many different causes of cancer, including genetic alterations, environmental factors like air pollution and smoking, and lifestyle choices. Lung cancer in particular is still a major global health concern. Smoking is a major risk factor for LC, which presents a serious threat to public health due to its numerous subtypes, including small cell and non-small cell carcinoma. Domestic exposure to radon, occupational exposures (like asbestos), estrogen, domestic fuel smoke, infections (like HPV and tuberculosis), genetic risk factors, and epigenetic regulators (like DNA methylation and histone modifications) are all associated with lung cancer development. Additionally, by comprehending the complex link between cancer and DNA methylation, targeted medicines that particularly target the aberrant methylation patterns in cancer cells can be developed. This individualized approach to treatment has a lot of potential to enhance patient outcomes and lessen the negative effects of conventional chemotherapy. Furthermore, new findings in the field of epigenetics continue to shed light on the significance of DNA methylation in the advancement of cancer, information that will be useful in the creation of innovative diagnostic techniques and treatment plans. Researchers and medical professionals can strive toward more individualized and successful treatments for people with lung cancer and other cancers by utilizing the potential of epigenetics. In addition, focusing on histone alterations has become a viable approach in the fight against lung cancer. Preclinical research and clinical trials have demonstrated the promise of inhibitors of histone deacetylases and histone methyltransferases as lung cancer therapeutics. Tumor growth and metastasis can be prevented by reprogramming gene expression patterns and restoring normal chromatin structure through the manipulation of histone modifications. In general, knowing how histone modification functions in lung cancer gives new prospects for the development of targeted therapeutics as well as insightful knowledge about the molecular pathways underlying the disease. To fully realize the promise of histone modification as a therapeutic target in LC, more studies in this area are necessary. An acetyl group is added to the lysine residues on histone proteins as a result of a post-translational alteration known as histone acetylation. Histone acetyltransferases (HATs) catalyze this alteration, which is linked to an open chromatin structure and increases the accessibility of DNA to transcription factors and other regulatory proteins. In the end, this results in improved gene expression. Histone deacetylases cause a closed chromatin shape and the suppression of gene expression by removing acetyl groups from histones. HDAC1 in particular has been linked to tumor growth, metastasis, and chemotherapy resistance. It has been discovered to be overexpressed in several types of cancer. HDAC1 activity inhibition has shown potential as a therapeutic approach for cancer treatment. Another significant post-translational alteration that controls chromatin dynamics and gene expression is histone phosphorylation, particularly at serine residues. Histone phosphorylation is frequently stimulus-dependent and can be caused by many different signaling cascades. This alteration has a role in the DNA damage response and the activation of particular genes in response to cellular stress and environmental stimuli. Histone phosphorylation can draw in proteins that are important for chromatin remodeling and DNA repair, enabling healthy cellular reactions to damage DNA. Apart from alterations to the histone structure, lncRNAs have been identified as important participants in the initiation and progression of