Introduction to Chemical Process Industries PDF
Document Details
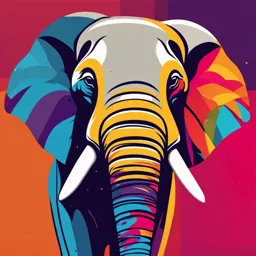
Uploaded by PremierBeryllium
Batangas State University
Engr. Keyza Jean M. Rivera
Tags
Summary
This document introduces the chemical process industries, outlining the various sectors, unit operations, and unit processes involved. It explores the differences between batch and continuous processes, steady state, and methods for process safety analysis. It's a fundamental resource for understanding the field.
Full Transcript
Introduction to Chemical Process Industries Engr. Keyza Jean M. Rivera BS Chemical Engineering, BatStateU MS Environmental Engineering, U.P. Diliman Introduction to Chemical Processing The business of the chemical industry is to change the chemical structur...
Introduction to Chemical Process Industries Engr. Keyza Jean M. Rivera BS Chemical Engineering, BatStateU MS Environmental Engineering, U.P. Diliman Introduction to Chemical Processing The business of the chemical industry is to change the chemical structure of natural materials in order to derive products of value to other industries or in daily life. Chemicals are produced from these raw materials – principally minerals, metals, and hydrocarbons in a series of processing steps. Further treatment, such as mixing and blending, is often required to convert them into end-products (e.g., paints, adhesives, medicines and cosmetics). Thus the chemical industry covers a much wider field than what is usually called “chemicals” since it also includes products such as artificial fibres, resins, soaps, paints, photographic films, and more. Main Sectors of Chemical Industry basic inorganics: acids, alkalis, and salts, mainly used elsewhere in industry; and industrial gases, such as oxygen, nitrogen, and acetylene basic organics: feedstocks for plastics, resins, synthetic rubbers, and synthetic fibres; solvents and detergent raw materials; dyestuffs and pigments fertilizers and pesticides (including herbicides, fungicides, and insecticides) plastics, resins, synthetic rubbers, cellulosic and synthetic fibres pharmaceuticals (drugs and medicines) paints, varnishes, and lacquers soaps, detergents, cleaning preparations, perfumes, cosmetics, and other toiletries miscellaneous chemicals, such as polishes, explosives, adhesives, inks, photographic films, and chemicals UNIT Unit Operations gives idea OPERATIONS about science related to specific physical operation; different equipments-its design, material of construction and Unit Operations give idea about science-related operation; and calculation to specific physical operation; different of various physical equipment and its design, material of parameters (mass flow, heat flow, mass balance, construction, and operation; and calculation of power and force etc.). various physical parameters (mass flow, heat flow, mass balance, power, and force etc.). Examples of unit operations are drying, evaporation, distillation, crystallization, leaching, filtration, extraction, etc. Unit Process The is the process in which chemical changes take place to the material present in the reaction and result into a product. This basically consists of a reaction between two or more chemicals which result in another chemical, such as sulphonation, nitration, oxidation, halogenation, and many more. Example 1 Example 2 Electrolysis of NaCl Solution Production of Hydrogen NaCl is fed to an electrolysis Hydrogen is produced using a very popular method, steam-methane cell in which electricity is reforming reaction. In this method, methane reacts with steam at a passed and sodium pressure under 3–25 bar in the presence of a nickel catalyst at about hydroxide is produced. In 750 ℃ to produce hydrogen. In this steam-methane reforming this reaction, decomposition reaction, chemical reaction under pressure and temperature is being reaction takes place. conducted to react methane with steam and generate hydrogen. Difference Between Unit Operation and Unit Process Unit Operation Unit Process Process in which physical changes and not chemical Process in which chemical changes take place are changes take place are known as unit operation. considered as unit process. Mixing, blending, Bromination, halogenation, crushing, distillation, etc. are considered as sulphonation, etc. are called unit process. unit operations. Roles of Chemical Engineer in a Processing Plant Chemical engineering involves the production and manufacturing of products through chemical processes. This includes designing equipment, systems, and processes for refining raw materials and for mixing, compounding, and processing chemicals. Roles of Chemical Engineer in a Processing Plant Chemical engineers translate processes developed in the lab into practical applications for the commercial production of products, and then work to maintain and improve those processes. They rely on the main foundations of engineering: math, physics, and chemistry. Biology also plays an increasingly important role. Broadly, chemical engineers conceive and design processes involved in chemical manufacturing. The main role of chemical engineers is to design and troubleshoot processes for the production of chemicals, fuels, foods, pharmaceuticals, and biologicals, to name just a few. They are most often employed by large-scale manufacturing plants to maximize productivity and product quality while minimizing costs. Chemical engineers who work in business Roles of Chemical Engineer and management offices often visit research in a Processing Plant and production facilities. Interaction with other people and team collaboration are critical to the success of projects involving chemical engineering. Chemical engineers typically work in manufacturing plants, research laboratories, or pilot plant facilities. They work around large-scale production equipment that is housed both indoors and outdoors. Accordingly, they are often required to wear personal protective equipment (e.g., hard hats, goggles, and steel-toe shoes). BATCH PROCESS In a batch process, an allotment of starting material is introduced into the process, and a sequence of steps to treat that material is started and finished within a certain period of time, often within the same piece of equipment. The process is then interrupted, the processed material is removed, another allotment of the starting material is introduced, and the sequence of steps is repeated. Example: materials are loaded into a reactor, a reaction is carried out in the reactor, and then the final materials are removed. CONTINUOUS PROCESS A continuous process operates without interruption in the flows and reactions of the process. The starting material enters continuously, is usually subjected to various steps by moving from one piece of equipment to another, and exits the process continuously. Example: materials continually flow into and out of a reactor, while the reaction proceeds as the material moves through the reactor. STEADY STATE Steady-state is a condition on any process in which none of the characteristics of interest (temperatures, flow rates, pressures, and so forth) change with time. A process that is not steady-state is termed unsteady-state, time-dependent, or transient. Graphically Representing Processes Other (fishbone diagram, molecular Process Flow interaction diagram) Diagram Block Diagram Piping and Instrumentation Diagram Block Diagram Includes an overview of the process, treating processes as black boxes that are used to represent either a single equipment item or a combination of equipment items that collectively accomplish one of the principal steps in the process. Process Flow Diagram Includes major unit operations (components), their interconnections, and sometimes state conditions (e.g., temperatures, pressures). It may also include a stream table. P&ID Includes piping, sensors, and other instrumentation Process Hazard Analysis Persons performing the process hazard analysis should be knowledgeable and experienced in relevant chemistry, engineering, and process operations. Each analysis team normally includes at least one person who is thoroughly familiar with the process being analyzed and one person who is competent in the hazard analysis methodology being used. The priority order used to determine where within the facility to begin conducting process hazard analyses is based on the following criteria: · extent and nature of the process hazards; number of potentially affected workers; operating and incident history of the process; and age of the process. METHODS FOR CONDUCTING PROCESS SAFETY ANALYSIS “What if” method The “what if?” method asks a series of questions to review potential hazard scenarios and possible consequences and is most often used when examining proposed modifications or changes to the process, materials, equipment, or facility. METHODS FOR CONDUCTING PROCESS SAFETY ANALYSIS Checklist The “checklist” method is similar to the “what if?” method, except that a previously developed checklist is used which is specific to the operation, materials, process, and equipment. This method is useful when conducting pre-startup reviews upon completion of initial construction or following major turnarounds or additions to the process unit. A combination of the “what if?” and “checklist” methods is often used when analyzing units that are identical in construction, materials, equipment, and process. METHODS FOR CONDUCTING PROCESS SAFETY ANALYSIS HAZOP The hazard and operability (HAZOP) study method is commonly used in the chemical and petroleum industries. It involves a multi-disciplinary team, guided by an experienced leader. The team uses specific guide words, such as “no”, “increase”, “decrease”, and “reverse”, which are systematically applied to identify the consequences of deviations from design intent for the processes, equipment, and operations being analyzed. METHODS FOR CONDUCTING PROCESS SAFETY ANALYSIS Fault Tree/ Event Tree Analysis Fault tree/event tree analyses are similar, formal deductive techniques used to estimate the quantitative likelihood of an event occurring. Fault tree analysis works backward from a defined incident to identify and display the combination of operational errors and/ or equipment failures which were involved in the incident. Event tree analysis, which is the