Periodontal Microbiology and Dental Plaque PDF
Document Details
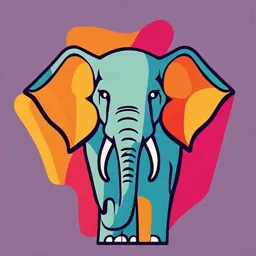
Uploaded by RichTourmaline9881
Yakın Doğu Üniversitesi Dişhekimliği Fakültesi
AYŞE ÇAYGÜR YORAN
Tags
Summary
This document provides an overview of periodontal microbiology and dental plaque, including learning outcomes, colonization processes, and the role of pathogens. It discusses the different types of bacteria involved and their importance in periodontal health and disease.
Full Transcript
Assist. Prof. Dr. AYŞE ÇAYGÜR YORAN YAKINDOĞU ÜNİVERSİTESİ DİŞHEKİMLİĞİ FAKÜLTESİ Learning outcomes: 1-Will be able to explain oral microflora in periodontal health and periodontal diseases. 2- Will be able to define dental plaque, define dental plaque Hypothesis in line with periodontal pathogene...
Assist. Prof. Dr. AYŞE ÇAYGÜR YORAN YAKINDOĞU ÜNİVERSİTESİ DİŞHEKİMLİĞİ FAKÜLTESİ Learning outcomes: 1-Will be able to explain oral microflora in periodontal health and periodontal diseases. 2- Will be able to define dental plaque, define dental plaque Hypothesis in line with periodontal pathogenesis. 3- Will be able to clinically distinguish materia alba, dental plaque and other attachments accumulated on the tooth surface. 4- Will be able to explain the content of dental plaque, explain the The process of plaque 5- Will be able to define periodontal pathogens and explain the virulence factors of pathogens causing periodontal diseases. 6- Will be able to explain the factors in transition from periodontal health to disease. Periodontal Microbiology and Dental Plaque The colonization of the oral cavity also starts close to the time of birth. Within hours after birth, the sterile oral cavity will be colonized by low numbers of mainly facultative and aerobic bacteria. At that time, the oral microbiota of newborns closely resembles the mother’s vaginal microbiota or, for newborns delivered by cesarean section, the mother’s skin microbi- ota. From the second day, anaerobic bacteria can be detected in the infant’s edentulous mouth. The number of oral bacteria increases gradually as a result of exposure to external environmental microbial sources.Streptococcus salivarius and Streptococcus mitis have been identified as the first and most dominant oral microbes to colonize the oral cavity of newborn infants.Veillonella spp. , Neisseria spp., Actinomyces spp. , and Staphylococcus spp. are also among the first colonizers of the oral cavity. After tooth eruption, a more complex oral microbiota is established. The species that colonize the teeth after eruption include Streptococcus sanguinis, Lactobacillus spp., and Streptococcus oralis. Oral streptococci, including S. oralis, Streptococcus anginosus, mutans streptococci (Streptococcus mutans and Streptococcus sobrinus), and Streptococcus gordonii are commonly reported to be present after the first year of life. In addition, anaerobes, including Fusobacterium spp. and Prevotella spp. (Figure 8-1, H and I), can alsobe detected in young children. In later childhood, the bacterial diversity and numbers in the oral cavity increase as more teeth erupt and provide more areas for the adherence and retention of bacteria. Because of the paucity of longitudinal studies, relatively little is known about the initial colonization of key microbes found in the oral cavity of children and adults.- It is estimated that more than 500 different species are capable of colonizing the adult mouth and that any individual typically harbors around 50 to 150 different species When one thinks about bacteria, one almost immediately associates them with different pathologies. However, most oral bacteria are harmless commensals under normal circumstances. This means that this microbiota lives in harmony with its host but that, under specific conditions (i.e., increased mass and/or pathogenicity, suppression of commensal or beneficial bacteria, and/or reduced host response), disease can occur. The importance of the commensal microbiota is clearly illustrated by the development of yeast infections when the normal oral microbiota is reduced, such as after a longer period of systemic antibiotic usage. In addition, it has been shown that aggressive periodontitis is associated with a loss of colonization of S. sanguinis. On the other hand, it was recently shown in mice that the commensal microbiota is required for P. gingivalis induced bone loss. The Oral Cavity From a Microbe’s Perspective With the exception of those microorganisms that are present in feces and in secretory fluids, all bacteria maintain themselves within their host by adhering to a surface. This principle also applies to the oral cavity. From an ecologic viewpoint, the oral cavity, which communicates with the pharynx, should be considered as an “open growth system” with an uninterrupted ingestion and removal of microorganisms and their nutrients. A dynamic equilibrium exists between the adhesion forces of microorganisms and a variety of removal forces that originate from the following sources: (1) swallowing, mastication, or blowing the nose; (2) tongue and oral hygiene implements; (3) the wash-out effect of the salivary, nasal, and crevicular fluid outflow; and (4) the active motion of the cilia of the nasal and sinus walls. Most organisms can only survive in the oropharynx when they adhere to either the soft tissues or the hard surfaces (i.e., teeth, restorative or prosthetic materials, and implants). The ability of a bacterium to adhere to its host is crucial for the induction of infectious diseases, such as gingivitis or periodontitis. Oral bacteria and especially pathogenic bacteria, such as Porphyromonas gingivalis and Aggregatibacter actinomycetemcomitans, have a large battery of virulence factors, one of which is the ability to adhere to hard intraoral surfaces and/or to the oral mucosae On the basis of physical and morphologic criteria, the oral cavity can be divided into six major ecosystems (also called niches), each with the following distinct ecologic determinants: • The intraoral and supragingival hard surfaces (teeth, implants, restorations, and prostheses) • Subgingival regions adjacent to a hard surface, including the periodontal/periimplant pocket (characterized by the presence of crevicular fluid, the root cementum or implant surface, and the pocket epithelium) • The buccal palatal epithelium and the epithelium of the floor of the mouth • The dorsum of the tongue • The tonsils • The saliva In health, there is a core set of microorganisms that are almost universally present in these ecosystems. This core microbiome includes members of the phyla Firmicutes (Streptococcus spp., Veillonella spp., and Granulicatella spp.), Proteobacteria (Neisseria spp., Campylobacter spp., and Haemophilus spp.), Actinobacteria (Corynebacterium spp., Rothia spp., and Actinomyces spp.), Bacteroidetes (Prevotella spp., Capnocytophaga spp., and Porphyromonas spp.) and Fusobacteria (Fusobacterium spp.).In periodontal pockets, studies have shown high numbers of bacteria attached to pocket epithelial cells in vivo. Areas of gingival inflammation are characterized by an increased number of adhering bacteria. These adhering bacteria can also infiltrate the pocket wall in relatively large numbers and reach the underlying stroma . In general, there is a positive correlation between the adhesion rate of pathogenic bacteria todifferent epithelia and the susceptibility of the affected patient to certain infections. In the periodontal pocket, different strategies contribute to bacterial