AQA Biology GCSE Cell Biology Notes PDF
Document Details
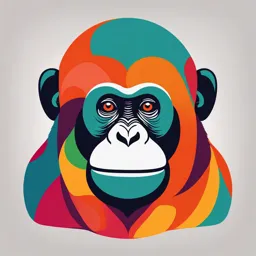
Uploaded by BenevolentTelescope
AQA
Tags
Summary
These are detailed notes on cell biology for AQA GCSE Biology. The notes cover eukaryotic and prokaryotic cells, cell structure, organelles, and orders of magnitude. Topics also include cell function and examples of specialised cells.
Full Transcript
AQA Biology GCSE Topic 1: Cell Biology Notes Content in bold is for higher tier only. Content is for both separate science and double award students unless indicated in heading....
AQA Biology GCSE Topic 1: Cell Biology Notes Content in bold is for higher tier only. Content is for both separate science and double award students unless indicated in heading. This work by PMT Education is licensed under https://bit.ly/pmt-cc https://bit.ly/pmt-edu-cc CC BY-NC-ND 4.0 https://bit.ly/pmt-cc https://bit.ly/pmt-edu https://bit.ly/pmt-cc Cell Structure (1.1) Eukaryotes and Prokaryotes (1.1.1) All living things are made of cells, which can either be prokaryotic or eukaryotic. Animal and plant cells are eukaryotic. They have a: Cell membrane Cytoplasm Nucleus containing DNA Bacterial cells are prokaryotic and are much smaller. They have a: Cell wall Cell membrane Cytoplasm Single circular strand of DNA and plasmids (small rings of DNA found in the cytoplasm) The structures mentioned above (e.g. cell membrane) are examples of organelles - structures in a cell that have different functions Cells are extremely small, and we can use orders of magnitude to understand how much bigger or smaller one is from another: If an object is 10 times bigger than another then we say it is 101 times bigger. 3 If an object is 1000 times bigger than another then we say it is 10 times bigger. If an object is 10 times smaller than another then we say it is 10-1 times smaller. Prefixes go before units of measurement (such as ‘metres’) to show the multiple of the unit. Prefix Multiply unit by... Centi 0.01 Milli 0.001 Micro 0.000, 001 Nano 0.000, 000, 001 https://bit.ly/pmt-cc https://bit.ly/pmt-edu https://bit.ly/pmt-cc Animals and Plants (1.1.2) The subcellular structures inside cells all have a specific function. In animal and plant cells... Structure Function Nucleus Contains DNA coding for a particular protein needed to build new cells. Enclosed in a nuclear membrane. Cytoplasm Liquid substance in which chemical reactions occur. Contains enzymes (biological catalysts, i.e. proteins that speed up the rate of reaction). Organelles are found in it Cell membrane Controls what enters and leaves the cell Mitochondria Where aerobic respiration reactions occur, providing energy for the cell Ribosomes Where protein synthesis occurs. Found on a structure called the rough endoplasmic reticulum. Only in plant cells… Structure Function Chloroplasts Where photosynthesis takes place, providing food for the plant Contains chlorophyll pigment (which makes it green) which harvests the light needed for photosynthesis. Permanent vacuole Contains cell sap Found within the cytoplasm Improves cell’s rigidity Cell wall (also present in algal cells) Made from cellulose Provides strength to the cell Bacterial cells are prokaryotic, so do not share as many similarities in the type of organelles as animal and plant cells do. https://bit.ly/pmt-cc https://bit.ly/pmt-edu https://bit.ly/pmt-cc In bacterial cells... Structure Function Cytoplasm Above Cell membrane Above Cell wall Made of a different compound (peptidogylcan) Single circular strand of DNA As they have no nucleus, this floats in the cytoplasm Plasmids Small rings of DNA To be able to calculate the size or area of sub-cellular structures, you should find a shape such as a circle or rectangle that resembles it. The rules that would normally be used to calculate the size/area of that shape (e.g length x width for a rectangle) should be applied to it. Cell Specialisation (1.1.3) Cells specialise by undergoing differentiation: a process that involves the cell gaining new sub-cellular structures in order for it to be suited to its role. Cells can either differentiate once early on or have the ability to differentiate their whole life (these are called stem cells). In animals, most cells only differentiate once, but in plants many cells retain the ability. Examples of specialised cells in animals 1. Sperm cells: specialised to carry the male’s DNA to the egg cell (ovum) for successful reproduction Streamlined head and long tail to aid swimming Many mitochondria (where respiration happens) which supply the energy to allow the cell to move The acrosome (top of the head) has digestive enzymes which break down the outer layers of membrane of the egg cell 2. Nerve cells: specialised to