reverse of fault tree analysis, works forwards from specific events, or sequences of events, in order to pinpoint those that could result in hazards, and thereby calculate the likelihood of an event’s sequence occurring. METHODS FOR CONDUCTING PROCESS SAFETY ANALYSIS Failure Mode and Effect Analysis The failure mode and effects analysis method tabulates each process system or unit of equipment with its failure modes, the effect of each potential failure on the system, or unit and how critical each failure could be to the integrity of the system. The failure modes are then ranked in importance to determine which is most likely to cause a serious incident. Management of Change Chemical process facilities should develop and implement programs which provide for the revision of process safety information, procedures, and practices as changes occur. Such programs include a system of management authorization and written documentation for changes to materials, chemicals, technology, equipment, procedures, personnel, and facilities that affect each process. Management of change programs in the chemical industry, for example, include the following areas: change of hydrocarbon process technology; changes in facility, equipment, or materials (e.g., catalysts or additives); management of change personnel, and organizational and personnel changes; temporary changes, variances, and permanent changes; enhancement of process safety knowledge; and management of subtle change (anything which is not replacement in kind) or non-routine changes. ANY QUESTIONS? Thank you! Petroleum and Petrochemical Industry Background of the Study Petrochemical Industry is a strategic sector of the economy that could anchor the country’s development. Because of its strong linkages upstream, midstream and downstream, the sector provides robust multiplier effects on other main sectors of the economy such as construction, electronics and computer, medical services, transportation and automotive, packaging, education, telecommunications, electrical and water distribution, agriculture and fishery, and furniture, among others. Background of the Study The establishment of the Philippine’s first naphtha cracker facility by JG Summit Olefins Corp. (JGSOC) is expected to provide the first step for upstream integration of the petrochemical industry. It provides a powerful means to actualize the potential of developing various downstream operations that would broaden the petrochemical industry product range and deepen its primary product offerings in the country. In the petrochemical industry, the organic chemicals produced in the largest volumes are methanol, ethylene, propylene, butadiene, benzene, toluene, and xylenes. Ethylene, propylene, and butadiene, along with butylenes, are collectively called olefins, which belong to a class of unsaturated aliphatic hydrocarbons having the general formula CnH2n. Olefins contain one or more double bonds, which make them chemically reactive. Benzene, toluene, and xylenes, commonly referred to as aromatics, are unsaturated cyclic hydrocarbons containing one or more rings. Olefins, aromatics, and methanol are precursors to a variety of chemical products and are generally referred to as primary petrochemicals. How Petrochemicals are Formed: The Petrochemical Plant A vast majority of petrochemicals are obtained from fossil fuels, such as natural gas and crude oil. The rest usually comes from coal and biomass. A major, if not the most important element of the industry is the petrochemical plant itself. These plants are the powerhouses that convert the natural resources into petrochemicals to be used as the building blocks for other processes and products. How Petrochemicals are Formed: The Petrochemical Plant The process requires energy in huge amounts and involves a distillation phase, in which hydrocarbons are separated from the fossil fuels. The separated hydrocarbons are then sent to facilities called “crackers”. These facilities convert them into useful chemicals, known as “feedstock”. Chemical feedstock refers to any type of unprocessed material used in a manufacturing process as a base material to be transformed into another end-product. The Primary Classes of Petrochemical Feedstock Includes: Aromatics Benzene, Toluene, and Xylenes Olefins Methanol Ethylene, Propylene, and Butadiene Basic petrochemicals such as these are the basis of many products, including plastic, paper, fibres, adhesives, and detergents. They are also responsible for producing a whole range of chemicals known as petrochemical intermediates. These derivatives are more complex versions of primary petrochemicals. They are also used in making a variety of products. Some typical examples include: Vinyl Acetate – used in making paint Ethylene Glycol – used in polyester textile fibres Key Products Made from Petrochemicals Petrochemicals form the backbone of modern economies and are found in a wide range of consumer and industrial products, many of which are considered essential for daily use. Highly versatile, petrochemicals can be found in everything from synthetic tires to automotive headlamps and lenses. While most individuals associate these chemicals with plastics, their potential uses span a much broader spectrum of applications, from household groceries to rocket propulsion. How Petrochemicals Are Used: Products of the Petrochemical Industry ü Hydrocarbons in manufacturing and transportation ü Resins, films, and plastics in medicine ü Preservatives in food or use in food packaging ü Agriculture, from plastics and pesticides to fertilizers ü Household products, such as carpeting, detergents, and more ü Rubber ü Synthetic fibers ü Adhesives ü Dyes ü Paints and coatings The Order of Petrochemical Processing: From Raw Material to Final Usage There are five steps in the order of petrochemical processing that turn raw materials into a variety of products. 01 Feedstocks 04 Manufacturing Products 02 Basic Chemicals 05 Consumer Products 03 Chemicals Intermediates/Derivatives The Many Uses of Petrochemicals Primary Hydrocarbons Raw crude oil and natural gas are purified into a relatively small number of hydrocarbons (combinations of hydrogen and carbon). These are used directly in manufacturing and transportation or act as feedstock to make other chemicals. Methane Butanes a greenhouse gas that can hydrocarbon gases that be used as fuel and is often are generally used for included in rocket fuel fuel and in industry Ethylene Butadienes used to make plastics and films, used in the manufacture as well as detergents, synthetic of synthetic rubbers lubricants, and styrenes (used to make protective packaging) Propylene BTX a colorless, odorless gas used for fuel Benzene, toluene, and xylene are aromatic and to make polypropylene, a versatile hydrocarbons. A major part of gasoline, benzene is also plastic polymer used to make products used to make nylon fibers which, in turn, are used to ranging from carpets to structural foam make clothing, packaging, and many other products. Other Uses of Petrochemicals Medicine Petrochemicals play many roles in medicine because they are used to create resins, films, and plastics. Here are just a few examples: 1. Phenol and Cumene are used to create a substance that is essential for manufacturing penicillin (an extremely important antibiotic) and aspirin. 2. Petrochemical resins are used to purify drugs, thus cutting costs and speeding the manufacturing process. 3. Resins made from petrochemicals are used in the manufacture of drugs including treatments for AIDS, arthritis, and cancer. 4. Plastics and resins made with petrochemicals are used to make devices such as artificial limbs and skin. 5. Plastics are used to make a huge range of medical equipment including bottles, disposable syringes, and much more. Other Uses of Petrochemicals Food Petrochemicals are used to make most food preservatives that keep food fresh on the shelf or in a can. In addition, you'll find petrochemicals listed as ingredients in many chocolates and candies. Food colorings made with petrochemicals are used in a surprising number of products including chips, packaged foods, and canned or jarred foods. Other Uses of Petrochemicals Agriculture More than a billion pounds of plastic, all made with petrochemicals, find use annually in U.S. agriculture. The chemicals are used to make everything from plastic sheeting and mulch to pesticides and fertilizers. Plastics are also used to make twine, silage, and tubing. Petroleum fuels are also used to transport foods (which are, of course, stored in plastic containers). Other Uses of Petrochemicals Household Products Because it is used to make plastics, fibers, synthetic rubber, and films, petrochemicals are used in a bewildering array of household products. To name just a few: ü Carpeting ü Stocking ü Crayons ü Perfume ü Detergents ü Safety glass ü Dyes ü Shampoo ü Fertilizers ü Soft contact lenses ü Milk jugs ü Wax Principal Sources The principal source of alkanes is petroleum with accompanying natural gas. Formed along with the alkanes are the cycloalkanes known to petroleum industry as naphthenes. The three major sources of alkanes throughout the world are the fossil fuels namely natural gas, petroleum, and coal. These fossil fuels account for approximately 90% of the total energy consumed in the United States. Nuclear electric power and hydro electric power make up the most of the remaining 10%. In addition, these fossil fuels provide the bulk of organic chemicals consumed worldwide. Natural Gas Natural gas consists of approximately 90 to 95% methane, 5 to 10% ethane, and a mixture of other low boiling alkanes, chiefly propane, butane, and 2-methylpropane. The current widespread use of ethylene as the organic chemical industry’s most important building block is due largely to the ease with which ethane can be separated from natural gas and cracked into ethylene. Cracking is a process whereby saturated hydrocarbon is converted into an unsaturated hydrocarbon plus H2. Ethane is cracked by heating it in a furnace at 800 to 900°C for a fraction of a second. Petroleum Petroleum is a thick, viscous liquid mixture of literally thousands of compounds, most of them hydrocarbons, formed from the decomposition of ancient marine plants and animals. Petroleum and petroleum-derived