computed tomography equipment techniques 7.pdf
Document Details
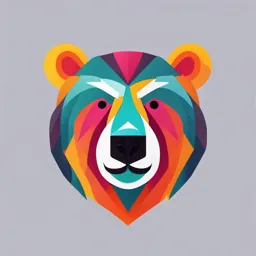
Uploaded by FruitfulLandArt
جامعة التقنية الوسطى
Tags
Full Transcript
Middle Technical University (MTU) College of Health and Medical Techniques -Baghdad Radiological Techniques Department Computed Tomography EquipmentsTechniques Second stage/ 2nd coarse Title: Collimation, Filtration Detector: Detector Characteristics & types Name of the instructor: Lec. Dr. Lamyaa F...
Middle Technical University (MTU) College of Health and Medical Techniques -Baghdad Radiological Techniques Department Computed Tomography EquipmentsTechniques Second stage/ 2nd coarse Title: Collimation, Filtration Detector: Detector Characteristics & types Name of the instructor: Lec. Dr. Lamyaa Fadhil Abdul Hussein Target population: Students of second class 55 Scientific Content: Filtration In clinical CT, a special filter must be used, it serves a dual purpose: 1. Filtration removes long-wavelength x rays because they do not play a role in CT image formation; instead they contribute to patient dose. As a result of filtration, the mean energy of the beam increases and the beam becomes arder. Recall that the total filtration is equal to the sum of the inherent filtration and the added filtration. In CT the inherent filtration has a thickness of about 3 mm Al-equivalent. The added filtration, on the other hand, consists of filters that are flat or shaped filters made of copper sheets, for example, the thickness of which can range from 0.1 to 0.4 mm. Fig. 1: Attenuation of radiation through a circular object. The beam becomes more penetrating (harder) in section 3 because of differences in attenuation in sections 1 and 2. The heavier arrows indicate less attenuation and m ore penetrating rays. 56 2. Filtration shapes the energy distribution across the radiation beam to produce uniform beam hardening when x rays pass through the filter and the object. In Figure (1), the attenuation differs in sections 1, 2, and 3 and the penetration increases in sections 2 and 3. This results from the absorption of the soft radiation in sections 1 and 2, which is referred to as hardening of the beam. Because the detector system does not respond to beamSpecial shaped filters conform to the shape of the object and are positioned between the x-ray tube and the patient (Fig. 2). These filters are called shaped filters, such as the bilateral symmetry with a thickness that increases with the distance from the center. Bowtie filters compensate for the difference in beam path length through the axial plane of the object such that a more uniform fluence can be delivered to the detector. Fig.2: Two types of beam-shaping filters for use in CT. These filters attenuate the beam so that a more uniform (monochromatic) beam falls onto the detectors. 57 Collimation Collimation is required during CT imaging for precisely the same reasons as in conventional radiography. Proper collimation reduces patient radiation dose by restricting the beam to the anatomy of interest only. The basic collimation scheme in CT is shown in Fig. 3, with adjustable prepatient and postpatient (predetector collimators). Fig.3: Collimation scheme typical of CT scanning In general, a set of collimator sections is carefully arranged to shape the beam. Both proximal and distal collimators are arranged to ensure a constant beam width at the detector. Detector collimators can shape the beam and remove scattered radiation. Moreover, the collimator section at the distal end of the collimator assembly also helps define the thickness of the slice to be imaged. Various slice thicknesses are available depending on the type of scanner. 58 Adaptive Section Collimation The introduction of MSCT scanners posed some challenges with the design of the collimation scheme especially as the detectors become wider. The problems are related to what has been referred to as overscanning and overbeaming. Overbeaming relates to x-ray beams being slightly wider than the detector which means that patients are exposed over a small area without the signal being detected. Overscanning refers to exposure of the patient outside the imaged range which occurs for spiral CT with multi-row detectors at the start and the end of the scan These two problems result in increased dose to the patient. For example, overscanning may result in an increase of 5% to 30% in dose keeping the length of the scan in mind. The problems of overscanning and overbeaming can be solved using a technique called adaptive section collimation. With adaptive section collimation (see Fig. 4) parts of the x-ray beam exposing tissue outside of the volume to be imaged are blocked in the z-direction by dynamically adjusted collimators at the beginning and at the end of the CT scan FIG.4: Graphic illustration of the concepts of overbeaming, overranging,and adaptive section collimation technology during spiral scanning. 