tumor. Rather than encoding proteins, these RNA molecules control the expression of genes at different levels. Two well-researched lncRNAs that have been connected to cancer are MALAT1 and H19. MALAT1 is increased in multiple cancer types and stimulates the growth, invasion, and metastasis of tumors by several different methods, including manipulation of chromatin structure, alternative splicing, and regulation of gene expression. Conversely, H19 plays a role in cell division, proliferation, and apoptosis; its dysregulation has been linked to several malignancies. The potential of histone modifications and lncRNAs as biomarkers for early detection, prognosis, and therapeutic targets is underscored by their role in the initiation and progression of cancer. Comprehending the complex regulatory pathways facilitated by these molecules can yield a significant understanding of the fundamental molecular mechanisms of cancer and could potentially result in the creation of innovative and more potent cancer therapies. A number of lncRNAs are related to the initiation and progression of NSCLC, including CASC2, CASC9.5, XIST, and MEG3. These lncRNAs can function as either tumor suppressors or oncogenes, and their expression levels may be utilized as possible therapeutic targets for NSCLC as well as diagnostic markers. Drug targets may include posttranscriptional RNA modifications like m^6^A and m^5^C, which are crucial for controlling RNA activities. These alterations affect mRNA stability, splicing, translation efficiency, and other functions. They are dynamically controlled by particular enzymes. A predictive risk model for m^6^A and m^5^C changes can be used to assess and treat patients with early-stage lung cancer. **REFERENCES** 1. Aghdassi, A., Sendler, M., Guenther, A., Mayerle, J., Behn, C. O., Heidecke, C. D., \... & Weiss, F. U. (2012). Recruitment of histone deacetylases HDAC1 and HDAC2 by the transcriptional repressor ZEB1 downregulates E-cadherin expression in pancreatic cancer. *Gut*, *61*(3), 439-448. 2. Akhtar, N., & Bansal, J. G. (2017). Risk factors of Lung Cancer in nonsmoker. *Current problems in cancer*, *41*(5), 328-339. 3. Alaskhar Alhamwe, B., Khalaila, R., Wolf, J., von Bülow, V., Harb, H., Alhamdan, F., \... & Potaczek, D. P. (2018). Histone modifications and their role in epigenetics of atopy and allergic diseases. *Allergy, Asthma & Clinical Immunology*, *14*, 1-16. 4. Ali, M., Yan, K., Lalonde, M. E., Degerny, C., Rothbart, S. B., Strahl, B. D., \... & Kutateladze, T. G. (2012). Tandem PHD fingers of MORF/MOZ acetyltransferases display selectivity for acetylated histone H3 and are required for the association with chromatin. *Journal of molecular biology*, *424*(5), 328-338. 5. Ando, M., Kawazu, M., Ueno, T., Koinuma, D., Ando, K., Koya, J., \... & Mano, H. (2016). Mutational landscape and antiproliferative functions of ELF transcription factors in human cancer. *Cancer Research*, *76*(7), 1814-1824. 6. Ansari, J., Shackelford, R. E., & El-Osta, H. (2016). Epigenetics in non-small cell lung cancer: from basics to therapeutics. *Translational lung cancer research*, *5*(2), 155. 7. Audia, J. E., & Campbell, R. M. (2016). Histone modifications and cancer. *Cold Spring Harbor perspectives in biology*, *8*(4), a019521. 8. Augert, A., Zhang, Q., Bates, B., Cui, M., Wang, X., Wildey, G., \... & MacPherson, D. (2017). Small cell lung cancer exhibits frequent inactivating mutations in the histone methyltransferase KMT2D/MLL2: CALGB 151111 (Alliance). *Journal of Thoracic Oncology*, *12*(4), 704-713. 9. Aylon, Y., Bublik, D. R., Moskovits, N., Toperoff, G., Azaiza, D., Biagoni, F., \... & Oren, M. (2015). Reactivation of epigenetically silenced miR-512 and miR-373 sensitizes lung cancer cells to cisplatin and restricts tumor growth. *Cell Death and Differentiation*, *22*(8), 1328-1340. 