survival, such as adhesion to the pocket epithelium and, when dentine is encountered, the colonization of the dentine tubules.The crevicular fluid with its constant outflow does not favor the maintenance of unattached bacteria in the periodontal pocket. It has been suggested that teeth are the primary habitat for periodontopathogens, because soon after a full-mouth tooth extraction in patients with severe periodontitis, key pathogens such as A. actinomycetemcomitans and P. gingivalis disappeared from the oral cavity as determined by bacterial culturing techniques.Prevotella intermedia and other black-pigmented Prevotella spp. did remain, but at lower detection frequencies and numbers However, studies involving the use of molecular tools to detect and quantify oral bacteria seem to indicate that A. actinomycetemcomitans and P. gingivalis are not entirely eradicated after full-mouth extraction. They may remain colonizers of the oral cavity,but, when teeth are lost, their relative numbers decrease. Bacteria and Their Biofilm Mode of Living To many people, the word microbiologist conjures images of someone in a laboratory coat swilling a flask of bacterial cells that are growing happily as pure cultures in nutrient-rich broth. In nature, bacteria rarely enjoy such an easy life. The major struggle faced by bacteria lies in obtaining sufficient nutrients to support growth. Competition is rife, and most microbial communities contain many different species, sometimes 100 or more, sharing the same site. Nutrients tend to concentrate at interfaces, and consequently the densest bacterial populations are located at interfaces of various kinds. In aquatic ecosystems, the major interfaces are the solid–liquid boundaries, such as the surfaces of submerged rocks or soil particles, and the air–water interface that is present in open systems, such as oceans or lakes. Bacteria grow well in the laboratory at the solid–gas interface on the surface of an agar plate. However, desiccation rapidly kills most bacterial cells, and microbial growth at solid–gas interfaces is therefore generally restricted to areas where moisture is available. The human body provides several interfaces that support microbial populations, of which the most important in healthy individuals are the skin, gut, mouth, and female urogenital tract. As mentioned previously, the oral cavity is somewhat unique in this regard, because it provides hard,nonshedding surfaces (teeth) that are accessible for microbial colonization. Biofilm bacteria are often up to 1000 times more resistant to antimicrobial agents than their planktonic counterparts. Bacteria that grow in multispecies biofilms interact closely with neighboring cells. Sometimes these interactions are mutually beneficial, as is the case when one organism removes another’s waste products and- uses them as an energy source. In other instances, bacteria compete with their neighbors by secreting antibacterial molecules such as inhibitory peptides (bacteriocins) or hydrogen peroxide (H2O2). In addition, the biofilm mode of growth facilitates cell–cell signaling and deoxyribonucleic acid (DNA) exchange between bacteria. It is clear that microbial ecology within biofilm communities is highly complex and that, in many cases, knowledge is only emerging at this point. Microbial populations on the surfaces of teeth (dental plaque) are excellent examples of biofilm communities . The architecture of a dental plaque biofilm has many features in common with other biofilms. It is heterogeneous in structure, with clear evidence of open fluid-filled channels running through the plaque mass. Nutrients make contact with the sessile (attached) microcolonies by diffusion from the water channels to the microcolony rather than from the matrix. The bacteria exist and proliferate within the intercellular matrix through which the channels run. The matrix confers a specialized environment that distinguishes the bacteria that exist within the biofilm from those that are free-floating; this is the so-called “planktonic state” insolutions such as saliva or crevicular fluid. The biofilm matrix functions as a barrier. Substances produced by bacteria within the biofilm are retained and concentrated, which fosters metabolicinteractions among different bacteria. The intercellular matrix consists of organic and inorganic mate- rials derived from saliva, gingival crevicular fluid, and bacterial products. The inorganic components of plaque are predominantly calcium and phosphorus, with trace amounts of other minerals such as sodium, potassium, and fluoride. The source of inorganic constituents of supragingival plaque is primarily saliva. As the mineral content increases, the plaque mass becomes calcified to form calculus. Calculus is frequently found in areas of thedentition adjacent to salivary ducts (e.g., the lingual surface of the mandibular incisors and canines, the buccal surface of the maxillary first molars), which reflects the high concentration of minerals available from saliva in those regions. The inorganic components of subgingival plaque are derived from crevicular fluid (a serum transudate). The calcification of subgingival plaque also results in calculus formation (Figure 8-9). Subgingival calculus is typically dark green or dark brown, which probably reflects the presence of blood products that are associated with subgingival hemorrhage. The importance of these biofilms for oral diseases, such as dental caries and periodontitis—together with the relative ease with which tooth surface biofilms can be accessed—has led to dental plaque becoming one of the most highly studied biofilm systems. It is anticipated that, by understanding the mechanisms involved in the accumulation of dental plaque and the transition from health to disease, it will be possible to improve our control over these processes and to further restrict plaque-associated oral diseases. Structure of a Mature Dental Plaque Biofilm Dental plaque is defined clinically as a structured, resilient, yellow-grayish substance that adheres tenaciously to the intraoral hard surfaces, including removable and fixed restorations The tough extracellular matrix makes it impossible to remove plaque by rinsing or with the use of sprays. Plaque can thus be differentiated from other deposits that may be found on the tooth surface, such as materia alba and calculus. Materia alba refers to soft accumulations of bacteria, food matter, and tissue cells that lack the organized structure of dental plaque and that are easily displaced with a water spray. Calculus is a hard deposit that forms via the mineralization of dental plaque and that is generally covered by a layer of unmineralized plaque. Dental plaque is composed primarily of microorganisms. One gram of plaque (wet weight) contains approximatelybacteria.The number of bacteria in supragingival plaque on asingle tooth surface can exceed cells. In a periodontal pocket, counts can range from bacteria in a healthy crevice to more than bacteria in a deep pocket. With the use of highly sensitive molecular techniques for microbial identification, it has been estimated that more than 500 distinct microbial phylotypes can be present as natural inhabitants of dental plaque.Any individual may harbor 150 or more different species. Non-bacterial microorganisms that are found in plaque include archaea,yeasts, protozoa, and viruses.Dental plaque is broadly classified as supragingival or subgingival on the basis of its position on the tooth surface toward the gingival