transmit electrical signals quickly from one place in the body to another The axon is long, enabling the impulses to be carried along long distances Having lots of extensions from the cell body (called dendrites) means branched connections can form with other nerve cells The nerve endings have many mitochondria which supply the energy to make special transmitter chemicals called neurotransmitters. These allow the impulse to be passed from one cell to another. https://bit.ly/pmt-cc https://bit.ly/pmt-edu https://bit.ly/pmt-cc 3. Muscle cells: specialised to contract quickly to move bones (striated muscle) or simply to squeeze (smooth muscle, e.g found in blood vessels so blood pressure can be varied), therefore causing movement Special proteins (myosin and actin) slide over each other, causing the muscle to contract Lots of mitochondria to provide energy from respiration for contraction They can store a chemical called glycogen that is used in respiration by mitochondria Examples of specialised cells in plants 1. Root hair cells: specialised to take up water by osmosis and mineral ions by active transport from the soil as they are found in the tips of roots Have a large surface area due to root hairs, meaning more water can move in The large permanent vacuole affects the speed of movement of water from the soil to the cell Mitochondria to provide energy from respiration for the active transport of mineral ions into the root hair cell 2. Xylem cells: specialised to transport water and mineral ions up the plant from the roots to the shoots Upon formation, a chemical called lignin is deposited which causes the cells to die. They become hollow and are joined end-to-end to form a continuous tube so water and mineral ions can move through Lignin is deposited in spirals which helps the cells withstand the pressure from the movement of water 3. Phloem cells: specialised to carry the products of photosynthesis (food) to all parts of the plants Cell walls of each cell form structures called sieve plates when they break down, allowing the movement of substances from cell to cell Despite losing many sub-cellular structures, the energy these cells need to be alive is supplied by the mitochondria of the companion cells. Cell Differentiation (1.1.4) To become specialised and be suited to its role, stem cells must undergo differentiation to form specialised cells. This involves some of their genes being switched on or off to produce different proteins, allowing the cell to acquire different sub-cellular substances for it to carry out a specific function. In animals, almost all cells differentiate at an early stage and then lose this ability. Most specialised cells can make more of the same cell by undergoing mitosis (the process that involves a cell dividing to produce 2 identical cells). Others such as red blood cells (which https://bit.ly/pmt-cc https://bit.ly/pmt-edu https://bit.ly/pmt-cc lose their nucleus) cannot divide and are replaced by adult stem cells (which retain their ability to undergo differentiation). In mature animals, cell division mostly only happens to repair or replace damaged cells, as they undergo little growth. In plants, many types of cells retain the ability to differentiate throughout life. They only differentiate when they reach their final position in the plant, but they can still re-differentiate when it is moved to another position. Microscopy (1.1.5) Extremely small structures such as cells cannot be seen without microscopes, which enlarge the image. The first cells of a cork were observed by Robert Hooke in 1665 using a light microscope. It has two lenses, an objective and eyepiece The objective lense produces a magnified image, which is then magnified and directed into the eye by the eyepiece lense It is usually illuminated from underneath They have, approximately, a maximum magnification of x2000 and a resolving power (this affects resolution: the ability to distinguish between two points) of 200nm (the lower the RP, the more detail is seen) Used to view tissues, cells and large sub-cellular structures In the 1930s the electron microscope was developed, enabling scientists to view deep inside sub-cellular structures, such as mitochondria, ribosomes, chloroplasts and plasmids. Electrons, as opposed to light, are used to form an image because the electrons have a much smaller wavelength than that of light waves There are two types: a scanning electron microscope that create 3D images (at a slightly lower magnification) and a transmission electron microscope which creates 2D images detailing organelles They have a magnification of up to x2,000,000 and resolving power of 10nm (SEM) and 0.2nm (TEM) Common calculations: 1. Magnification of a light microscope: magnification of the eyepiece lens x magnification of the objective lens 2. Size of an object: size of image/magnification = size of object (this formula can be rearranged to obtain the other values, make sure you are in the same units!) https://bit.ly/pmt-cc https://bit.ly/pmt-edu https://bit.ly/pmt-cc When working with calculations in microscopy, it is common to come across very large or small numbers. Standard form can be useful when working with these numbers. Through multiplying a certain number by a power of 10, it can get bigger or smaller. To be able to compare the size of numbers while using standard form, the ‘number’ which being multiplied by a power of 10 needs to be between 1 and 10. Examples: 1.5 x 10-5 = 0.000015 3.4 x 103 = 3400 Culturing Microorganisms (1.1.6 - Biology Only) Microorganisms are very small, so in order for scientists to study them they need to grow many of them in the lab using nutrients (culturing them). The culture medium contains carbohydrates for energy, minerals, proteins and vitamins. There are two ways to grow microorganisms in the lab: 1. In nutrient broth solution- involves making a suspension of bacteria to be grown and mixing with sterile nutrient broth (the culture medium), stoppering the flask with cotton wool to prevent air from contaminating it and shaking regularly to provide oxygen for the growing bacteria. 2. On an agar gel plate- the agar acts as the culture medium, and bacteria grown on it form colonies on the surface. Making the plate: Hot sterilised agar jelly is poured into a sterilised Petri dish, which is left to cool and set Wire loops called inoculating loops are dipped in a solution of the microorganism and spread over the agar evenly A lid is taped on and the plate is incubated for a few days so the microorganisms can grow (stored upside down) The reasons why we follow certain steps in this procedure need to be understood. Step Why? Petri dishes and culture media If this step does not take place, they are likely to must be sterilised before use, often be contaminated with other microorganisms. done by an autoclave (an oven) or These could be harmless but will compete with the UV light. desired bacteria for nutrients and space, or they could be harmful (for example through a mutation taking place), potentially producing a new https://bit.ly/pmt-cc https://bit.ly/pmt-edu https://bit.ly/pmt-cc pathogen. Inoculating loops must be sterilised This kills unwanted microorganisms, which is by passing them through a flame. needed for reasons above. The lid of the Petri dish should be Sealing stops airborne microorganisms from sealed (but not completely) with contaminating the culture, but it should not be tape. sealed all the way around as this would result in harmful anaerobic bacteria growing (due to no oxygen entering). The Petri dish should be stored This is to prevent condensation from the lid landing upside down. on the agar surface and disrupting growth. The culture should be incubated at If it were incubated at a higher temperature, nearer 25 degrees. 37 degrees (human body temperature), it would be more likely that bacteria that could be harmful to humans would be able to grow as this is their optimum temperature. At lower temperatures, colonies of such bacteria would not be able to grow. If they have a supply of nutrients and a suitable temperature, bacteria can multiply by binary fission (one splitting into two) as fast as every 20 minutes. You can calculate the number of bacteria in a population after a certain time if given the mean division time. The formula is: bacteria at beginning x 2 number of divisions = bacteria at end To calculate the number of divisions, divide the time the population is left for by the mean division time for that bacteria The number of bacteria at the end of the growth period can be very large, so it is common for it to be left in standard form (see above in ‘microscopy’ for more on standard form) *Bold = Higher tier* If the microorganisms are bacteria, they can be used to test the effects that different antibiotics (or disinfectants) have on their growth. The investigation involves soaking paper discs in different antibiotics, which are placed on an agar plate with bacteria. After leaving the plate, the size of the clear area around the discs shows how many bacteria have died, indicating therefore how effective the antibiotic is. 1. Soak the paper discs in different types/concentrations of antibiotics and place on an agar plate evenly spread with bacteria. One disc should be a control, soaked in sterile water. There should be no death of bacteria with this disc- showing only the type of antibiotic affects the size of the inhibition zone (the clear area left when they die). 