products fuel automobiles, aircraft, and trains. They provide most of the greases and lubricants required for the machinery of our highly industrialized society. Furthermore, petroleum along with natural gas, provide close to 90% of the organic raw materials for the synthesis and manufacture of synthetic fibers, plastics, detergents, drugs, dyes, and a multitude of other products. Petroleum The fundamental separation process in refining petroleum is fractional distillation. Practically all crude oil that enters a refinery goes to distillation units where it is heated to temperatures as high as 370 to 425°C and separated into fractions. Each fraction contains a mixture of hydrocarbons that boils within a particular range. Following are the common names associated with several of these fractions along the major uses of each. 1. Gases 2. Naphtha 3. Kerosene 4. Fuel Oil 5. Lubricating Oil 6. Asphalt Gases Boiling below 20°C are taken off at the top of the distillation column. This fraction is a mixture of low- molecular-weight hydrocarbons, predominantly propane, butane, and 2-methyl propane, substances that can be liquefied under pressure at room temperature. The liquefied mixture, known as liquefied petroleum gas (LPG), can be stored and shipped in metal tanks and is convenient source of gaseous fuel for home heating and cooking. Naphtha Naphtha is a mixture of C5 to C12 alkanes and cycloalkanes. The naphthas also contain small amounts of benzene, toluene, xylene, and other aromatic hydrocarbons. The light naphtha fraction, BP 20-150°C, is the source of straight-run gasoline and averages approximately 25% of crude petroleum. In a sense, naphthas are the most valuable distillation fractions because they are useful not only as fuel but also as sources of raw materials for the organic chemical industry. Others Kerosene is a mixture of C9 to C15 hydrocarbons. Fuel oil, BP 250 to 400°C, is a mixture of C15 to C18 hydrocarbons. It is from this fraction that diesel fuel is obtained. Lubricating oil and heavy fuel oil distill from the column at the temperatures above 350°C. Asphalt is the name given to the black, tarry residue remaining after removal of the other volatile fractions. Reforming The most common reforming processes are cracking, as illustrated by thermal conversion of ethane to ethylene, and a catalytic reforming. Catalytic reforming is illustrated by the conversion of hexane first to cyclohexane and then to benzene. Reforming Gasoline is a complex mixture of C6 to C12 hydrocarbons. The quality of gasoline as a fuel for internal combustion engines is expressed in terms of octane rating. The procedure for measuring octane rating was established in 1929. Two compounds were selected as reference fuels. One of these, 2,2,4-trimethylpentane (―isooctane࠱), has very good antiknock properties (the fuel/air mixture burns smoothly in the combustion chamber) and was assigned an octane rating of 100. Reforming The name ―isooctane࠱ as used here is a trivial name; its only relation to the name 2,2,4-trimethylpentane is that both show eight carbon atoms. Heptane, the other reference compound, has poor antiknock properties and was assigned an octane rating of 0. Reforming The octane rating of a particular gasoline is that percent isooctane in a mixture of isooctane and heptane that has equivalent knock properties. For example, the knock properties of 2-methylhexane are the same as those mixture of 42% isooctane and 58% heptane; therefore, the octane rating of 2- methylhexane is 42. octane itself has an octane rating of -20, which means that it produces even more engine knocking than heptane. Upstream, Midstream and Downstream Processing Upstream This refers to anything having to do with the exploration and production of oil and natural gas. Geologic surveys and any information gathering used to locate specific areas where minerals are likely to be found is commonly called ‘exploration.’ The term ‘upstream’ also includes the steps involved in the actual drilling and bringing oil and natural gas resources to the surface, referred to as ‘production’. Upstream, Midstream and Downstream Processing Midstream The ‘midstream’ segment of the oil and natural gas industry refers to anything required to transport and store crude oil and natural gas before they are refined and processed into fuels and key elements needed to make a very long list of products we use every day. Midstream includes pipelines and all the infrastructure needed to move these resources long distances, such as pumping stations, tank trucks, rail tank cars and transcontinental tankers. Upstream, Midstream and Downstream Processing Downstream The final sector of the oil and natural gas industry is known as ‘downstream.’ This includes everything involved in turning crude oil and natural gas into thousands of finished products we depend on every day. Some of the more obvious products are fuels like gasoline, diesel, kerosene, jet fuels, heating oils and asphalt for building roads. But long- chain hydrocarbons found in both oil and natural gas are used to make far less obvious products like synthetic rubbers, fertilizers, preservatives, containers, and plastics for parts in countless products. Environmental Impacts As we move towards zero-emissions and 100% clean energy, the oil and gas industry is launching a last-ditch effort to protect its profit, betting big on petrochemicals: toxic chemicals made from oil and gas that are used to make plastics, industrial chemicals, and pesticides. Environmental Impacts 1. Petrochemical plants use oil and gas to make plastics, industrial chemicals, and pesticides. Lifecycle of Petrochemicals: Shale gas is extracted through fracking. Fracked gas liquids are separated from fracked gas, and refined into their distinct products, such as propane, butane, and ethane. Under high pressure and temperature, ethane is broken down into ethylene — the building block of most plastics. Toxic chemicals from discarded plastics can leach into groundwater. Environmental Impacts 2. Big Oil is betting on petrochemicals to stay afloat. The Gulf Coast is especially attractive to the petrochemical industry because of its proximity to international ports, where most of the products will be exported. Above, plastic waste fills a beach in Manila, Philippines. As the clean energy transition threatens Big Oil’s bottom line, the industry is looking to offload a glut of cheap oil and fracked gas and build up its profit margins by turning to petrochemicals. Environmental Impacts 3. Communities of color and indigenous communities pay the price while polluters profit. Petrochemical companies promise economic benefits when they come to town, but the reality is that communities pay the price while polluters profit. Companies often bring in workers to operate the plants, rather than hiring locally. They benefit from special tax breaks and other incentives that deny economic benefits to the community. Environmental Impacts 3. Communities of color and indigenous communities pay the price while polluters profit. For far too long, polluters have targeted communities of color, indigenous communities, and low-income communities where they think they can get away with it. New petrochemical facilities would dump even more pollution in these same communities, exacerbating health problems like cancer and asthma. Environmental Impacts 4. Petrochemicals are fueling the climate crisis. Petrochemicals are a carbon bomb that threaten to cancel out the progress we’ve made on solving the climate crisis. As we make the switch to clean energy and transportation, petrochemicals are on track to be the largest driver of world oil demand. New petrochemical facilities would extend the life of the oil and gas industry and undermine efforts to keep fossil fuels in the ground. Petrochemical facilities are energy- intensive and dump an enormous amount of carbon pollution into the air. Environmental Impacts 5. A petrochemical boom will lock in plastic pollution for decades. Our planet is drowning in plastics that pollute our air and water. The only way to stop the plastic pollution crisis is to make and use less plastics. Plastic waste ends up in landfills, where its toxic chemicals leach into groundwater and flow downstream into lakes and rivers. Or it ends up in our oceans — scientists estimate 8 million metric tons of plastic enter the ocean every year. Environmental Impacts 5. A petrochemical boom will lock in plastic pollution for decades. There, it threatens wildlife and poisons our food chain. The plastics industry is counting on more petrochemical plants to lock in plastics consumption, and its profits, for decades to come. Half of all plastics ever manufactured have been made in the last 15 years. Environmental Impacts 6. Petrochemical products are poisoning our homes and workplaces. Petrochemical products are everywhere, exposing us to dangerous chemicals that threaten our health. The plastic products we use at home everyday leach additives like BPA into our families’ food and water, which end up in our bloodstreams. These chemicals are linked to cancers, hormone disruption, and developmental problems. Environmental Impacts 6. Petrochemical products are poisoning our homes and workplaces. Petrochemicals are a major threat to workers, too. Exposure to industrial chemicals commonly used in shops and offices can lead to poisoning, skin rashes, and cancers. Some chemicals, like paint strippers that use methylene chloride, can even cause heart failure and sudden death. Any questions? THANK YOU! VEGETABLE OILS AND BIOFUELS INTRODUCTION Vegetable oils are obtained from plants. They are important ingredients in many food, and can be hardened through a chemical process to make, for example, margarine. They can also be used as fuels, for example as biodiesel. Emulsifiers are food additives that prevent oil and water mixtures in food from separating. Vegetable oils are natural oils found in seeds, nuts, and some fruit. These oils can be extracted. The plant material is crushed and pressed to squeeze the oil out. Olive oil is obtained this way. Sometimes the oil is more difficult to extract and has to be dissolved in a solvent. Once the oil is dissolved, the solvent is removed by distillation, and impurities such as water are also removed, to leave pure vegetable oil. Sunflower oil is obtained in this way. Several factors impact the oxidative stability of native oils, including, among others, the level of unsaturation and the amount and types of natural antioxidants present in the oil. STRUCTURE OF VEGETABLE OILS Molecules of vegetable oils consist of glycerol and fatty acids. In the diagram, you can see how three long chains of carbon atoms are attached to a glycerol molecule to make one molecule of vegetable oil. Vegetable oils have higher boiling points than water. This means that food can be cooked or fried at higher temperatures than they can be cooked or boiled in water. Food cooked in vegetable oils. 01 cooks faster than if it were boiled and has different flavors than if it were boiled. However, vegetable oils are a source of energy in the diet. Food cooked in vegetable oils releases more energy when it is eaten than food cooked in water. This can have an impact on our. 02 health. For example, people who eat a lot of fried food may become overweight. FATS AND OILS The fatty acids in some vegetable oils are saturated: they only have single bonds between their carbon atoms. 