margin. • Supragingival plaque is found at or above the gingival margin; when in direct contact with the gingival margin, it is referred to as marginal plaque. • Subgingival plaque is found below the gingival margin, between the tooth and the gingival pocket epithelium. Supragingival plaque typically demonstrates the stratified organization of a multilayered accumulation of bacterial morphotypes. Gram-positive cocci and short rods predominate at the tooth surface, whereas gram-negative rods and filaments as well as spirochetes predominate in the outer surface of the mature plaque mass. In general, the subgingival microbiota differs in composition from the supragingival plaque, primarily because of the local avail- ability of blood products and a low reduction–oxidation (redox) potential, which characterizes the anaerobic environment. Many periodontopathogens are fastidious strict anaerobes and as such may contribute little to the initiation of disease in shallow gingival pockets. In deep periodontal pockets, however, they find their preferred habitat. The identification of bacteria within intact dental plaque repre- sents a significant challenge. Most of the previously cited studies of dental plaque microbiota used techniques that involved the disruption of the dental plaque matrix followed by microbial culture or culture-independent identification. Techniques have been developed that allow for the specific visualization of individual bacteria within mixed populations. Many strains of Actinomyces coaggregate with oral streptococci, and therefore the spatial organization of these organisms within dental plaque may be influenced by adhesin–receptor interactions. In fact, a protein adhesin of A. naeslundii has been shown to colocalize with a cognate oral streptococcal receptor polysaccharide in in situ biofilms using specific antibody (immunofluorescence) labeling. These studies clearly demonstrate that the interactions identified among bacteria isolated in the laboratory are relevant to the dental plaque biofilm. TABLE :Differences Between Tooth Deposits Materia Alba Dental Plaque Calculus White cheeselike accumulation Resilient clear to yellow-grayish Hard deposit that forms via substance the mineralization of dental plaque Soft accumulation of salivary proteins, some bacteria, many desquamated epithelial cells, and occasional disintegrating food debris Primarily composed of bacteria in a matrix of salivary glycoproteins and extracellular polysaccharides Lacks an organized structure and is therefore not as complex as dental plaque Considered to be a biofilm Easily displaced with a water spray Impossible to remove by rinsing or with Generally covered by a layer of unmineralized dental plaque the use of sprays The environmental parameters of the subgingival region differ from those of the supragingival region. The gingival crevice or pocket is bathed by the flow of crevicular fluid, which contains many substances that bacteria may use as nutrients. Host inflammatory cells and mediators are likely to have considerable influence on the establishment and growth of bacteria in the subgingival region. Both morphologic and microbiologic studies of subgingival plaque reveal distinctions between the tooth-associated regions and the soft-tissue–associated regions of subgingival plaque.The tooth-associated cervical plaque that adheres to the root cementum does not markedly differ from that observed in gingivitis. At this location, filamentous microorganisms dominate, but cocci and rods also occur. This plaque is dominated by grampositive rods and cocci, including S. mitis, S. sanguinis, Actinomyces oris, A. naeslundii, and Eubacterium spp. However, in the deeper parts of the pocket, the filamentous organisms become fewer in number; in the apical portion, they seem to be virtually absent. Instead, the microbiota is dominated by smaller organisms without a particular orientation. The apical border of the plaque mass is separated from the junctional epithelium by a layer of host leukocytes, and the bacterial population of this apicaltooth–associated region shows an increased concentration of gram negative rods The layers of microorganisms that face the soft tissue lack a definite intermicrobial matrix and contain primarily gram-negative rods and cocci as well as large numbers of filaments, flagellated rods, and spirochetes. Studies of plaque associated with crevicular epithelial cells indicate a predominance of species such as S. oralis, S. intermedius, Parvimonas micra (formerly Micromonas micra and Peptostreptococcus micros), P. gingivalis, P. intermedia, T. forsythia, and F. nucleatum. Host-tissue cells (e.g., white blood cells, epithelial cells) may also be found in this region.Bacteria are also found within the host tissues, such as in the soft tissues , and within epithelial cells as well as in the dentinal tubules The composition of the subgingival plaque depends on the pocket depth. The apical part is more dominated by spirochetes, cocci, and rods, whereas in the coronal part more filaments are observed. The site specificity of plaque is significantly associated with diseases of the periodontium. Marginal plaque, for example, is of prime importance during the initiation and development of gingivitis. Supragingival plaque and tooth-associated subgingival plaque are critical in calculus formation and root caries, whereas tissue associated subgingival plaque is important in the tissue destruction that characterizes different forms of periodontitis. Biofilms also establish on artificial surfaces exposed to the oral environment, such as prostheses and implants. A large series of articles compared the microbiota in pockets around the teeth with those around implants of partially edentulous patients. The similarities were striking. Accumulation of a Dental Plaque Biofilm The process of plaque formation can be divided into several phases: (1) the formation of the pellicle on the tooth surface; (2) the initial adhesion/attachment of bacteria; and (3) colonization/plaque maturation. Formation of the Pellicle. All surfaces in the oral cavity, including the hard and soft tissues, are coated with a layer of organic material known as the acquired pellicle. The pellicle on tooth surfaces consists of more than peptides, proteins, and glycoproteins, including keratins, mucins, proline-rich proteins, phosphoproteins (e.g., statherin), histidine-rich proteins, and other molecules that can function as adhesion sites (receptors) for bacteria. The salivary pellicle can be detected on clean enamel surfaces within 1 minute after their introduction into the mouths of volunteers. By 2 hours, the pellicle is essentially in equilibrium between adsorption and detachment, although further pellicle maturation can be observed for several hours. Transmission electron microscopy shows the pellicle to be composed of two layers: a thin basal layer that is very difficult to remove, even with harsh chemical and mechanical treatments, and a thicker globular layer, up to 1 μm or more, that is easier to detach. From these observations, it can be concluded that dental enamel is permanently covered with an acquired pellicle from the moment that teeth erupt. Consequently, bacteria that adhere to tooth surfaces do not contact the enamel directly but interact with the acquired enamel pellicle. However, the pellicle is not merely a passive adhesion matrix. Many proteins retain enzymatic activity when they are incorporated into the pellicle, and some of these, such as peroxidases, lysozyme, and