2. If the bacteria are resistant to the antibiotic they will not die, but non-resistant will die, leaving an inhibition zone. https://bit.ly/pmt-cc https://bit.ly/pmt-edu https://bit.ly/pmt-cc 3. Leave the plate at 25 degrees for 2 days. 4. The zone of inhibition can be measured- the bigger it is, the more bacteria are killed and therefore the more effective the antibiotic is. In both investigations- growing bacteria and testing the effectiveness of antibiotics- you need to calculate cross-sectional areas (of colonies or inhibition zones). This involves using the formula πr², where r is the radius of the circle. Cell Division (1.2) Chromosomes (1.2.1) The nucleus contains your genetic information. This is found in the form of chromosomes, which contain coils of DNA. A gene is a short section of DNA that codes for a protein and as a result controls a characteristic- therefore each chromosome carries many genes. There are 23 pairs of chromosomes in each cell of the body, as you inherit one from your mother and one from your father - resulting in 46 chromosomes in total in each cell. Sex cells (gametes) are the exception: there are half the number of chromosomes, resulting in 23 chromosomes in total in each gamete cell. Mitosis and the Cell Cycle (1.2.2) The cell cycle is a series of steps that the cell has to undergo in order to divide. Mitosis is a step in this cycle- the stage when the cell divides. Stage 1 (Interphase): In this stage the cell grows, organelles (such as ribosome and mitochondria) grow and increase in number, the synthesis of proteins occurs, DNA is replicated (forming the characteristic ‘X’ shape) and energy stores are increased Stage 2 (Mitosis): The chromosomes line up at the equator of the cell and cell fibres pull each chromosome of the ‘X’ to either side of the cell. Stage 3 (Cytokinesis): Two identical daughter cells form when the cytoplasm and cell membranes divide Cell division by mitosis in multicellular organisms is important in their growth and development, and when replacing damaged cells. Mitosis is also a vital part of asexual reproduction, as this type of reproduction only involves one organism, so to produce offspring it simply replicates its own cells. https://bit.ly/pmt-cc https://bit.ly/pmt-edu https://bit.ly/pmt-cc Stem Cells (1.2.3) A stem cell is an undifferentiated cell which can undergo division to produce many more similar cells, of which some will differentiate to have different functions. Types of stem cells 1. Embryonic stem cells Form when an egg and sperm cell fuse to form a zygote They can differentiate into any type of cell in the body Scientists can clone these cells (though culturing them) and direct them to differentiate into almost any cell in the body These could potentially be used to replace insulin-producing cells in those suffering from diabetes, new neural cells for diseases such as Alzheimer’s, or nerve cells for those paralysed with spinal cord injuries 2. Adult stem cells If found in bone marrow they can form many types of cells including blood cells 3. Meristems in plants Found in root and shoot tips They can differentiate into any type of plant, and have this ability throughout the life of the plant They can be used to make clones of the plant- this may be necessary if the parent plant has certain desirable features (such as disease resistance), for research or to save a rare plant from extinction Therapeutic cloning involves an embryo being produced with the same genes as the patient. The embryo produced could then be harvested to obtain the embryonic stem cells. These could be grown into any cells the patient needed, such as new tissues or organs. The advantage is that they would not be rejected as they would have the exact same genetic make-up as the individual. Benefits vs problems of research with stem cells Benefits Problems Can be used to replace damaged or We do not completely understand the diseased body parts. process of differentiation, so it is hard to control stem cells to form the cells we desire. Unwanted embryos from fertility clinics Removal of stem cells results in destruction could be used as they would otherwise of the embryo. https://bit.ly/pmt-cc https://bit.ly/pmt-edu https://bit.ly/pmt-cc be discarded. Research into the process of People may have religious or ethical differentiation. objections as it is seen as interference with the natural process of reproduction. If the growing stem cells are contaminated with a virus, an infection can be transferred to the individual. Money and time could be better spent into other areas of medicine. Transport in Cells (1.3) Diffusion (1.3.1) The definition of diffusion in the specification is ‘the spreading out of the particles of any substance in