01 SATURATED 02 UNSATURATED Unsaturated oils tend to be liquid at Saturated oils tend to be solid at room room temperature, and are useful for temperature, and are sometimes called frying food. They can be divided into two vegetable fats instead of vegetable oils. Lard categories: monounsaturated fats have is an example of a saturated oil. The fatty one double bond in each fatty acid acids in some vegetable oils are unsaturated: polyunsaturated fats have many double they have double bonds between some of bonds. Unsaturated fats are thought to their carbon atoms. be a healthier option in the diet than saturated fats. FATS AND OILS Vegetable oils do not dissolve in water. If oil and water are shaken together, tiny droplets of one liquid spread through the other liquid, forming a mixture called an emulsion. EMULSIONS 01 Emulsions are thicker (more viscous) than the oil or water they contain. This makes them useful in food such as salad dressings and ice cream. 02 Emulsions are also used in cosmetics and paints. There are two main types of emulsion: a. oil droplets in water (milk, ice cream, salad cream) b. water droplets in oil (margarine, butter, skin cream) 03 If an emulsion is left to stand, eventually a layer of oil will form on the surface of the water. Emulsifiers are substances that stabilise emulsions, stopping them separating out. Egg yolk contains a natural emulsifier. Mayonnaise is a stable emulsion of vegetable oil and vinegar with egg yolk. EMULSIONS Emulsifier molecules have two different ends: a hydrophilic end - 'water-loving' - that forms chemical bonds with water but not with oils, a hydrophobic end - 'water-hating’ -, that forms chemical bonds with oils but not with water. Lecithin is an emulsifier commonly used in food. It is obtained from oil seeds and is a mixture of different substances. A molecular model of one of these substances is seen in the diagram. The hydrophilic 'head' dissolves in the water and the hydrophobic 'tail' dissolves in the oil. In this way, the water and oil droplets become unable to separate out. BROMINE WATER TEST Unsaturated vegetable oils contain double carbon-carbon bonds. These can be detected using bromine water (just as alkenes can be detected). 01 02 Bromine water becomes colourless when Bromine water can also be used to determine the shaken with an unsaturated vegetable oil, amount of unsaturation of a vegetable oil. The but it stays orange-brown when shaken with more unsaturated a vegetable oil is, the more a saturated vegetable fat. bromine water it can decolourise. HYDROGENATION Higher tier Saturated vegetable fats are solid at room temperature, and have a higher melting point than unsaturated oils. This makes them suitable for making margarine, or for commercial use in the making of cakes and pastry. Unsaturated vegetable oils can be ‘hardened’ by reacting them with hydrogen, a reaction called hydrogenation. During hydrogenation, vegetable oils are reacted with hydrogen gas at about 60ºC. A nickel catalyst is used to speed up the reaction. The double bonds are converted to single bonds in the reaction. In this way unsaturated fats can be made into saturated fats – they are hardened. The structure of part of a fatty acid. RURAL VEGETABLE OIL PRODUCTION Rural oil extraction usually occurs near the areas of raw material production. This provides the small scale processor with access to raw materials, helps to ensure that perishable oil crops are processed quickly, and reduces transport costs. For rural communities and the urban poor, unrefined vegetable oils contribute significantly to the total amount of oil consumed. Crude oils are affordable to low-income groups and serve as important sources of beta- carotene and tocopherols. To maintain the quality of the raw material, care is needed during and after the harvesting of oil-bearing fruits that are perishable and susceptible to fat breakdown. Bruising of fresh palm fruits accelerates lipase activity leading to fat degradation. Oil-bearing crops such as shea nuts are prone to mold infestation during storage. This is curtailed by heat treatment: steaming or boiling, coupled with sun-drying to reduce the moisture content. RURAL VEGETABLE OIL PRODUCTION 01 Storage 04 Dehydration 02 Pre-treatment 05 Pressing Cakes 03 Extraction STORAGE The moisture content of oil seeds and nuts influences the quality of raw materials over time. In most rural operations, sun-drying reduces the moisture content of oil seeds to below 10 percent. Adequate ventilation or aeration of the seeds or nuts during storage ensures that low moisture levels are maintained and microbial development is avoided. This is important in the storage of groundnuts which are highly susceptible to aflatoxin contamination through the growth of Aspergillus flavus. STORAGE Since aflatoxins and pesticides are not removed by rural extraction techniques, microbial contamination and the application of insecticides should be avoided. There is a need for storage practices which are affordable and available to the small-scale processor. Perishable raw materials such as palm fruits should be processed as soon as possible after harvesting. In humid developing countries, the sun-drying of oil seeds with a high moisture content, such as mature coconut, is slow and inefficient. Such conditions promote mold growth which results in high free fatty acid levels and poor organoleptic qualities. Coconut oil for human consumption should be obtained soon after harvest. PRETREATMENT The first operation after harvesting involves sterilization and heat treatment by steaming or boiling, this inactivates lipolytic enzymes which could cause rapid degradation of the oil and facilitates the pulping of the mesocarp for oil extraction. "Sterilised" palm fruits are pulped in a wooden pestle and mortar or mechanised digestor. Decortication or shelling separates the oil-bearing portion of the raw material and eliminates the parts that have little or no nutritional value. Small-scale mechanical shellers are available for kernels and nuts although manual cracking is still prevalent. Most oil seeds and nuts are heat-treated by roasting to liquify the oil in the plant cells and facilitate its release during extraction. All oil seeds and nuts undergo this treatment except palm fruits for which "sterilization" replaces this operation. To increase the surface area and maximize oil yield, the oil-bearing part of groundnuts, sunflower, sesame, coconut, palm kernel and shea nuts is reduced in size. Mechanical disc attrition mills are commonly used in rural operations. EXTRACTION In oil extraction, milled seed is mixed with hot water and boiled to allow the oil to float and be skimmed off. The milled oil seed is mixed with hot water to make a paste for kneading by hand or machine until the oil separates as an emulsion. In groundnut oil extraction, salt is usually added to coagulate the protein and enhance oil separation. A large rotating pestle in a fixed mortar system can be powered by motor, humans or animals to apply friction and pressure to the oil seeds to release oil at the base of the mortar. Other traditional systems used in rural oil extraction include the use of heavy stones, wedges, levers and twisted ropes. For pressing, a plate or piston is manually forced into a perforated cylinder containing the milled or pulped oil mass by means of a worm. DEHYDRATION By boiling in shallow pans, traces of water in crude oil are removed after settling. This is common in all rural techniques which recognize the catalytic role of water in the development of rancidity and poor organoleptic qualities. PRESSING CAKES A by-product of processing, the pressed cake, may be useful depending on the oil extraction technique applied. Cakes from water-extracted oil are usually depleted of nutrients. Other traditional techniques, for instance, those used for groundnut and copra ensure that the byproducts, if handled with care, are suitable for human consumption. LARGE SCALE PRODUCTION 01 STORAGE 02 PROCESSING 03 OIL REFINING STORAGE Many steps in industrial processing find their origin in the traditional processes. In large-scale operations, oilseeds are dried to less than 10 percent moisture. They may be stored for prolonged time periods under suitable conditions of aeration with precautions against insect and rodent infestation. Such storage reduces mold infection and mycotoxin contamination and minimizes biological degradative processes which lead to the development of free fatty acids and color in the oil. Oil-bearing fruits such as olive and palm are treated as quickly as possible. Palm is sterilized as a first step in processing. Adipose tissues and fish-based raw materials (that is, the body or liver) are rendered within a few hours by boiling to destroy enzymes and prevent oil deterioration. PROCESSING Oilseeds are generally cleaned of foreign matter before dehulling. The kernels are ground to reduce size and cooked with steam, and the oil is extracted in a screw or hydraulic press. The pressed cake is flaked for later extraction of residual fat with solvents such as "food grade" hexane. Oil can be directly extracted with solvent from products