α-amylase, may affect the physiology and metabolism of adhering bacterial cells.In addition, a close ecological relationship between the pellicle and its associated microbiology seems to exist. Walker and cowork ers reported that dental plaque samples will only produce in vitro biofilms if the surface on which they were grown contained a salivary pellicle belonging to the patient who donated the plaque sample. No biofilm could be grown on a pellicle that come from a different subject. Initial Adhesion/Attachment of Bacteria. Toothbrushing removes most but not all bacteria from the exposed surfaces of teeth. However, recolonization begins immediately, and bacteria can be detected within 3 minutes of the introduction of sterile enamel into the mouth. Colonization and Plaque Maturation. The primary colonizing bacteria adhered to the tooth surface provide new receptors for attachment by other bacteria as part of a process known as “coadhesion.” Together with the growth of adherent microorganisms, coadhesion leads to the development of micro- colonies and eventually to a mature biofilm. Cell–cell adhesion between genetically distinct oral bacteria also occurs in the fluid phase (i.e., in saliva). In the laboratory, interactions between genetically distinct cells in suspension result in clumps or “coaggregates” that are macro scopically visible . Different species—or even different strains of a single species have distinct sets of coaggregation partners . Fusobacteria coaggregate with all other human oral bacteria, whereas Veillonella spp., Capnocytophaga spp. and Prevotella spp. bind with streptococci and/or actinomyces.Each newly accreted cell becomes itself a new surface and therefore may act as a coaggregation bridge to the next potentially accreting cell type that passes by. Phase 1: Transport to the Surface. The first stage involves the initial transport of the bacterium to the tooth surface. Random contacts may occur, for example, through Brownian motion (average displacement, 40 μm/hour), through sedimentation of microorganisms, through liquid flow (several orders of magnitude faster than diffusion), or through active bacterial movement (chemotactic activity). It should be noted, however, that relatively few oral bacteria are motile, and forces such as saliva flow or mechanical contact between oral soft tissues and teeth are almost certainly more important than swimming for bringing the primary colonizing bacteria into contact with teeth. Phase 2: Initial Adhesion. The second stage results in an initial reversible adhesion of the bacterium. This is initiated when the bacterial cell comes into close proximity to the surface (separation distance, ≈50 nm). Long- and short-range forces, including van der Waals attractive forces and electrostatic repulsive forces, operate at this distance. The behavior of bacterial cells can be reasonably described by the Derjaguin–Landau–Verwey–Overbeek theory of colloid stability.141 According to this theory, the total interaction energy (also called the total Gibbs energy [GTOT]), is the sum of the attractive forces (GA) and the electrostatic repulsion (GR). At the physiologic ionic strength of saliva, the van der Waals forces result in a net attraction of bacterial cells at distances of tens of nanometers from the surface. Approximately 10 nm from the surface, there is a secondary net energy minimum. Electrostatic repulsion prevents bacterial cells from getting even closer to the surface. At distances of approximately 10 nm from the surface, bacterial cells are reversibly bound. It is thought that stronger binding at this point is the consequence of interactions between bacterial adhesins and receptors in the salivary pellicle. It has been estimated that 10 to 50 ligand-receptor interactions are required to attain essentially irreversible binding of a bacterial cell to the pellicle Phase 3: Strong Attachment. After initial adhesion, a firm anchorage between the bacterium and the surface is established. On a rough surface, bacteria are more protected against shear forces so that a change from reversible to irreversible binding may occur more easily and more frequently. The substratum surface free energy becomes important, because the water film between the interacting surfaces has to be removed before short-range forces can be involved. The binding between the bacteria and the pellicle is mediated by specific adhesins on the bacterial cell surface(usually proteins) and complementary receptors (proteins, glycoproteins, or polysaccharides) in the acquired pellicle. Many proteins in the acquired pellicle can act as receptors for streptococci, including α-amylase, acid proline-rich proteins, statherin, and salivary agglutinin glycoprotein gp340.335 The specific adhesins of primary colonizing bacteria have been the subject of many investigations, because these represent potential targets for interference with the formation of dental plaque. One of the best-characterized interactions is the binding between the antigen I/II family adhesins of oral streptococci and gp340.159,160,285 Antigen I/II family adhesins are 160-kDa to 180-kDa proteins expressed on the surfaces of many oral streptococci, including S. mutans, S. sobrinus S. gordonii, and S. intermedius. Gp340 is present in fluid-phase saliva, and it is a component of the salivary pellicle. In the fluid phase, interactions between antigen I/II proteins and gp340 result in the aggregation of bacteria. Large clumps of bacterial cells do not stick well to surfaces, and they are probabl removed by swallowing. Coaggregation is a direct interaction; it is distinct from agglu- tination, which occurs when cells are stuck together by molecules in solution. At least 18 genera from the oral cavity have shown some form of coaggregation. All oral bacteria possess surface molecules that foster some sort of cell–cell interaction .The initial stages of coaggregation or coadhesion are essentially the same as the first steps involved in bacterial binding to surfaces: bacterial cells come into contact through passive or active transport and bind weakly through nonspecific hydrophobic, electrostatic, and van der Waals forces.These steps can be dramatically accelerated in vitro by vigorously mixing dense sus- pensions of bacterial cells. Strong cell–cell binding is then deter- mined by the presence of adhesin proteins or carbohydrates on one partner and complementary receptor proteins or carbohydrates on the other. Note that adhesin–receptor interactions are mediated by the fundamental physicochemical forces (i.e., hydrophobic, electrostatic, and van der Waals), but they are highly specific. stages of plaque formation, coaggregation between different gram negative species is likely to predominate. Examples of these types of interactions are the coaggregation of F. nucleatum with P. gingivalis or Treponema denticola Table: Diagrammatic representation of initial plaque formation. Early colonizers bind to receptors in the pellicle. Each adherent cell becomes in turn the nascent surface and bridge for additional species (secondary colonizers). The complementary sets of adhesin receptor symbols (example in box) represent the various kinds of coaggregations as well as the interactions with molecules in the pellicle. The symbol with a stem (adhesin) represents a cellular component that is heat inactivated (cell suspension heated to 85°C for 30 minutes) and sensitive to protease treatment. The cell type that exhibits the complementary symbol (receptor) is insensitive