59 Detectors CT detectors capture the radiation beam from the patient and convert it into electrical signals, which are subsequently converted into binary coded information. Detector Characteristics Detectors exhibit several characteristics essential for CT image production affecting good image quality, such as: efficiency & Response time (speed of response). Efficiency refers to the ability to capture, absorb, and convert x-ray photons to electrical signals. CT detectors must possess high capture efficiency, absorption efficiency, and conversion efficiency. Three important factors contributing to the detector efficiency are: Capture efficiency refers to the efficiency with which the detectors can obtain photons transmitted from the patient; the size of the detector area facing the beam and distance between two detectors determines capture efficiency. Absorption efficiency refers to the number of photons absorbed by the detector and depends on the atomic number, physical density, size, and thickness of the detector face. Conversion efficiency refers to the ability to accurately convert the absorbed x-ray signal into an electrical signal. The Overall efficiency is the product of the three, and it generally lies between 0.45 and 0.85. A value of less than 1 indicates a nonideal detector system and results in a required increase in patient radiation dose if image quality is to be maintained. The term dose efficiency sometimes has been used to indicate overall efficiency. 60 The response time of the detector refers to the speed with which the detector can detect an x-ray event and recover to detect another event. Response times should be very short (i.e., microseconds). X-ray detectors used in CT systems must (a) have a high overall efficiency to minimize the patient radiation dose, have a large dynamic range, (b) be very stable with time, and (c) be insensitive to temperature variations within the gantry. Types The conversion of x-rays to electrical energy in a detector is based on two fundamental principles (Fig. 5). Scintillation detectors (luminescent materials) convert x-ray energy into light, after which the light is converted into electrical energy by a photodetector (Fig.5, A). Gas- ionization detectors, on the other hand, convert x-ray energy directly to electrical energy, (Fig. 5, B). Fig. 5: Two methods for converting x-ray photons into electrical energy. A, Scintillation crystal detection and conversion scheme. B, Conversion of x rays into electrical energy through gas ionization. 61 1. Scintillation detectors Are solid-state detectors that consist of a scintillation crystal coupled to a photodiode tube. When x rays fall onto the crystal, flashes of light, or scintillations, are produced. The light is then directed to the photomultiplier, or PM tube. As illustrated in Fig.6, the light from the crystal strikes the photocathode of the PM tube, which then releases electrons. These electrons cascade through a series of dynodes that are carefully arranged and maintained at different potentials to result in a small output signal. Fig. 6: Schematic representation of a scintillation detector based on the photomultiplier tube. In CT, scintillation detectors must exhibit a high light output, short primary decay time -output stability (time, temperature), compact packaging, and easy machining. 62 As a result, single crystals and polycrystalline ceramics have become popular scintillators for use in CT imaging. In the past, early scanners used sodium iodide crystals coupled to PM tubes. Because of afterglow problems and the limited dynamic range of sodium iodide, other crystals such as calcium fluoride and bismuth germanate were used in later scanners. Scintillation materials currently used with photo- diodes are cadmium tungstate (CdWO4) and a ceramic material made of high-purity, rare earth oxides based on doped rare earth compounds. 2. Gas-Ionization Detectors Gas-ionization detectors, which are based on the principle of ionization, were introduced in third- generation scanners. The basic configuration of a gas- ionization detector consists of a series of individual gas chambers, usually separated by tungsten plates carefully positioned to act as electron collection plates (Fig. 7). When x rays fall on the individual chambers, ionization of the gas (usually xenon) results and produces positive and negative ions. The positive ions migrate to the negatively charged plate, whereas the negative ions are attracted to the positively charged plate. This migration of ions causes a small signal current that varies directly with the number of photons absorbed. Fig. 7: Schematic representation of a Gas-ionization detectors. 63 The gas chambers are enclosed by a relatively thick ceramic substrate material because the xenon gas is pressurized to about 30 atmospheres to increase the number of gas molecules available for ionization. Xenon detectors have excellent stability and fast response times. However, their quantum detection efficiency (QDE) is less than that of solid-state detectors. As reported in the past, the QDE is 95% to 100% for crystal solid-state scintillation detectors and 94% to 98% for ceramic solid-state detectors, and it is only 50% to 60% for xenon gas detectors. It is important to note that with the introduction of MSCT scanners with their characteristic multirow detector arrays, gas-ionization detectors are not used anymore. MSCT scanners are all based on the use of solid-state detector arrays. Detector electronics (DAS) The DAS refers to the detector electronics positioned between the detector array and the computer (Fig. 8). Because the DAS is located between the detectors and the computer, it performs three major functions: (1) measuring the transmitted radiation beam, (2) encoding these measurements into binary data, and (3) transmitting the binary data to the computer. 64 Fig. 8: ( A ) Position of the data acquisition system in CT. (B) Essential components of the data acquisition system in CT. 65