10. Baek, S. H. (2011). When signaling kinases meet histones and histone modifiers in the nucleus. *Molecular cell*, *42*(3), 274-284. 11. Baglietto, L., Ponzi, E., Haycock, P., Hodge, A., Bianca Assumma, M., Jung, C. H., \... & Severi, G. (2017). DNA methylation changes measured in pre‐diagnostic peripheral blood samples are associated with smoking and lung cancer risk. *International journal of cancer*, *140*(1), 50-61. 12. Bajbouj, K., Al-Ali, A., Ramakrishnan, R. K., Saber-Ayad, M., & Hamid, Q. (2021). Histone modification in NSCLC: molecular mechanisms and therapeutic targets. *International journal of molecular sciences*, *22*(21), 11701. 13. Balmes, J. R. (2019). Household air pollution from domestic combustion of solid fuels and health. *Journal of Allergy and Clinical Immunology*, *143*(6), 1979-1987. 14. Bedford, M. T., & Clarke, S. G. (2009). Protein arginine methylation in mammals: who, what, and why. *Molecular cell*, *33*(1), 1-13. 15. Bhan, A., Soleimani, M., & Mandal, S. S. (2017). Long noncoding RNA and cancer: a new paradigm. *Cancer research*, *77*(15), 3965-3981. 16. Booth, H., & WH, H. P. (2007). Classification and nomenclature of all human homeobox genes. 17. Bowman, G. D., & Poirier, M. G. (2014). Post-translational modifications of histones that influence nucleosome dynamics. *Chemical reviews*, *115*(6), 2274-2295. 18. Braicu, C., Zimta, A. A., Harangus, A., Iurca, I., Irimie, A., Coza, O., & Berindan-Neagoe, I. (2019). The function of non-coding RNAs in lung cancer tumorigenesis. *Cancers*, *11*(5), 605. 19. Bray, F., Laversanne, M., Sung, H., Ferlay, J., Siegel, R. L., Soerjomataram, I., & Jemal, A. (2024). Global cancer statistics 2022: GLOBOCAN estimates of incidence and mortality worldwide for 36 cancers in 185 countries. *CA: a cancer journal for clinicians*, *74*(3), 229-263. 20. Brenner, D. R., McLaughlin, J. R., & Hung, R. J. (2011). Previous lung diseases and lung cancer risk: a systematic review and meta-analysis. *PloS one*, *6*(3), e17479. 21. Cai, X., Janku, F., Zhan, Q., & Fan, J. B. (2015). Accessing genetic information with liquid biopsies. *Trends in Genetics*, *31*(10), 564-575. 22. Cao, L. L., Song, X., Pei, L., Liu, L., Wang, H., & Jia, M. (2017). Histone deacetylase HDAC1 expression correlates with the progression and prognosis of lung cancer: A meta-analysis. *Medicine*, *96*(31), e7663. 23. Carter, B. D., Abnet, C. C., Feskanich, D., Freedman, N. D., Hartge, P., Lewis, C. E., \... & Jacobs, E. J. (2015). Smoking and mortality---beyond established causes. *New England journal of medicine*, *372*(7), 631-640. 24. Castro, D., Moreira, M., Gouveia, A. M., Pozza, D. H., & De Mello, R. A. (2017). MicroRNAs in lung cancer. *Oncotarget*, *8*(46), 81679. 25. Chang, S., Chen, B., Wang, X., Wu, K., & Sun, Y. (2017). Long non-coding RNA XIST regulates PTEN expression by sponging miR-181a and promotes hepatocellular carcinoma progression. *BMC cancer*, *17*, 1-14. 26. Chen, J., Moir, D., & Whyte, J. (2012). Canadian population risk of radon induced lung cancer: a re-assessment based on the recent cross-Canada radon survey. *Radiation protection dosimetry*, *152*(1-3), 9-13. 27. Chen, T. F., Hao, H. F., Zhang, Y., Chen, X. Y., Zhao, H. S., Yang, R., \... & Liu, S. X. (2022). HBO1 induces histone acetylation and is important for non-small cell lung cancer cell growth. *International Journal of Biological Sciences*, *18*(8), 3313. 28. Chen, W. W., Qi, J. W., Hang, Y., Wu, J. X., Zhou, X. X., Chen, J. Z., \... & Wang, H. H. (2020). Simvastatin is beneficial to lung cancer progression by inducing METTL3-induced m6A modification on EZH2 mRNA. *European Review for Medical & Pharmacological Sciences*, *24*(8). 