to either treatment. The symbols with a rectangular shape represent lactose-inhibitable coaggregations; the others are lactose-noninhibitable. Primary colonizers Streptococcus gordonii Streptococcus intermedius Streptococcus mitis Streptococcus oralis Streptococcus sanguinis Actinomyces gerencseriae Actinomyces israelii Actinomyces naeslundii Actinomyces oris Aggregatibacter actinomycetemcomitans serotype a Capnocytophaga gingivalis Capnocytophaga ochracea Capnocytophaga sputigena Eikenella corrodens Actinomyces odontolyticus Veillonella parvula Secondary colonizers Campylobacter gracilis Campylobacter rectus Campylobacter showae Eubacterium nodatum Aggregatibacter actinomycetemcomitans serotype b Fusobacterium nucleatum spp nucleatum Fusobacterium nucleatum spp vincentii Fusobacterium nucleatum spp polymorphum Fusobacterium periodonticum Parvimonas micra Prevotella intermedia Prevotella loescheii Prevotella nigrescens Streptococcus constellatus Tannerella forsythia Porphyromonas gingivalis Treponema denticola Table:Overview of Primary and Secondary Colonizers in Dental Plaque Factors That Affect Supragingival Dental Plaque Formation Clinically, early undisturbed plaque formation on teeth follows an exponential growth curve when measured planimetrically.During the first 24 hours when starting with a clean tooth surface, plaque growth is negligible from a clinical viewpoint (i.e., <3% coverage of the vestibular tooth surface, which is an amount nearly undetectable clinically). This “lag time” is a result of the fact that the microbial population must reach a certain size before it can easily be detected by a clinician. During the following 3 days, coverage progresses rapidly to the point at which, after 4 days, an average of 30% of the total coronal tooth area will be covered with plaque . Several reports have shown that the microbial composition of the dental plaque will change, with a shift toward a more anaerobic and a more gram-negative flora, including an influx of fusobacteria, filaments, spiral forms, and spirochetes.. This was clearly illustrated in experimental gingivitis studies. With this ecologic shift within the biofilm, there is a transition from the early aerobic environment, which is characterized by gram-positive facultative species, to a highly oxygen-deprived environment, in which gram-negative anaerobic microorganisms predominate. Bacterial growth in older plaque is much slower than in newly formed dental plaque, presumably because nutrients become limiting for much of the plaque biomass. Topography of Supragingival Plaque. Early plaque formation on teeth follows a typical topographic pattern), with initial growth along the gingival margin and from the interdental spaces (i.e., the areas protected from shear forces). Later, a further extension in the coronal direction can be observed. This pattern may change severely when the tooth surface contains irregularities that offer a favorable growth path. Plaque formation may also originate from grooves, The idea that coaggregation is important during the formation of oral biofilms opens new perspectives, especially for the use of probiotics. Special examples of coaggregations are the corncob formation, in which streptococci adhere to filaments of Corynebacterium matruchotii or Actinomyces spp., and the testtube brush formation, composed of filamentous bacteria to which gram-negative rods adhere. An analysis of more than 13,000 plaque samples that looked for 40 subgingival microorganisms using a DNA-hybridization methodology defined color-coded “complexes” of periodontal microorganisms that tend to be found together in health or disease. The composition of the different complexes was based on the frequency with which different clusters of microorganisms were recovered, and the complexes were color-coded for easy conceptualization (Figure 8-22).365 Interestingly, the early colonizers are either independent of defined complexes (A. naeslundii, A. oris) or members of the yellow (Streptococcus spp.) or purple complexes (A. odontolyticus) The microorganisms primarily considered secondary colonizers fell into the green, orange, and red complexes. The green complex includes Eikenella corrodens, A. actinomycetemcomitans serotype a, and Capnocytophaga spp. The orange complex includes Fusobacterium, Prevotella, and Campylobacter spp. (see Figure 8-1, R). The green and orange complexes include species recognized as pathogens in periodontal and nonperiodontal infections. The red complex consists of P. gingivalis, T. forsythia, and T. denticola. This complex is of particular interest because it is associated with bleeding on probing.The existence of complexes of species in plaque is another reflection of bacterial interdependency in the biofilm environment. A diagram of the association among subgingival species The data were derived from subgingival plaque samples taken from the mesial aspect of each tooth in 185 adult subjects. Each sample was individually analyzed for the presence of 40 subgingival species with the use of checkerboard DNA–DNA hybridization. Associations were sought among species via cluster analysis and community ordination techniques. The complexes to the left are comprised of species that are thought to colonize the tooth surface and to proliferate at anearly stage. The orange complex becomes numerically dominant later; it is thought to bridge the early colonizers and the red complex species, which become numerically more dominant during the later stages of plaque development. Surface Microroughness. Rough intraoral surfaces (e.g., crown margins, implant abutments, denture bases) accumulate and retain more plaque and calculus in terms of thickness, area, and colony-forming units. Ample plaque also reveals an increased maturity/pathogenicity of its bacterial components, which is characterized by an increased proportion of motile organisms and spirochetes and/or a denser packing of them.Smoothing an intraoral surface decreases the rate of plaque formation. Below a certain surface roughness (Ra < 0.2 μm), however,further smoothing does not result in an additional reduction in plaque formation. There seems to be a threshold level for surface roughness (Ra ≈ 0.2 μm) above which bacterial adhesion will be facilitated.Although surface free energy and surface roughness are two factors that influence plaque growth, the latter predominates. Individual Variables That Influence Plaque Formation. The rate of plaque formation differs significantly between subjects, and these differences may overrule surface characteristics. A distinction is often made between “heavy” (fast) and “light”(slow) plaque formers (see Video 8-2: Difference in Plaque Growth Between Heavy and Light Plaque Formers). A multiple regression analysis showed that the clinical wettability of the tooth surfaces, the saliva-induced aggregation of oral bacteria, and the relative salivary flow conditions around the sampled teeth explained 90% of the variation. Moreover, the saliva from light plaque formers reduced the colloidal stability of bacterial suspensions of, for example, S. sanguinis.Variation Within the Dentition. Within a dental arch, large differences in plaque growth rate can be detected. In general, early plaque formation occurs faster: in the lower jaw (as compared with the upper jaw); in molar areas; on the buccal tooth surfaces (as compared with palatal sites, especially in the upper jaw); and in the interdental regions (as compared with the buccal or lingual surfaces).Impact of Gingival Inflammation