solution, or particles of a gas, resulting in a net movement from an area of higher concentration to an area of lower concentration’. When many particles are close together they collide with each other more often, resulting in them moving around and becoming mixed with the other particles in the area. It is passive as no energy is required. Substances can move over cell membranes via diffusion, into and out of cells. The molecules have to be small in order to be able to move across- for example oxygen, glucose, amino acids and water, but starch and proteins cannot. Examples of where this takes place in the body: Oxygen moves through the membranes of structures in the lung called alveoli into the red blood cells, and is carried to cells across the body for respiration. Carbon dioxide (the waste product of respiration) moves from the red blood cells into the lungs to be exhaled. These movements of gases is called gas exchange. Urea (a waste product) moves from the liver cells into the blood plasma to be transported to the kidney for excretion Many factors affect the rate of diffusion: Factor Effect Concentration gradient (difference in The greater the difference in concentration, concentrations) the faster the rate of diffusion. This is because more particles are randomly moving down the gradient than are moving against it. https://bit.ly/pmt-cc https://bit.ly/pmt-edu https://bit.ly/pmt-cc Temperature The greater the temperature, the greater the movement of particles, resulting in more collisions and therefore a faster rate of diffusion. Surface area of the membrane The greater the surface area, the more space for particles to move through, resulting in a faster rate of diffusion. Surface area to volume ratio- the size of the surface area of the organism compared to its volume Calculated by finding the volume (length x width x height) and the surface area (length x width), and writing the ratio in the smallest whole numbers If this is large, the organism is less likely to require specialised exchange surfaces and a transport system because the rate of diffusion is sufficient in supplying and removing the necessary gases E.g 15 (surface area): 5 (volume) is written as 3:1 Single-celled organisms can use diffusion to transport molecules into their body from the air- this is because they have a relatively large surface area to volume ratio. Due to their low metabolic demands, diffusion across the surface of the organism is sufficient enough to meet its needs. In multicellular organisms the surface area to volume ratio is small so they cannot rely on diffusion alone. Instead, surfaces and organ systems have a number of adaptations that allows molecules to be transported in and out of cells. Examples: In the lungs, oxygen is transferred to the blood and carbon dioxide is transferred to the lungs. This takes place across the surface of millions of air sacs called alveoli, which are covered in tiny capillaries, which supply the blood. In the small intestine, cells have projections called villi. Digested food is absorbed over the membrane of these cells, into the bloodstream. The gills are where gas exchange takes place in fish. Water which has oxygen passes through the mouth and over the gills. Each gill has plates called gill filaments, and upon these are gill lamellae, which is where diffusion of oxygen into the blood and diffusion of carbon dioxide into the water takes place. Blood flows in one direction while water flows in the other. https://bit.ly/pmt-cc https://bit.ly/pmt-edu https://bit.ly/pmt-cc The roots of plants are adapted to take up water and mineral ions. Roots have root hair cells with large surface areas, which project into the soil. In the leaves of the plant there are many different tissues to aid with gas exchange. Carbon dioxide diffuses through stomata for photosynthesis, whilst oxygen and water vapour move out through them. The stomata are controlled by guard cells, which change the size of the stomata based on how much water the plant received (the guard cells swell with lots of water and make the stomata larger) Adaptation Why? Example Having a large The greater the Lungs: the small, spherical alveoli (sites of surface area surface area, the more gaseous exchange) in the lungs create a very particles can move large surface area (approximately 75m2 in through, resulting in a humans). faster rate of diffusion Small intestine: the cells of the small intestine have millions of villi, which are projections which increase the surface area. This means digested food can be absorbed into the blood faster Fish gills: these contain lamellae to increase the surface area. Leaves: the flattened shape increases the surface area. The air spaces inside the leaf increase the