which are low in oil content, that is, soybean, rice bran and corn germ. After sterilization, oil-bearing fruits are pulped (digested) before mechanical pressing often in a screw press. Palm kernels are removed from pressed cakes and further processed for oil. Animal tissues are reduced in size before rendering by wet or dry processes. After autoclaving, tissues of fish are pressed and the oil/water suspension is passed through centrifuges to separate the oil. 01 Degumming with water to remove the easily hydratable phospholipids and metals. Addition of a small amount of phosphoric or citric acid to convert the remaining 02 non-hydralable phospholipids (Ca, Mg salts) into hydratable phospholipids. Neutralising of the free fatty acids with a slight excess of sodium hydroxide 03 solution, followed by the washing out of soaps and hydrated phospholipids. Bleaching with natural or acid-activated clay minerals to adsorb colouring OIL REFINING 04 components and to decompose hydroperoxides. (ALKALINE REFINING METHOD) Deodorising to remove volatile components, mainly aldehydes and ketones, with low threshold values for detection by taste or smell. Deodorisation is essentially a 05 steam distillation process carried out at low pressures (2-6 mbar) and elevated temperatures (180-220°C). MARKET TRENDS, ISSUES, AND DRIVERS As of 2016, the Philippines are one of the largest exporters of coconuts and coconut oil in the world. However, coconut oil has now become a more export-oriented commodity and is used less for domestic consumption purposes. It is gradually being replaced by palm oil in recent years. The cooking oil market in the Philippines has increased on account of an increase in food demand by a growing number of households and expanding health-conscious middle class in the country. The Philippines Cooking Oil Market is traditionally dominated by the unorganized sector on account of providing the cooking oil at a lower cost than the organized players in the Industry. The major organized players include Minola, Golden Fiesta, Baguio, Marca Leon, and others. INNOVATIONS AND RESEARCHES Many studies have directed efforts to the development of vegetable oils purification, deacidification, discoloration, and solvent recovery methods to reduce costs and provide alternative economically sustainable processes. The viability of separating the oil from the hexane using solvent-resistant NF membranes arises because of their unique characteristics, properties, and other advantages when compared to the traditional separation techniques. One of the advantages is that the separation process can be conducted at room temperature, and it is therefore suitable for heat-sensitive products and can be used in almost all oil processing stages, as in the diagram. Bioenergy is energy derived from biofuels. Biofuels are fuels produced directly or indirectly from organic BIOFUELS material – biomass – including plant materials and animal waste. Overall, bioenergy covers approximately 10% of the total world energy demand. Traditional unprocessed biomass such as fuelwood, charcoal and animal dung accounts for most of this and represents the main source of energy for a large number of people in developing countries who use it mainly for cooking and heating. More advanced and efficient conversion technologies now allow the extraction of biofuels from materials such as wood, crops and waste material. Biofuels can be solid, gaseous or liquid, even though the term is often used in the literature in a narrow sense to refer only to liquid biofuels for transport. SOURCES Biofuels may be derived from agricultural crops, including conventional food plants or from special energy crops. Biofuels may also be derived from forestry, agricultural or fishery products or municipal wastes, as well as from agro-industry, food industry and food service by-products and wastes. Biofuels generally come from a few common sources. The concept lies in the fact the energy contained in raw materials, such as plants, originally came from the sun. Photosynthesis stores energy in the plants’ cells, which is present in the following materials: Sugar cane, sugar beet, and corn, maize, and other starches can be 01 Sugar Crops fermented, which yields ethanol. Soybean, oil palm, or even algae can be burned. To produce power, some 02 Natural Plant Oils diesel engines burn of these. You can also blend them with petroleum- based fuels. They often convert these into ethanol, methanol, and other liquid biofuels. 03 Wood/byproducts They also form wood gas. You can use firewood as a solid fuel. If a furnace supports it, they use 04 Burned Wood chipped wood as a fuel-based biomass KINDS OF BIOFUELS 01 02 Primary Biofuels Secondary Biofuels such as fuelwood, wood chips and pellets, organic result from processing of biomass and include materials are used in an unprocessed form, primarily liquid biofuels such as ethanol and biodiesel that for heating, cooking or electricity production. can be used in vehicles and industrial processes. TYPES OF LIQUID BIOFUELS FOR TRANSPORT 01 Ethanol Ethanol is a type of alcohol that can be produced using any feedstock containing significant amounts of sugar, such as sugar cane or sugar beet, or starch, such as maize and wheat. Sugar can be directly fermented to alcohol, while starch first needs to be converted to sugar. The fermentation process is similar to that used to make wine or beer, and pure ethanol is obtained by distillation. A liter of ethanol contains approximately two thirds of the energy provided by a liter of petrol. However, when mixed with petrol, it improves the combustion performance and lowers the emissions of carbon monoxide and sulphur oxide. TYPES OF LIQUID BIOFUELS FOR TRANSPORT 02 Biodiesel Biodiesel is produced, mainly in the European Union, by combining vegetable oil or animal fat with an alcohol. Biodiesel can be blended with traditional diesel fuel or burned in its pure form in compression ignition engines. Its energy content is somewhat less than that of diesel (88 to 95%). Biodiesel can be derived from a wide range of oils, including rapeseed, soybean, palm, coconut or jatropha oils and therefore the resulting fuels can display a greater variety of physical properties than ethanol. Generations of Biofuels First Generation Biofuels 01 are produced through conventional technology with sugar, starch, vegetable oil, or animal fats as sources. Second Generation Biofuels are produced from non-food crops or portions of food 02 crops that are not edible and considered as wastes, e.g. stems, husks, wood chips, and fruit skins and peeling. Third Generation Biofuels are those that are produced from algae. Production of 03 oilgae or algae fuel involves fermentation of the algae carbohydrate. Second and third generation biofuels are also called advanced biofuels. HIGH TEMPERATURE DECONSTRUCTION Pyrolysis 01 which is the thermal/chemical decomposition of feedstock without oxygen. The outcome is a bio-oil with hydrocarbons. There are more oxygenated compounds per unit than in petroleum crude oils. Before it can be made into a fuel or processed in a refinery, it must upgrade the intermediate. Hydrothermal Liquefaction 02 produces a bio-oil by adding heat and pressure to a wet feedstock slurry. After you treat it with water, it is further processed in a reactor. Thermal Deconstruction 03 is also accomplished with gasification, which occurs at temperatures above 700°C. You can add oxygen carrier or steam before you clean and condition the gas. LOW TEMPERATURE DECONSTRUCTION To facilitate the conversion process, you can use enzymes and other catalysts, such as heat. The carbohydrate material converts into an intermediate sugar compound. They can then ferment the building blocks. It’s also possible to chemically catalyze them. The process involves pretreatment, where you prepare the feedstock for hydrolysis using mechanical or chemical processing methods. They break down this material into soluble and insoluble components. This exposes the sugar polymers. Hydrolysis further breaks down the polymers. This forms molecules that are used as fuels or building blocks. PRODUCTION STAGES 01 05 03 02 04 06 Filtration Heating/Mixing They filter the oil, which Titration The results mixture is eliminates all food heated and mixed with particles. It is easier to A method of chemical care. filter warmer liquids. Water Removal analysis to determine Sodium Methoxide Settling/Separation You can do this with a the concentration of Preparation coffee filter. The reactions are the analyte present. It You mix methanol, at a As the mixture cools, faster when you helps determine how quantity of around 20 the biofuel will float on remove water. You can much lye is needed. percent of the top. You drain the accomplish this by vegetable oil used, with leftover glycerin and boiling the mixture at sodium hydroxide. use the pure biofuel. about 100°C. BIOFUELS AS A SOURCE OF RENEWABLE ENERGY Bioenergy is one of many diverse resources available to help meet our demand for energy. It is a form of renewable energy that is derived from recently living organic materials known as biomass, which can be used to produce transportation fuels, heat, electricity, and products. Solid, liquid, or gaseous fuels that are produced from biomass are called biofuels. They are renewable and are good substitutes to fossil fuels. Most biofuels available in the market today are made from plants. They are often used as transportation fuels. ADVANTAGES OF BIOFUELS It reduce vehicle emission which makes it eco-friendly. It is made from renewable sources and can be prepared locally. Increases engine performance because it has higher cetane numbers as compared to Petro diesel. It has excellent lubricity. Increased safety in storage and transport because the fuel is nontoxic and biodegradable (Storage, high flash point) Production of bio diesel in India will reduce dependence on foreign suppliers, thus helpful in price stability. Reduction of greenhouse gases at least by 3.3 kg CO2 equivalent per kg of biodiesel. Any questions? THANK YOU! FERTILIZER INDUSTRY What is Fertilizer? Fertilizer is a natural or artificial substance containing the chemical elements that improve growth and productiveness of plants They enhance the natural fertility of the soil or replace the chemical