and Saliva. Several studies clearly indicate that early in vivo plaque formation is more rapid on tooth surfaces facing inflamed gingival margins than on those adjacent to healthy gingivae. These studies suggest that the increase in crevicular fluid production enhances plaque formation. Probably some substances from this exudate (e.g., minerals, proteins, carbohydrates) favor both the initial adhesion and/ or the growth of the early colonizing bacteria. In addition, it is known that, during the night, the plaque growth rate is reduced by some 50%.302 This seems surprising, because one would expect that reduced plaque removal and the decreased salivary flow at night would enhance plaque growth. The fact that the supragingival plaque obtains its nutrients mainly from the saliva appears to be of greater significance than the antibacterial activity of saliva.Impact of Patient’s Age. Although older studies were con tradictory, more recent papers clearly indicate that a subject’s age does not influence de novo plaque formation. In a study by Fransson and colleagues,100 no differences could be detected in de novo plaque formation between a group of young (20 to 25 years old) patients and a group of older (65 to 80 years old) subjects who abolished mechanical tooth cleaning measures for 21 days, neither in amount nor in composition.- This observation largely confirms data by Holm-Pedersen and colleagues and data by Winkel and coworkers. However, the developed plaque in the older patient group resulted in more severe gingival inflammation, which seems to indicate an increased susceptibility to gingivitis with aging. Spontaneous Tooth Cleaning. Many clinicians still think that plaque is removed spontaneously from the teeth, such as during eating. However, on the basis of the firm attachment between the bacteria and the surface, this seems unlikely. Even in the occlu- sal surfaces of the molars, plaque remains, even after chewing fibrous food (e.g., carrots, apples, chips). The inefficiency of the spontaneous plaque removal is neatly illustrated by the clinical pictures , which were taken before and after dinner starting from 4 days of undisturbed plaque formation. Only negligible differences in plaque extent could be observed. De Novo Subgingival Plaque Formation It is technically impossible to record the dynamics of subgingival plaque formation in an established dentition for the simple reason that one cannot sterilize a periodontal pocket. Some early studies involving the use of culturing techniques examined the changes within the subgingival microbiota during the first week after mechanical debridement and reported an only partial reduction of around 3 logs (from 108bacterial cells to 105 cells) followed by rapid regrowth toward nearly pretreatment levels (−0.5 log) within 7 days. The fast recolonization was explained by several factors. A critical review of the effectiveness of subgingival debridement, for instance, revealed that a high proportion of treated tooth surfaces (5% to 80%) still harbored plaque and/or calculus after scaling. These remaining bacteria were considered the primary source for the subgingival recolonization.278 Some pathogens penetrate the soft tissues or the dentinal tubules and eventually escape instrumentation Characteristics of Biofilm Bacteria (Life in “Slime City”) Metabolism of Dental Plaque Bacteria The majority of nutrients for dental plaque bacteria originate from saliva or gingival crevicular fluid, although the host diet provides an occasional but nevertheless important food supply. The transi- tion from gram-positive to gram-negative microorganisms observed in the structural development of dental plaque is paralleled by a physiologic transition in the developing plaque. The early colonizers (e.g., Streptococcus and Actinomyces spp.) use oxygen and lower the redox potential of the environment, which then favors the growth of anaerobic species.76,405 Many of the gram-positive early colonizers use sugars as an energy source. The bacteria that predominate in mature plaque are anaerobic and asaccharolytic (i.e., they do not break down sugars), and they use amino acids and small peptides as energy sources. Laboratory studies have demonstrated many metabolic interactions among the different bacteria found in dental plaque. For example, lactate and formate are byproducts of the metabolism of streptococci and Actinomyces spp.; they may be used in the metabolism of other plaque microorganisms, including Veillonella spp. and A. actinomycetemcomitans.The growth of P. gingivalis is enhanced by metabolic byproducts produced by other microorganisms, such as succinate from C.ochracea and protoheme from Campylobacter rectus.Overall, the total plaque population is more efficient than any one constituent organism at releasing energy from the available substrates.Metabolic interactions also occur between the host and the plaque microorganisms. The bacterial enzymes that degrade host proteins mediate the release of ammonia, which may be used by bacteria as a nitrogen source.Hemin iron from the breakdown of host hemoglobin may be important in the metabolism of P. gingivalis. Increases in steroid hormones are associated with significant increases in the proportions of P. intermedia found in subgingival plaque. These nutritional interdependencies are probably critical to the growth and survival of microorganisms in dental plaque, and they may partly explain the evolution of the highly specific structural interactions observed among bacteria in plaque. Communication Between Biofilm Bacteria Bacterial cells do not exist in isolation. In a biofilm, bacteria have the capacity to communicate with each other. One example of this is quorum sensing, in which bacteria secrete a signaling molecule that accumulates in the local environment and triggers a response such as a change in the expression of specific genes once they reach a critical threshold concentration. The threshold concentration is reached only at a high cell density, and therefore bacteria sense that the population has reached a critical mass or quorum. There is some evidence that intercellular communication can occur after cell–cell contact and that, in this case, communication may not involve secreted signaling molecules.Two types of signaling moleculeshave been detected from dental plaque bacteria: peptides released by gram-positive organisms during growth and a “universal” signa molecule called autoinducer 2 (AI-2). Peptide signals are produced by oral streptococci; they are recognized by cells of the same strain that produced them and possibly also by different species of streptococci. Responses are induced only when a threshold concentration of the peptide is attained, and thus the peptides act as cell density or quorum sensors. Local concentrations of signaling molecules may be enhanced in biofilms if the signals become trapped in the biofilm matrix. The streptococcal peptides are known as competence-stimulating peptides, because the major response to these signals is the induction of competence, a physiologic state during which cells are primed for DNA uptake and incorporation. In some species, such as S. mutans, a small proportion of the cells in a population respond to competence-stimulating peptides by lysing Lysis is considered to be an altruistic behavior that helps to disseminate genetic information throughout the population of S. mutans cells. Little is known about the specific functions of AI-2 in oral biofilms. However, this molecule has been