29. Chen, Y., Liu, X., Li, Y., Quan, C., Zheng, L., & Huang, K. (2018). Lung cancer therapy targeting histone methylation: opportunities and challenges. *Computational and structural biotechnology journal*, *16*, 211-223. 30. Chen, Z., Ye, X., Tang, N., Shen, S., Li, Z., Niu, X., \... & Xu, L. (2014). The histone acetylranseferase h MOF acetylates Nrf 2 and regulates anti‐drug responses in human non‐small cell lung cancer. *British journal of pharmacology*, *171*(13), 3196-3211. 31. Chen, Z., Zhang, Y., Yang, J., Jin, M., Wang, X. W., Shen, Z. Q., \... & Li, J. W. (2011). Estrogen promotes benzo \[a\] pyrene-induced lung carcinogenesis through oxidative stress damage and cytochrome c-mediated caspase-3 activation pathways in female mice. *Cancer letters*, *308*(1), 14-22. 32. Cheng, X. (2014). Structural and functional coordination of DNA and histone methylation. *Cold Spring Harbor perspectives in biology*, *6*(8), a018747. 33. Chi, Y., Wang, D., Wang, J., Yu, W., & Yang, J. (2019). Long non-coding RNA in the pathogenesis of cancers. *Cells*, *8*(9), 1015. 34. Choe, J., Lin, S., Zhang, W., Liu, Q., Wang, L., Ramirez-Moya, J., \... & Gregory, R. I. (2018). mRNA circularization by METTL3--eIF3h enhances translation and promotes oncogenesis. *Nature*, *561*(7724), 556-560. 35. Chrun, E. S., Modolo, F., & Daniel, F. I. (2017). Histone modifications: A review about the presence of this epigenetic phenomenon in carcinogenesis. *Pathology-Research and Practice*, *213*(11), 1329-1339. 36. Contreras-Sanzón, E., Prado-Garcia, H., Romero-Garcia, S., Nuñez-Corona, D., Ortiz-Quintero, B., Luna-Rivero, C., \... & Carlos-Reyes, Á. (2022). Histone deacetylases modulate resistance to the therapy in lung cancer. *Frontiers in Genetics*, *13*, 960263. 37. Corrales, L., Rosell, R., Cardona, A. F., Martin, C., Zatarain-Barron, Z. L., & Arrieta, O. (2020). Lung cancer in never smokers: The role of different risk factors other than tobacco smoking. *Critical reviews in oncology/hematology*, *148*, 102895. 38. Cui, J., Mo, J., Luo, M., Yu, Q., Zhou, S., Li, T., \... & Luo, W. (2015). c-Myc-activated long non-coding RNA H19 downregulates miR-107 and promotes cell cycle progression of non-small cell lung cancer. *International journal of clinical and experimental pathology*, *8*(10), 12400. 39. de Groot, P. M., Wu, C. C., Carter, B. W., & Munden, R. F. (2018). The epidemiology of lung cancer. *Translational lung cancer research*, *7*(3), 220. 40. Dhillon, A. S., Hagan, S., Rath, O., & Kolch, W. (2007). MAP kinase signalling pathways in cancer. *Oncogene*, *26*(22), 3279-3290. 41. Dor, Y., & Cedar, H. (2018). Principles of DNA methylation and their implications for biology and medicine. *The Lancet*, *392*(10149), 777-786. 42. D\'Oto, A., Tian, Q. W., Davidoff, A. M., & Yang, J. (2016). Histone demethylases and their roles in cancer epigenetics. *Journal of medical oncology and therapeutics*, *1*(2), 34. 43. Duma, N., Santana-Davila, R., & Molina, J. R. (2019, August). Non--small cell lung cancer: epidemiology, screening, diagnosis, and treatment. In *Mayo Clinic Proceedings* (Vol. 94, No. 8, pp. 1623-1640). Elsevier. 44. Durham, A. L., & Adcock, I. M. (2015). The relationship between COPD and lung cancer. *Lung cancer*, *90*(2), 121-127. 45. Eckschlager, T., Plch, J., Stiborova, M., & Hrabeta, J. (2017). Histone deacetylase inhibitors as anticancer drugs. *International journal of molecular sciences*, *18*(7), 1414. 46. Fang, J., Sun, C. C., & Gong, C. (2016). Long noncoding RNA XIST acts as an oncogene in non-small cell lung cancer by epigenetically repressing KLF2 expression. *Biochemical and biophysical research communications*, *478*(2), 811-817. 