elements taken from the soil by previous crops Plant nutrients are building blocks of crop material. Without nutrients crops cannot grow. Three primary nutrients are nitrogen, phosphorus, and potassium. THREE MAIN NUTRIENTS FOUND IN FERTILIZER Phosphorus Nitrogen is essential for proper the primary component root formation and aids of proteins, is crucial N P the plant's resistance to for development and drought. Additionally, it is growth plant life. The necessary for plant availability of nitrogen growth and development. affects a plant's development, vigor, color, and yield. Potassium is essential to crop photosynthesis. Potassium aids with crop improvement quality and drought, disease, K and lodging resistance in crops. ADDITIONAL NUTRIENTS FOUND IN FERTILIZER Magnesium Sulfur is needed for is particularly crucial photosynthesis, during the early S Mg converting light into phases of growth, to chemical energy for manufacture critical nutritional purposes proteins, oils, and amino acids Calcium especially crucial for fruit and vegetable productivity, quality, Ca and shelf life. MINERAL FERTILIZER When crops are harvested, a significant amount of the nutrients that the crops absorbed from the soil are removed from the field. While crop leftovers and other organic matter can restore some nutrients to the soil, they cannot, by themselves, guarantee optimal fertilization and crop yields over time. In order to maximize crop output and quality while reducing negative environmental effects, mineral fertilizers can offer an ideal nutrient balance that is matched to the needs of the particular crop, soil, and climate conditions. FERTILIZER TO THE ENVIRONMENT ADVANTAGES DISADVANTAGES It increases crop yield and Weeds thrive alongside improved improves poor quality land crop growth. Sprays of herbicide Manure enhances soil are therefore also necessary. quality, recycles nitrogen, Fish perish when too much and adds vital microbes. nitrogen from fertilizers enters Improved pasture helps water systems. animals gain weight more Farmers in less developed quickly. nations, in particular, risk Fertilizers can aid in the harming their health by using reclamation of marshes fertilizers, pesticides, and for pasture once it has herbicides improperly. been drained. When artificial fertilizers are used without organic inputs, soil structure is not improved. FERTILIZER INDUSTRY The fertilizer sector is made up of businesses that cover the full supply chain, from manufacturing to shipping to sale, and they all collaborate to provide farmers with fertilizer in a timely, efficient, and sustainable manner. The fertilizer industry played a significant role in the centrally planned economy and is still a vital component of modern agricultural production since it supplies the necessary mineral elements for healthy crop growth and bountiful harvests. FERTILIZER PRODUCTION NITROGEN-BASED An important sector of the global economy, nitrogen fertilizers account for almost 100 million tons of varied goods annually. The most popular nitrogen fertilizers include a variety of liquid and solid chemicals, the most common of which are urea, ammonia, and ammonium nitrate. Using the Haber process, ammonia is created by combining nitrogen from the air and hydrogen from natural gas at high pressure and temperature (200–300 bars and roughly 450°C). Anhydrous ammonia is either chilled or held as a liquid under pressure. It is frequently transformed into other sorts of fertilizers for handling convenience. FERTILIZER PRODUCTION NITROGEN-BASED Ammonia and air are combined to create nitric oxide gas, which is then absorbed in water to produce nitric acid. The resulting concentrated nitric acid is then mixed with ammonia gas in a tank at temperatures between 100 and 180°C to produce a neutralization reaction. Ammonium nitrate, an important nitrogen-based fertilizer, is produced by this process. Urea, another nitrogen-based fertilizer, is created when ammonia and carbon dioxide react under intense pressure. Both urea and ammonium nitrate can be further concentrated and transformed into solid forms like granules or prills. Additionally, liquid urea ammonium nitrate (UAN) can be produced by adding urea to an ammonium nitrate solution in a different chemical step. FERTILIZER PRODUCTION NITROGEN-BASED FERTILIZER PRODUCTION PHOSPHORUS-BASED Most commercially available phosphate fertilizers are produced using phosphate rock (PR) as their main raw ingredient. Due to their large reserves of top-notch phosphate rock, Morocco, China, and the United States are major players in the phosphate sector. In order to extract the ground phosphate rock, mines must transport it to recovery units where sand, clay, and other impurities are sorted and eliminated because it is easier to move materials during these operations while they are moist and less dust is produced. FERTILIZER PRODUCTION PHOSPHORUS-BASED The wet-process reaction of phosphate rock (PR) and sulfuric acid yields phosphoric acid (40–55%). It is employed in the production of solid and liquid fertilizers such as (single and triple superphosphates) SSP, TSP, (mono and diammonium phosphate) MAP, and DAP. The Dorr-Oliver slurry granulation method is used to create TSP, which yields GTSP with superior storage qualities. Because of their affordability and nutritious content, ammoniated phosphates (MAP, DAP) are preferred. They are made by combining weak phosphoric acid with ammonia. FERTILIZER PRODUCTION PHOSPHORUS-BASED FERTILIZER PRODUCTION POTASSIUM-BASED Potassium, which is obtained from mined ores, is a crucial nutrient for plants and crops. It takes a variety of chemical procedures to transform potash rock into plant- friendly forms including potassium chloride, sulfate, and nitrate for the production of potassium-based fertilizers. A mixture of potassium minerals used to make potassium fertilizers is referred to as potash. Since it dissolves in water, purifying procedures are needed to get rid of contaminants like sodium chloride (common salt). When describing the potassium content of a substance, potassium chloride, potassium sulfate, potassium carbonate, or potassium nitrate are the main ingredients in potash fertilizers. FERTILIZER PRODUCTION POTASSIUM-BASED N-P-K FERTILIZERS NITROGEN COMPONENT One essential nutrient for plant growth and development is nitrogen. It is crucial for maintaining plant health and the nutritional content of harvested crops since it is crucial for the production of proteins, which make up a sizeable component of the tissues of living things. Through the introduction of natural gas and steam into a vessel and the subsequent combustion of the gas and steam, a method for producing ammonia from air has been created. In the resultant mixture, carbon dioxide, nitrogen, and hydrogen predominate. Applying an electric current to the system results in the generation of ammonia and the elimination of carbon dioxide. Ammonia production is accelerated and made more effective by the use of catalysts like magnetite (Fe3O4). N-P-K FERTILIZERS NITROGEN COMPONENT Ammonia can be used as fertilizer, but it is frequently transformed into other materials for simpler management. Ammonia and air are combined in a tank to create nitric acid, which is then created via a reaction that uses a catalyst to change ammonia into nitric oxide. Nitric acid is then produced by reacting the nitric oxide with water. Ammonium nitrate, which has a high nitrogen content and is produced using nitric acid and ammonia, is a desirable fertilizer component. In a tank, the two compounds are combined, starting a neutralization reaction that produces ammonium nitrate. This substance can be kept until it is ground up and combined with other fertilizer ingredients. N-P-K FERTILIZERS PHOSPHORUS COMPONENT Phosphorus is an essential component of a plant's regular growth and development as well as the process of photosynthesis, which uses energy. The phosphorus in commercial fertilizers comes from phosphate rock. Phosphoric acid is created by treating phosphate rock with sulfuric acid in order to remove the phosphorus therein. In order to produce triple superphosphate, a good solid supply of phosphorus, a portion of this phosphoric acid is further reacted with sulfuric acid and nitric acid. A portion of the phosphoric acid is additionally mixed with ammonia in a different tank to create ammonium phosphate, another efficient main fertilizer. N-P-K FERTILIZERS POTASSIUM COMPONENT Commercial fertilizers contain the essential component potassium, which helps plants resist illness and improves crop yields and quality. By bolstering their root systems and reducing wilting, it also assists to protect plants from harmful weather conditions like cold or dry spells. Potassium chloride is frequently added in high quantities throughout the fertilizer manufacturing process. Manufacturers transform it into granules to make it easier to mix with other fertilizer ingredients. The next stage of mixing is made easier by this phase. N-P-K FERTILIZERS GRANULATING AND BLENDING The substances (ammonium nitrate, potassium chloride, ammonium phosphate, and triple superphosphate) are granulated and blended to create highly useful fertilizer. The solid materials are rotated in a drum during the granulation process to create tiny spherical shapes. The particles are then screened, dried, and coated with inert dust to stop moisture from re-accumulating. The various particles are then combined using a spinning mixing drum in the proper ratios. Finally, a conveyor belt carries the combined fertilizer to the bagging machine for packaging. N-P-K FERTILIZERS N-P-K FERTILIZERS BAGGING Large quantities of fertilizer are often given to farmers. Fertilizer is initially delivered into a sizable hopper before being used to fill these bags. A suitable quantity is discharged into a bag that is kept open by a clamping device from the hopper. Better packing is made possible by the bag's vibration-generating surface. Once the bag has been fully filled, it is carried upright to a machine where it is sealed shut. The bag is then transported to a palletizer, where it is stacked with other bags in preparation for delivery to distributors and ultimately to farmers. N-P-K FERTILIZERS BAGGING N-P-K FERTILIZERS QUALITY CONTROL Manufacturers keep an eye