demonstrated to play a role in mutualistic interactions between S. oralis and A. oris (A. naeslundii). Thus, in an in vitro model system, neither S. oralis nor A. oris formed biofilms in monoculture. When cultured together, these organisms grew abundantly on surfaces to form thick, confluent biofilms. This mutualistic behavior was only observed when AI-2 was present: disrupting the gene for AI-2 in S. oralis abrogated mutualistic growth. These data demonstrate that AI-2 is produced and sensed by oral bacteria and suggest that interbacterial communication is important for the development of dental plaque. Quorum sensing therefore appears to play diverse roles in, for example, modulating the expression of genes for antibiotic resistance, encouraging the growth of beneficial species in the biofilm, and discouraging the growth of competitors. Interactions Between Dental Plaque Bacteria There is some evidence from laboratory studies that nonpathogenic organisms in subgingival dental plaque can modify the behavior of periodontal pathogens. For example, long and short fimbriae of P. gingivalis are required for adhesion and biofilm formation. The expression of long fimbriae is downregulated in the presence of S. cristatus, and short fimbriae are downregulated by S. gordonii, S. mitis, or S. sanguinis. Changes in bacterial physiology after transitions from monoculture to mixed-species communities may be quite wide ranging. With the use of a proteomics approach to probe the phenotype of P. gingivalis, it has been shown that the expression of almost 500 P. gingivalis proteins is changed in model oral microbial communities that contain S. gordonii and F. nucleatum. At present, it is not clear how these changes affect the interaction between P. gingivalis and the host. In multispecies biofilms in which many bacteria are juxtaposed to cells of different species, interactions between genetically distinct microorganisms can be mutually beneficial . However, there are many examples of competitive interactions between different bacteria. For example, S. mutans produces antimicrobial peptides that have broad activity against bacteria in vitro. Other oral streptococci compete with S. mutans Several clinical studies followed the detection frequency and relative proportion of cariogenic species after periodontal therapy.They all suggest a relative increase in the number as well as the detection frequency of S. mutans up to 8 months after mechanical debridement. In a cross-sectional study, subgingival plaque samples from chronic periodontitis patients were tested for the presence and levels of S. mutans and putative periodontal pathogens.The patients were divided into four groups on the basis of the stage of periodontal treatment: untreated, after initial periodontal therapy, maintenance phase without periodontal surgery, and after periodontal surgery. The prevalence of mutans streptococci in the four study groups was equivalent. The shift toward a more cariogenic flora observed after initial and surgical periodontal therapy could be explained by a subgingival outgrowth by S. mutans occupying spots that became available after periodontal therapy (e.g., increased number of free adhesion/receptor sites), the creation of a new ecosystem in the subgingival area (i.e., a more anaerobic environment, changes in redox potential, pH, and nutrition) that allows or facilitates the growth of S. mutans species, and/or a downgrowth of S. mutans from the supragingival area in which the species could survive in the saliva. In addition, Socransky and coworkers showed that the total numbers of P. gingivalis, T. forsythia, and T. denticola are reduced 2 weeks after scaling and rootplaning and that concomitantly several orange complex organisms are also reduced in number at this point. However, the proportion of S. sanguinis in the population increased 2 weeks after treatment by scaling and root planing in conjunction with azithromycin or metronidazole and 3 months after treatment with doxycycline.Although these observations may be due to an antagonistic relationship between S. sanguinis and periodontal pathogens, they may also reflect the relatively high intrinsic antibiotic resistance of oral streptococci. In subgingival biofilms, oxygen is scarce, and therefore H2O2 production by bacteria is low. Oxidizing agents may be more important in the interaction between the host and the pathogen, because reactive oxygen species are a major component of the neutrophil response to bacteria. Another example of competitive interaction exists between streptococci and periodontopathogens. S. sanguinis, S. salivarius, and S. mitis have been shown to inhibit hard- and soft-tissue colonization of A. actinomycetemcomitans , P. gingivalis, and Prevotella intermedia in vitro. Biofilms and Antimicrobial Resistance Bacteria growing in microbial communities adherent to a surface do not “behave” the same way as bacteria growing suspended in a liquid environment (i.e., in a planktonic or unattached state). For example, the resistance of bacteria to antimicrobial agents is dramatically increased in the biofilm. Almost without exception, organisms in a biofilm are 1000 to 1500 times more resistant as compared with antibiotics in their planktonic state. The mechanisms of this increased resistance differ from species to species, from antibiotic to antibiotic, and for biofilms growing in different habitats. It is generally accepted that the resistance of bacteria to antibiotics is affected by their nutritional status, growth rate, temperature, pH, and prior exposure to subeffective concentrations of antimicrobial agents.Variations in any of these parameters will lead to a varied response to antibiotics within a biofilm. An important mechanism of resistance appears to be the slower rate of growth of bacterial species in a biofilm, which makes them less susceptible to many but not all antibiotics. The biofilm matrix, although not a significant physical barrier to the diffusion of antibiotics, does have certain properties that can retard antibiotic penetration. For example, strongly charged or chemically highly reactive agents can fail to reach the deeper zones of the biofilm, because the biofilm acts as an ion-exchange resin that removes such molecules from solution. In addition, extracellular enzymes such as β-lactamases, formaldehyde lyase, and formaldehyde dehydrogenase may become trapped and concentrated in the extracellular matrix, and as such inactivate some antibiotics (especially positively charged hydrophilic antibiotics). Bacterial Transmission and Translocation The transmission of pathogens from one locus to another is an important aspect of infectious diseases. In theory, such transmission may jeopardize the outcome of periodontal therapy; in addition, the transmission of pathogens from one person to another may be important in terms of disease transmission. The significance of such an intraoral transmission or of a vertical or horizontal transmission is, however, difficult to prove or to quantify. One of the first questions in this regard is, “Are oral bacteria transmissible between humans?” Molecular fingerprinting techniques have clearly illustrated that periodontal pathogens are transmissible within members of a family. This bacterial transmission between subjects (and even between animals and human beings) should not be confused with contagion (with the term contagious referring to the likelihood of a microorganism being transmitted from an infected to an uninfected host to create