47. Fang, R., Xiao, T., Fang, Z., Sun, Y., Li, F., Gao, Y., \... & Ji, H. (2012). MicroRNA-143 (miR-143) regulates cancer glycolysis via targeting hexokinase 2 gene. *Journal of Biological Chemistry*, *287*(27), 23227-23235. 48. Fang, S., Shen, Y., Chen, B., Wu, Y., Jia, L., Li, Y., \... & Chen, Q. (2018). H3K27me3 induces multidrug resistance in small cell lung cancer by affecting HOXA1 DNA methylation via regulation of the lncRNA HOTAIR. *Annals of translational medicine*, *6*(22). 49. Farooq, M., & Herman, J. G. (2020). Noninvasive diagnostics for early detection of lung cancer: challenges and potential with a focus on changes in DNA methylation. *Cancer Epidemiology, Biomarkers & Prevention*, *29*(12), 2416-2422. 50. Feng, H., Zhang, Z., Qing, X., Wang, X., Liang, C., & Liu, D. (2016). Promoter methylation of APC and RAR-β genes as prognostic markers in non-small cell lung cancer (NSCLC). *Experimental and molecular pathology*, *100*(1), 109-113. 51. Gagou, M. E., Zuazua-Villar, P., & Meuth, M. (2010). Enhanced H2AX phosphorylation, DNA replication fork arrest, and cell death in the absence of Chk1. *Molecular biology of the cell*, *21*(5), 739-752. 52. Gan, J., Huang, M., Wang, W., Fu, G., Hu, M., Zhong, H., \... & Cao, Q. (2024). Novel genome-wide DNA methylation profiling reveals distinct epigenetic landscape, prognostic model and cellular composition of early-stage lung adenocarcinoma. *Journal of Translational Medicine*, *22*(1), 428. 53. Gates, P., Jaffe, A., & Copeland, J. (2014). Cannabis smoking and respiratory health: consideration of the literature. *Respirology*, *19*(5), 655-662. 54. Geula, S., Moshitch-Moshkovitz, S., Dominissini, D., Mansour, A. A., Kol, N., Salmon-Divon, M., \... & Hanna, J. H. (2015). m6A mRNA methylation facilitates resolution of naïve pluripotency toward differentiation. *Science*, *347*(6225), 1002-1006. 55. Giaginis, C., Alexandrou, P., Delladetsima, I., Giannopoulou, I., Patsouris, E., & Theocharis, S. (2014). Clinical significance of histone deacetylase (HDAC)-1, HDAC-2, HDAC-4, and HDAC-6 expression in human malignant and benign thyroid lesions. *Tumor Biology*, *35*, 61-71. 56. Guo, S., Zhu, Q., Jiang, T., Wang, R., Shen, Y., Zhu, X., \... & He, D. Y. (2017). Genome-wide DNA methylation patterns in CD4+ T cells from Chinese Han patients with rheumatoid arthritis. *Modern rheumatology*, *27*(3), 441-447. 57. Guo, T., Li, J., Zhang, L., Hou, W., Wang, R., Zhang, J., & Gao, P. (2019). Multidimensional communication of microRNAs and long non-coding RNAs in lung cancer. *Journal of Cancer Research and Clinical Oncology*, *145*, 31-48. 58. Ha, M., & Kim, V. N. (2014). Regulation of microRNA biogenesis. *Nature reviews Molecular cell biology*, *15*(8), 509-524. 59. Han, H., Huang, H., Chen, A. P., Tang, Y., Huang, X., & Chen, C. (2024). High CASC expression predicts poor prognosis of lung cancer: A systematic review with meta-analysis. *PloS one*, *19*(4), e0292726. 60. Hansen, K. D., Timp, W., Bravo, H. C., Sabunciyan, S., Langmead, B., McDonald, O. G., \... & Feinberg, A. P. (2011). Increased methylation variation in epigenetic domains across cancer types. *Nature genetics*, *43*(8), 768-775. 61. Haruehanroengra, P., Zheng, Y. Y., Zhou, Y., Huang, Y., & Sheng, J. (2020). RNA modifications and cancer. *RNA biology*, *17*(11), 1560-1575. 62. Hecht, S. S., & Szabo, E. (2014). Fifty years of tobacco carcinogenesis research: from mechanisms to early detection and prevention of lung cancer. *Cancer prevention research*, *7*(1), 1-8. 63. Heyn, H., & Esteller, M. (2012). DNA methylation profiling in the clinic: applications and challenges. *Nature Reviews Genetics*, *13*(10), 679-692. 64. Ho, K. F., Ho, S. S. H., Huang, R. J., Chuang, H. C., Cao, J. J., Han, Y., \... & Zhang, R. (2016). Chemical composition and bioreactivity of PM2. 