surface area, so more carbon dioxide can enter cells. Having a thin Provides a short Lungs: alveoli and capillary walls are membrane diffusion pathway, extremely thin. allowing the process to occur faster Small intestine: villi have a single layer of surface cell. Having an Creates a steep Lungs: the lungs constantly supply oxygen to efficient blood concentration make the blood from alveoli capillaries supply/being gradient, so diffusion oxygenated, by exchanging it for carbon ventilated (in occurs faster dioxide that can be breathed out. This is a animals) constant process meaning the concentration gradient is always steep. Fish: water flows in one direction and blood flows in the other - this means that a steep concentration gradient is maintained as the concentration of oxygen is always much https://bit.ly/pmt-cc https://bit.ly/pmt-edu https://bit.ly/pmt-cc higher in the water - so it will diffuse across. Osmosis (1.3.2) Water is able to move across cell membranes by osmosis- the movement of water from a less concentrated solution to a more concentrated one through a partially permeable membrane. A dilute solution of sugar has a high concentration of water (and therefore a high water potential). A concentrated solution of sugar has a low concentration of water (and therefore a low water potential). Water moves from a dilute solution to a concentrated solution because it moves from an area of high water potential to low water potential- down the concentration gradient. It is passive (does not use energy). The cytoplasm of a cell contains salts and sugars, so therefore when a cell is placed in a dilute solution, water will move in. This situation can be modelled with a partially permeable membrane bag containing sugar molecules, with a glass tube placed in it with the top out of the water This can be placed in solutions of varying concentrations in order to observe the movement of water in and out by looking at the level of the water in the tube ○ If the concentration of sugar in external solution is the same as the internal, there will be no movement and the solution is said to be isotonic to the cell ○ If the concentration of sugar in external solution is higher than the internal, water moves out, and the solution is said to be hypertonic to the cell ○ If the concentration of sugar in external solution is lower than the internal, water moves in, and the solution is said to be hypotonic to the cell Osmosis in animals: If the external solution is more dilute (higher water potential), it will move into animal cells causing them to burst. On the other hand, if the external solution is more concentrated (lower water potential), excess water will leave the cell causing it to become shrivelled. Osmosis in plants: If the external solution is more dilute, water will move into the cell and into the vacuole, causing it to swell, resulting in pressure called turgor (essential in keeping the leaves and stems of plants rigid). If the external solution is less dilute, water will move out of the cell and they will become soft. Eventually the cell membrane will move away from the cell wall (called plasmolysis) and it will die. https://bit.ly/pmt-cc https://bit.ly/pmt-edu https://bit.ly/pmt-cc Experiment: The effect of sugar solutions on plant tissue Placing potato tubers (cylinders) in different concentrations of sugar solution results in different volumes of water moving in or out of the tubers. This affects its mass. By measuring the mass of the tuber before and after placing it in solution we can see whether the concentration of external solution or potato was higher, depending on whether it has got heavier or lighter. From this we can calculate the percentage change in mass (by dividing the change by the original mass) and plot on a graph. Active Transport (1.3.3) Active transport is the movement of particles from an area where they are in lower concentration to an area where they are in higher concentration- against their concentration gradient. This is not passive as diffusion is, but requires energy from respiration, which is why it is called active. In root hairs: They take up water and mineral ions (for healthy growth) from the soil Mineral ions are usually in higher concentrations in the cells, meaning diffusion cannot take place This requires energy from respiration to work In the gut: Substances such as glucose and amino acids from your food have to move from your gut into your bloodstream Sometimes there can be a lower concentration of sugar molecules in the gut than the blood, meaning diffusion cannot take place Active transport is required to move the sugar to the blood against its concentration gradient https://bit.ly/pmt-cc https://bit.ly/pmt-edu https://bit.ly/pmt-cc