disease). Asikainen and coworkers used a genetic finger printing method called arbitrarily primed-polymerase chain reaction to genotype A. actinomycetemcomitans isolates from family members. In 11 of 12 families, they found identical A. actinomycetemcomitans genotypes among family members. This suggests the transmission of this microorganism among family members. However, it was more often encountered that children carried a genotype identical to one of their parents than that spouses carried an identical genotype. This indicates that, in terms of A. actinomycetemcomitans transmission, vertical transmission is more important than horizontal transmission. Similar observations were made for the transmission of cariogenic species from mother to child.The vertical or horizontal transmission of P. gingivalis has rarely been observed. Nonbacterial Inhabitants of the Oral Cavity Oral microbiology is often reduced to “oral bacteriology.” However, the oral cavity comprises a much more diverse microbiota than merely a bacterial one. Viruses, fungi, archaea, and protozoa can be encountered in the oral cavity of humans. Many of these species can reside in the oral cavity in harmony with the host as commensals, similar to oral bacteria, but they can also cause several oral diseases. Viruses Viral diseases of the oral mucosa and the perioral region are often encountered in dental practice. Viruses are important ulcerogenic and tumorigenic agents of the human mouth. The finding of an abundance of mammalian viruses in periodontitis lesions may Yeasts Many yeast species have been isolated from the oral cavity. The majority of isolates are Candida, and the most prevalent species is C. albicans . Together with C. albicans, some of the most common opportunistic fungal pathogens in humans are C. tropicalis, C. glabrata, C. krusei, C parapsilosis, C. guilliermondii, and C. dubliniensis. Candida spp. thrive in low-pH environments, and high Candida loads are associated with the presence of relatively low-diversity dental plaque that contains aciduric and acidogenic species such as Streptococcus spp. and Lactobacillus spp. In addition to C. albicans, C. glabrata is now emerging as an important agent in both mucosal and bloodstream infections. Yeasts such as Rhodotorula glutinis and Saccharomyces cerevisiae are rarely found in the oral cavity and are not known to cause oral infections. Cryptococcus neoformans is occasionally isolated from the mouth but usually from patients with pulmonary cryptococcosis. Protozoa The mouth is the entry port for many parasites that have adapted to the human host. Only a few parasites affect the oral cavity, but an increasing body of literature indicates that oral protozoa are more common than previously appreciated. Depending on the type of infection, the parasitic infectious agents can be divided into two categories: those that induce local infections and those that induce systemic infections with indirect effects on the oral cavity. Archaea Archaea are single-celled organisms that are as distinct from bac- teria as they are from eukaryotes. The role of archaea in oral diseases is only beginning to be explored. Methanogenic archaea produce methane from hydrogen gas (H2)/carbon dioxide (CO2) and sometimes from formate, acetate, methanol, or methylamine. Microbiologic Specificity of Periodontal Diseases Nonspecific Plaque Hypothesis During the mid-1900s, periodontal diseases were thought to result from an accumulation of plaque over time, eventually in conjunction with a diminished host response and increased host susceptibility with age. This theory, which is called the nonspecific plaque hypothesis, was supported by epidemiologic studies that correlated both age and the amount of plaque with evidence of periodontitis. According to the nonspecific plaque hypothesis, periodontal disease results from the “elaboration of noxious products by the entire plaque flora.” When only small amounts of plaque arepresent, the noxious products are neutralized by the host. Similarly, large amounts of plaque would cause a higher production of noxious products, which would essentially overwhelm the host’s defenses. Specific Plaque Hypothesis The specific plaque hypothesis underlines the importance of the qualitative composition of the resident microbiota. The pathogenicity of dental plaque depends on the presence of or an increase in specific microorganisms. This concept encapsulates that plaque that harbors specific bacterial pathogens may provoke periodontal disease because key organisms produce substances that mediate the destruction of host tissues. The association of specific bacterial species with disease came about during the early 1960s, when the microscopic examination of plaque revealed that different bacterial morphotypes were found in healthy versus periodontally diseased sites disease. Criteria for the Identification of Periodontopathogens During the 1870s, Robert Koch developed the classic criteria by which a microorganism can be judged to be a causative agent in human infections. These criteria, known as Koch’s postulates, stipulate that the causative agent must do the following: 1. Be routinely isolated from diseased individuals 2. Be grown in pure culture in the laboratory 3. Produce a similar disease when inoculated into susceptible labo- ratory animals 4. Be recovered from lesions in a diseased laboratory animal Difficulties exist with regard to the application of these criteria to polymicrobial diseases, and the applicability of Koch’s postulates has been increasingly challenged in recent years. In the case of periodontitis, there are three primary problems: (1) the inability to culture all of the organisms that have been associated with disease (e.g., many of the oral spirochetes); (2) the difficulties inherent in defining and culturing sites of active disease; and (3) the lack of a good animal model system for the study of periodon- titis. In fact, if the ecologic plaque hypothesis proves correct, it must be inherently impossible to fulfill Koch’s postulates, because no single organism or group of organisms is responsible for all cases of disease. Sigmund Socransky, a researcher at the Forsyth Dental Center in Boston, proposed criteria by which periodontal microorganisms may be judged to be potential pathogens.363 According to these criteria, a potential pathogen must do the following: 1. Be associated with disease, as evidenced by increases in the number of organisms at diseased sites 2. Be eliminated or decreased in sites that demonstrate the clinical resolution of disease with treatment 3. Induce a host response in the form of an alteration in the host cellular or humoral immune response 4. Be capable of causing disease in experimental animal models 5. Produce demonstrable virulence factors that are responsible for enabling the microorganism to cause the destruction of the periodontal tissues On the basis of these criteria, data that support the role of A. actinomycetemcomitans and P. gingivalis as periodontal pathogens have been presented earlier in this chapter. The association and elimination criteria are discussed in the preceding sections. Ecological Plaque Hypothesis During the 1990s, Marsh and coworkers developed the “ecological plaque hypothesis” as an attempt to unify the existing theories regarding the role of dental plaque in oral disease (Figure 8-35). According to the ecological plaque hypothesis, both the total amount of dental plaque as well as the specific microbial composition of plaque may contribute to