5 during 2013 haze events in China. *Atmospheric Environment*, *126*, 162-170. 65. Hoang, P. H., & Landi, M. T. (2022). DNA methylation in lung cancer: mechanisms and associations with histological subtypes, molecular alterations, and major epidemiological factors. *Cancers*, *14*(4), 961 66. Hu, C., Meiners, S., Lukas, C., Stathopoulos, G. T., & Chen, J. (2020). Role of exosomal microRNAs in lung cancer biology and clinical applications. *Cell Proliferation*, *53*(6), e12828 67. Hu, H., Zhou, Y., Zhang, M., & Ding, R. (2019). Prognostic value of RASSF1A methylation status in non-small cell lung cancer (NSCLC) patients: A meta-analysis of prospective studies. *Biomarkers*, *24*(3), 207-216. 68. Huang, T., Chen, X., Hong, Q., Deng, Z., Ma, H., Xin, Y., \... & Duan, S. (2015). Meta-analyses of gene methylation and smoking behavior in non-small cell lung cancer patients. *Scientific reports*, *5*(1), 8897. 69. Hussain, S., Aleksic, J., Blanco, S., Dietmann, S., & Frye, M. (2013). Characterizing 5-methylcytosine in the mammalian epitranscriptome. *Genome biology*, *14*, 1-10. 70. Hutarew, G., Alinger-Scharinger, B., Sotlar, K., & Kraus, T. F. (2024). Genome-Wide Methylation Analysis in Two Wild-Type Non-Small Cell Lung Cancer Subgroups with Negative and High PD-L1 Expression. *Cancers*, *16*(10), 1841. 71. Iizuka, M., Takahashi, Y., Mizzen, C. A., Cook, R. G., Fujita, M., Allis, C. D., \... & Smith, M. M. (2009). Histone acetyltransferase Hbo1: catalytic activity, cellular abundance, and links to primary cancers. *Gene*, *436*(1-2), 108-114. 72. Inamura, K. (2017). Lung cancer: understanding its molecular pathology and the 2015 WHO classification. *Frontiers in oncology*, *7*, 193 73. Iqbal, M. A., Arora, S., Prakasam, G., Calin, G. A., & Syed, M. A. (2019). MicroRNA in lung cancer: role, mechanisms, pathways and therapeutic relevance. *Molecular aspects of medicine*, *70*, 3-20. 74. Jeltsch, A., & Jurkowska, R. Z. (2014). New concepts in DNA methylation. *Trends in biochemical sciences*, *39*(7), 310-318. 75. Jemal, A., Siegel, R., Xu, J., & Ward, E. (2010). Cancer statistics, 2010. *CA: a cancer journal for clinicians*, *60*(5), 277-300. 76. Ji, J., Zhang, Y., Redon, C. E., Reinhold, W. C., Chen, A. P., Fogli, L. K., \... & Bonner, W. M. (2017). Phosphorylated fraction of H2AX as a measurement for DNA damage in cancer cells and potential applications of a novel assay. *PloS one*, *12*(2), e0171582. 77. Jiang, C. L., He, S. W., Zhang, Y. D., Duan, H. X., Huang, T., Huang, Y. C., \... & Cao, Y. (2017). Air pollution and DNA methylation alterations in lung cancer: A systematic and comparative study. *Oncotarget*, *8*(1), 1369. 78. Jiang, C., & Pugh, B. F. (2009). Nucleosome positioning and gene regulation: advances through genomics. *Nature Reviews Genetics*, *10*(3), 161-172. 79. Jiang, X., Wang, J., Deng, X., Xiong, F., Zhang, S., Gong, Z., \... & Xiong, W. (2020). The role of microenvironment in tumor angiogenesis. *Journal of Experimental & Clinical Cancer Research*, *39*, 1-19. 80. Jiao, Y., Pawlik, T. M., Anders, R. A., Selaru, F. M., Streppel, M. M., Lucas, D. J., \... & Wood, L. D. (2013). Exome sequencing identifies frequent inactivating mutations in BAP1, ARID1A and PBRM1 in intrahepatic cholangiocarcinomas. *Nature genetics*, *45*(12), 1470-1473. 81. Kang, J. U., Koo, S. H., Kwon, K. C., Park, J. W., Shin, S. Y., Kim, J. M., & Jung, S. S. (2007). High frequency of genetic alterations in non-small cell lung cancer detected by multi-target fluorescence in situ hybridization. *Journal of Korean Medical Science*, *22*(Suppl), S47. 82. Kanwal, M., Ding, X. J., & Cao, Y. (2017). Familial risk for lung cancer. *Oncology letters*, *13*(2), 535-542. 