on the product at every stage of production to assure the quality of the fertilizer that is produced. To demonstrate that they adhere to the previously established requirements, both the raw materials and the completed goods are put through a battery of physical and chemical testing. pH, appearance, density, and melting point are just a few of the qualities put to the test. Since the manufacture of fertilizer is subject to government regulation, samples are subjected to composition analysis tests to ascertain the amount of total nitrogen, phosphate, and other components affecting the chemical composition. Depending on the particulars of the content of the fertilizer, many further tests are also carried out. ECONOMIC IMPACT DRIVERS DEMAND Consumption of fertilizer is influenced by economic and population growth. Both the US and China are significant agricultural producers, with the US excelling in the production of rice and soybeans. Global meat consumption is rising, especially for poultry and pork, which necessitates large-scale feed production. Fertilizers containing nitrogen, which make up 20– 30% of overall usage, are necessary to meet this demand. As low prices can impede grain production, resulting in supply shortages and price rises, maintaining production to meet rising demand is essential. ECONOMIC IMPACT DRIVERS DEMAND In 2017, less than 20% of the entire cost of producing maize was made up of fertilizer expenses, which have been falling over the previous three years. The relative share for other key crops is lower, ranging from 6% for soybeans to 11% for wheat. ECONOMIC IMPACT SEASONALITY OF FERTILIZER Application of fertilizer is intimately related to the seasonality of crop planting and harvesting, which differs greatly among locations as a result of weather. When seeds are sown in the northern hemisphere, fertilizer is often sprayed in the first part of the year. The second half of the year is when fertilizer is applied in the southern hemisphere. Winter wheat, which is planted in the second half of the year but fertilized in the spring, is an exception. Some nations harvest specific crops twice a year, especially those in the southern hemisphere like India and Brazil. ECONOMIC IMPACT ECONOMIC IMPACT PRODUCTION ECONOMICS Raw materials, electricity, and freight make up around 84% of the operational cash costs of the fertilizer sector. These purchases can be cancelled in large part with little warning, which lessens the cost impact of delivery delays. In response to a drop in supplies or to take advantage of more affordable imported ammonia, Yara's operations can be shut down quickly and inexpensively. APPLICATION AGRICULTURE By using fertilizers, crop yields can be increased and soil quality can be raised. By supplying important macro-minerals like calcium and phosphorus, animal feeds, such as those containing feed phosphates, serve a critical role in fostering animal growth. APPLICATION ENVIRONMENT Environmentally friendly applications have emerged as a result of the fertilizer industry's ongoing expansion and innovation. To lower NOx emissions and meet environmental regulations, selective catalytic reduction systems use ammonia and urea as reducing agents. Additionally, by carefully regulating the nitrate dosage, calcium nitrate is utilized to eliminate hydrogen sulfide from wastewater. APPLICATION INDUSTRIAL MANUFACTURING In addition to being used in agriculture and the environment, fertilizers can also be used as raw materials in a variety of businesses. In the glue business, for instance, urea is used to make urea-formaldehyde resins and adhesives. Ammonium nitrates, which are made from nitric acid, are used to make civil explosives. To create synthetic fibers, ammonia is used in the textile industry. In making concrete, calcium nitrate acts as an anti- freezing ingredient. It can also be used as a catalyst for making rubber gloves. Any questions? THANK YOU! BATANGAS STATE UNIVERSITY - ALANGILAN COLLEGE OF ENGINEERING DEPARTMENT OF CHEMICAL ENGINEERING ALANGILAN, BATANGAS CITY PAINTS and PIGMENTS INDUSTRY Presentation by Group 1 MEMBERS Alva, Amazona, Aquino, John Jovelle Hyah V. Jan Daryl M. Reinnier B. Arandia, Arenas, Bagadiong, Dehsery G. Hazel Ann A. Jireh Mae J. Content of the Presentation 01 INTRODUCTION TO THE INDUSTRY 02 COMPONENTS OF PAINTS AND PIGMENTS 03 TYPES AND PROPERTIES OF PAINTS 04 MANUFACTURING PROCESS AND OPERATIONS 05 USES AND APPLICATIONS 06 IMPACTS IN HEALTH AND ENVIRONMENT 07 INDUSTRIAL COMPANIES IN THE PHILIPPINES Paints and Pigment Industry The paint and pigments industry is responsible for producing a wide range of decorative and protective coatings. Paint is defined as a unique homogeneous mixture composed of 3 major ingredients namely Binder, Pigment, Solvents and Additives which when applied on the surface forms a thin layer that creates a solid dry adherent film or coating. It is formulated in a well-balanced proportion of its ingredients to achieve the intended color, finish and performance characteristics. Its production involves the usage of a binder to disperse the pigment, dissolved in a particular solvent mixed with required additives. Paints and Pigment Industry Painting is one of the earliest arts which emerged in the prehistoric period. Early paint solutions utiliz ed are said to be created from egg yolk, water, and oil which is colored with charcoal, plant dye, and soil. The 18th century arises the disovery of linseed oil and z inc ox ide and evolved significantly in the 20th century due to the introduction of synthetic polymers and pigments as well as water based paints which leads to the utiliz ed formulation of paint in modern period. Paints offers several functions which centers on material protection from environmental factors and from corrosion as well as surface appearance and property enhancement. BINDER Binder is a polymer (resins) that forms a continuous film on the substrate surface and serves as the main component of paint. It is used to firmly hold the pigments scattered throughout the coating and attach it into the surface. The type and quantity of binder influences properties such as exterior durability, stain resistance, adhesion, and toughness. BINDER Commonly used binder: Acrylic resins- This comes in water-based or solvent-based forms. Solvent-based Epoxy resins- This provides adhesion and paints are used as protective coatings. resistance to chemicals. It is best used for Water-borne emulsion paints are used as a interior coatings and exterior primers decorative paint for inside and outside of buildings Alkyd resins- This are made by combining Polyurethane- it is a liquid plastiic film fatty acids and polyols, with polybasic acids known for its durability and attractive causing condensation polymerization. The glossy finish resulting polymer is used as decorative gloss paints PIGMENTS Pigments are present in either organic or inorganic powder which gives the paint its color and opacity. The pigment opacity depends on two properties namely: Refractive Index (RI) and Particle Siz e. The concentration level of pigments influence the paint's appearance (gloss level) and properties such as film flow, and protective abilities. Pigments can be classified into two main categories namely Prime or Hiding Pigments and Inert or Ex tender Pigments. PIGMENTS Commonly used pigments: Titanium Dioxide (TiO2)- Carbon Black- Ultramarine Blue- It is a synthetic white It is a dark pigment It is a natural pigment d inorganic pigment that produced by combustion known for its color stability exists in two crystal of organic materials. It is as it resists fading and forms: Anatase (2.52, RI), used in automotive remains intact at high Rutile (2.76, RI), Enhance industry due to its temperatures. opacity giving gloss to reinforcing properties paints. and deep black hue. PIGMENTS Fillers and extenders are pigments that do not impart color but enhance adhesion and thickness, increase paint volume, and improve durability or abrasion resistance and texture of coating. Commonly used fillers and extenders: Limestone (calcium Baryte (BaSO4) - Kaoline Clay - carbonate, CaCO3) - This reinforces paint This is used to enhance This extend expensive components matte finishes pigments SOLVENT Solvents either water or an organic compound, act as a dispersing medium that suspends the binder, pigment, and additives in either true molecular solutions or colloidal dispersion such as emulsions until the paint sets. In water-borne paints, water serves as the solvent while in oil-based paint, the solvent is an organic material such as a paint thinner. This balances the viscosity, dry time, and spreading rate of paints and assists in achieving the gloss level and desired thick ness of the dry film. SOLVENT Solvents used as carrier: White spirits (mineral Ketones- Xylene - turpentine spirits)- It is a colorless liquid It is an aromatic solvent This consist of a with a carbonyl group that doesn't mix with mix ture of saturated (C=O) bonded to two water but blends with aliphatic and alicyclic other carbon atoms other solvents. It has a hydrocarbons used having ox ygen in their strong solubility and widely as a solvent and molecules. Methy Ethyl moderate evaporation rate thinner. Ketone ( MEK) is an making it utilized for ex ample of this solvent. various resins as well as used for both air dry and baked coatings ADDITIVES Additives are different chemical substances added in small amounts ranging from 0.001% & ≤ 5% to modify or enhance the paint properties such as color opacity, pigment dispersion, and stability. ADDITIVES Additives can be: Driers Plasticisers UV stabilizers Fungicides, Biocides and Anti-skinning agents Insecticides Adhesion promoters Flow control agents Corrosion inhibitor Anti-foaming Agents ( Defoamers) Texturizers Wetting Agents (Emulsifiers) OIL PAINT Oil painting is a type of painting produced using oil-based paints. Oil painting involves using pigments that use a medium of drying oil as the binder and painting with them on a canvas EMULSION PAINT Unlike traditional oil paints, the majority of emulsions are water-based paints with fast-drying characteristics. It contains binding materials such as polyvinyl acetate, and synthetic resins and etc. ENAMEL PAINT It contains white lead, oil, petroleum spirit and resinous material. The