83. Karachaliou, N., Pilotto, S., Lazzari, C., Bria, E., de Marinis, F., & Rosell, R. (2016). Cellular and molecular biology of small cell lung cancer: an overview. *Translational lung cancer research*, *5*(1), 2. 84. Khalil, A. M., Guttman, M., Huarte, M., Garber, M., Raj, A., Rivea Morales, D., \... & Rinn, J. L. (2009). Many human large intergenic noncoding RNAs associate with chromatin-modifying complexes and affect gene expression. *Proceedings of the National Academy of Sciences*, *106*(28), 11667-11672. 85. Khan, R. I. N., & Malla, W. A. (2021). m6A modification of RNA and its role in cancer, with a special focus on lung cancer. *Genomics*, *113*(4), 2860-2869. 86. Khan, S. A., Reddy, D., & Gupta, S. (2015). Global histone post-translational modifications and cancer: Biomarkers for diagnosis, prognosis and treatment? *World journal of biological chemistry*, *6*(4), 333. 87. Kim, S. H., Hwang, W. J., Cho, J. S., & Kang, D. R. (2016). Attributable risk of lung cancer deaths due to indoor radon exposure. *Annals of occupational and environmental medicine*, *28*(1), 8. 88. Kimura, H. (2013). Histone modifications for human epigenome analysis. *Journal of human genetics*, *58*(7), 439-445. 89. Kouzarides, T. (2007). Chromatin modifications and their function. *Cell*, *128*(4), 693-705. 90. Lan, R., & Wang, Q. (2020). Deciphering structure, function and mechanism of lysine acetyltransferase HBO1 in protein acetylation, transcription regulation, DNA replication and its oncogenic properties in cancer. *Cellular and Molecular Life Sciences*, *77*, 637-649. 91. Langevin, S. M., Kratzke, R. A., & Kelsey, K. T. (2015). Epigenetics of lung cancer. *Translational Research*, *165*(1), 74-90. 92. Lemjabbar-Alaoui, H., Hassan, O. U., Yang, Y. W., & Buchanan, P. (2015). Lung cancer: Biology and treatment options. *Biochimica et Biophysica Acta (BBA)-Reviews on Cancer*, *1856*(2), 189-210. 93. Lewis, D. R., Check, D. P., Caporaso, N. E., Travis, W. D., & Devesa, S. S. (2014). US lung cancer trends by histologic type. *Cancer*, *120*(18), 2883-2892. 94. Li, C., Wan, L., Liu, Z., Xu, G., Wang, S., Su, Z., \... & Zhang, H. T. (2018). Long non-coding RNA XIST promotes TGF-β-induced epithelial-mesenchymal transition by regulating miR-367/141-ZEB2 axis in non-small-cell lung cancer. *Cancer letters*, *418*, 185-195. 95. Li, G., Tian, Y., & Zhu, W. G. (2020). The roles of histone deacetylases and their inhibitors in cancer therapy. *Frontiers in cell and developmental biology*, *8*, 576946. 96. Li, H., Zhang, Y., Guo, Y., Liu, R., Yu, Q., Gong, L., \... & Wang, C. (2021). ALKBH1 promotes lung cancer by regulating m6A RNA demethylation. *Biochemical pharmacology*, *189*, 114284. 97. Li, J., Mahata, B., Escobar, M., Goell, J., Wang, K., Khemka, P., & Hilton, I. B. (2021). Programmable human histone phosphorylation and gene activation using a CRISPR/Cas9-based chromatin kinase. *Nature Communications*, *12*(1), 896. 98. Li, Y., Zhang, T., Zhang, H., Wang, X., Liu, X., Huang, Q., & Li, L. (2020). Clinical significance of P16 gene methylation in lung cancer. *Single-cell Sequencing and Methylation: Methods and Clinical Applications*, 133-142. 99. Li, Z., & Zhu, W. G. (2014). Targeting histone deacetylases for cancer therapy: from molecular mechanisms to clinical implications. *International journal of biological sciences*, *10*(7), 757. 100. Liang, R., Li, X., Li, W., Zhu, X., & Li, C. (2021). DNA methylation in lung cancer patients: Opening a\" window of life\" under precision medicine. *Biomedicine & Pharmacotherapy*, *144*, 112202. 101. Liang, W., Zhao, Y., Huang, W., Gao, Y., Xu, W., Tao, J., \... & He, J. (2019). Non-invasive diagnosis of early-stage lung cancer using high-throughput targeted DNA methylation sequencing of circulating tumor DNA (ctDNA). *Theranostics*, *9*(7), 2056. 102. Lin,