surface provided by it resists acids, alk alies and water very well. BITUMINOUS PAINT This type of paint is manufactured by dissolving asphalt or vegetable bitumen in oil or petroleum. It is black in color. It is used for painting iron work s under water. ALUMINIUM PAINT Aluminium paint is a type of paint coating that is made by mixing aluminium particles or flakes with oil/spirit varnish. The type of varnish can be used as per the requirement since spirit varnish leads to a shorter drying period. ANTI-CORROSIVE PAINT It consists essentially of oil, a strong dier, lead or zinc chrome and finely ground sand. It is cheap and resists corrosion well. It is black in color. SYNTHETIC RUBBER PAINT This paint is prepared from resins. It dries quickly and is little affected by weather and sunlight. It resists chemical attack well. This paint may be applied even on fresh concrete. Its cost is moderate and it can be applied easily. CEMENT PAINT It is available in powder form. It consists of white cement, pigment and other additives. It is durable and ex hibits ex cellent decorative appearance. It should be applied on rough surfaces rather than on smooth surfaces. OPACITY OR HIDING POWER This refers to the paint’s ability to obscure LIGHTFASTNESS the surface beneath it with one single coat. The lightfastness of a paint or pigment describes how well it withstands degradation from UV lightness. It is also the measurement of how chemically stable a material is when exp osed to light. ADHESION This refers to how well the paint sticks to the surface it's applied to APPLICATION OR DRYING TIME This refers to how easily a paint can be applied to a surface and how long it take s to dry. COLOR TEMPERATURE Different pigments of the same hue can ex hibit varying color temperatures. This is one reason there are many different choices for the same color. SELECTION OF RAW MATERIALS GRINDING AND MILLING MIXING AND BLENDING QUALITY CONTROL AND TESTING SHIPPING PACKAGING ARCHITECTURAL PROTECTION AESTHETIC ENHANCEMENTS SURFACE PRESERVATION INDUSTRIAL CORROSION RESISTANCE HEAT RESISTANCE CHEMICAL RESISTANCE AUTOMOTIVE AUTOMOTIVE COATINGS CUSTOM COLOR & FINISHES MARINE ANTI-FOULING PAINTS PROTECTIVE COATINGS AERO SPACE THERMAL CONTROL WEIGHT REDUCTION AESTHETIC & FUNCTIONAL REQUIREMENTS CONSUMER GOODS & PACKAGING PRODUCT DIFFERENTIATION BRAND IDENTITY PROTECTION & PRESERVATION INTERACTIVE & SMART PACKAGING ART & CREATIVE FINE ARTS CRAFTS & HOBBIES INNOVATION & CREATIVITY EDUCATION & COMMUNITY ENGAGEMENT AIR POLLUTION Paints have a detrimental effect on the atmosphere because of Volatile Organic Compounds (VOCs) and certain air pollutants. Air pollution happens through off-gassing which entails the release of air pollutants, contaminating the surrounding air. WATER POLLUTION When paint products are washed away from buildings during rainfall or when the painting equipment is washed, some of it can contaminate nearby bodies of water which can cause contamination. Contamination in water occurs through a runoff. SOIL POLLUTION Soil can be contaminated once harmful chemicals and heavy metals from paints get in contact with the soil. The soil can absorb harmful pollutants through leaching which can cause plant contamination and potential groundwater pollution. HEALTH IMPACTS Paints can cause severe health impacts if they come in contact with skin, eyes, nose, or throat through paint fumes. These fumes release VOCs, which are chemical pollutants emitted as gasses, which negatively affect one's health. Thank You Presentation by GROUP 1 IRON AND STEEL INDUSTRY Group 5 - ChE 3304 Group 5 Members Masangcay, Patricia Meraña, Ylaine Morales, Arabella Grace Ocampo, Jazmin Padua, Chris Nolan Paranaque, Trixie Mae Pelagio, Leelord TABLE OF CONTENTS 01 INTRODUCTION 05 WASTE CHARACTERISTICS AND TREATMENT 02 RAW MATERIALS AND PROPERTIES 06 RECYCLING AND DISPOSAL 03 MANUFACTURING PROCESSES AND 07 PRODUCTS’ APPLICATION OPERATION TYPES AND 04 CLASSIFICATION 01 INTRODUCTION IRON AND STEEL INDUSTRY Iron is the 4th most abundant element on Earth, and makes up more than 5% of the earth’s crust. Iron exists naturally in iron ore (sometimes called ironstone). Since iron has a strong affinity for oxygen, iron ore is an oxide of iron; it also contains varying quantities of other elements such as silicon, sulfur, manganese, and phosphorus. IRON AND STEEL HISTORY EARLY ORIGINS The use of iron dates back to around 1200 BC. The earliest evidence of iron being used as a material dates back to 3500 BCE in Egypt where beads of iron were taken from a meteor found. Tutankhamun was said to be buried with a dagger made of meteor iron. IRON AND STEEL HISTORY EARLY ORIGINS Meteoric Iron was also used as tools in pre-contact North America where the Thule people of Greenland began utilizing the pieces of the Cape York meteorite as edged tools around the year 1000. IRON AGE EARLY IRON SMELTING Between 3000 BCE and 2000 BCE, increasing numbers of smelted iron objects (distinguished from meteoric iron by their lack of nickel) appeared in Anatolia, Egypt, and Mesopotamia. The oldest known samples of smelted iron are small lumps found at copper-smelting sites on the Sinai Peninsula, dated to about 3000 BCE. IRON AGE TRANSITION FROM BRONZE TO IRON Iron did not replace bronze immediately as the chief metal for weapons and tools for several centuries due to higher fuel and labor requirements and initially inferior quality. Between 1200 and 1000 BCE, iron tools and weapons began to displace bronze ones throughout the Near East. IRON AGE The Iron Age commenced when iron began to replace bronze in tools and weapons due to its superior hardness, durability, and ability to hold a sharp edge. Iron remained the primary material for tools and weapons for over three thousand years until steel began to replace it after 1870. Early Iron Smelting/Production Techniques Bloomery/Smelting Process Iron smelting at this time was based on the bloomery, a furnace where bellows forced air through a pile of iron ore and burning charcoal. The iron was collected as a spongy mass, or bloom, which was then reheated and repeatedly beaten to remove slag, resulting in wrought iron. Wrought Iron Production Wrought iron was the most commonly produced metal through most of the Iron Age. Primitive blacksmiths hammered the pasty mass of bloom iron to remove impurities and compact the metal, producing wrought iron, which contains a small amount of carbon (0.02 to 0.08 percent). Early Iron Smelting/Production Techniques Cast Iron Production At very high temperatures, iron absorbs more carbon and melts, resulting in cast iron (3 to 4.5 percent carbon), which is hard but brittle. Blast Furnaces By the late Middle Ages, originating in China around the 4th century BCE and adopted by European ironmakers, the blast furnace, was developed which allowed for the efficient production of cast iron, known as pig iron. It is a tall chimney-like structure in which combustion is intensified by a blast of air pumped through alternating layers of charcoal, flux, and iron ore. Refining Processes Refining Cast Iron Methods to transform cast pig iron into wrought iron included using a finery or a puddling furnace. Puddling Furnace Developed by Henry Cort in 1784, this process involved stirring molten metal to oxidize carbon, producing wrought iron. Coke in Smelting In the 1700s, Abraham Darby discovered that coke could replace charcoal in smelting, alleviating deforestation issues. STEEL PRODUCTION Steel is an alloy with a carbon content of 0.2 to 1.5 percent. It is harder than wrought iron but not as brittle as cast iron. Early Steel Production The earliest signs of true steel production are from the 13th century BC in modern-day Turkey. Blister steel, one of the earliest forms of steel, began production aroun Germany and England in the 17th century and was produced by cementation, a process of increasing the carbon content in molten pig iron. Before 1856, steel was expensive and difficult to manufacture, primarily produced through cementation and crucible methods. Crucible Steel - Benjamin Huntsman developed crucible steel in the 1740s, offering high-quality but expensive steel. STEEL PRODUCTION Steel is an alloy with a carbon content of 0.2 to 1.5 percent. It is harder than wrought iron but not as brittle as cast iron. The Bessemer Process In 1856, Sir Henry Bessemer invented the Bessemer process, which allowed for the mass production of steel by blowing air through molten pig iron to reduce carbon content. Robert Mushet improved the process by adding spiegeleisen to adjust the carbon and oxygen levels, making steel production more efficient. The Bessemer process was later improved by Sidney Gilchrist Thomas, which involved the addition of limestone to remove phosphorus, making steel production from various iron ores possible. STEEL PRODUCTION Steel is an alloy with a carbon content of 0.2 to 1.5 percent. It is harder than wrought iron but not as brittle as cast iron. Open-Hearth Process Developed in the 1860s by Karl Wilhelm Siemens, this process allowed for precise control of steel composition and larger batch production. The open-hearth process could produce high-quality steel and recycle scrap metal, eventually replacing the Bessemer process by 1900 and was replaced by the basic oxygen process, a modification of the Bessemer process, after 1960. STEEL PRODUCTION 20th Century Advancements Electric Arc Furnaces (EAFs) - use electricity to melt recycled steel scrap, providing a more energy-efficient and environmentally friendly alternative to traditional blast furnaces. Linz-Donawitz (LD) process, or basic oxygen steelmaking - oxygen is blown through molten pig iron to reduce impurities and control alloy composition, resulting in high-quality steel. STEEL PRODUCTION Contemporary Techniques Development of direct reduced iron (DRI) processes, such as Midrex and HYL technologies, which use natural gas or hydrogen to directly reduce iron ore, producing high-purity iron for various applications, including steelmaking. Advancements in smelting technologies, including the use of plasma and novel reactor designs, aimed at enhancing energy efficiency and reducing environmental impact. Capture and utilization of by-products like carbon dioxide and waste heat, contributing to more sustainable ironmaking processes