Use of Composite Materials in Aircraft Construction PDF
Document Details
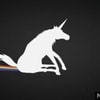
Uploaded by PleasedSugilite7751
Tags
Summary
This document discusses the use of composite materials in aircraft construction. It explores the different types of reinforcing fibers, such as fiberglass, aramid, carbon fiber, and ceramic, and their properties, advantages, and disadvantages. The text also covers the use of these materials in various aircraft components.
Full Transcript
Use of Composite Materials in Aircraft Construction Non-metallic structural materials played a key role in the early days of aviation. However, after the introduction of aluminium, non-metallic materials saw considerably less use. Today, aluminium is still the most widely used material in the constr...
Use of Composite Materials in Aircraft Construction Non-metallic structural materials played a key role in the early days of aviation. However, after the introduction of aluminium, non-metallic materials saw considerably less use. Today, aluminium is still the most widely used material in the construction and repair of aircraft. However, since its introduction, several new materials have come into use, many of which are spin-offs from the space program. These materials once again have the aviation industry taking a close look at non-metallic materials for use on aircraft, and they are becoming more common in construction of more aircraft components, even fuselages and wings. Composite Structure The term composite is used to describe two or more materials that are combined to form a structure that is much stronger than the individual components. Composite structures differ from metallic structures in several ways: excellent elastic properties, customisability in strength and stiffness, damage tolerance characteristics and sensitivity to environmental factors. Consequently, composites require a vastly different approach compared to metals with regard to their design, fabrication and assembly, quality control and maintenance. One main advantage to using a composite over a metal structure is its high strength-to-weight ratio. Weight reduction is a primary objective when designing structures using composite materials. In addition, the use of composites allows the formation of complex, aerodynamically contoured shapes, reducing drag and significantly extending the range of the aircraft. Composite strength depends on the types of fibres and bonding materials used and how the part is engineered to distribute and withstand specific stresses. Composite materials are found in the manufacture of primary flight controls and high-integrity structural repairs in aircraft. Composite Elements In aircraft construction, most currently produced composites consist of a reinforcing material to provide structural strength, joined with a matrix material to serve as the bonding substance. In addition, adding core material saves overall weight and gives shape to the structure. The three main parts of a fibre-reinforced composite are the fibre, the matrix and the interface or boundary between the individual elements of the composite. Reinforcing Fibres Reinforcing fibres provide the primary structural strength to the composite structure when combined with a matrix. Reinforcing fibres can be used in conjunction with one another (hybrids), woven into specific patterns (fibre science), combined with other materials such as rigid foams (sandwich structures) or simply used in combination with various matrix materials. Each type of composite combination provides specific advantages. Following are the four most common types of reinforcing fibres used in commercial aircraft composites: Fibreglass (glass cloth) Aramid Carbon/graphite Ceramic. Fibreglass (Glass Cloth) Fibreglass is made from small strands of molten silica glass (about 1260 °C) that are spun together and woven into cloth. Many different weaves of fibreglass are available, depending on the particular application. Its widespread availability and its low cost make fibreglass one of the most popular reinforcing fibres. Fibreglass weighs more than most other composite fibres, but has less strength. In the past, it was used for non-structural applications; the weave was heavy and added polyester resins made the part brittle. Recently, however, newly developed matrix formulas have increased the benets of using fibreglass. Aramid In the early 1970s, DuPont introduced Aramid, an organic aromatic-polyamide polymer commercially known as Kevlar®. Aramid exhibits high tensile strength, exceptional flexibility, high tensile stiffness, low compressive properties and excellent toughness. The tensile strength of Kevlar® composite material is approximately 4 times greater than alloyed aluminium. Aramid fibres are non-conductive and produce no galvanic reaction with metals. Another important advantage is its outstanding strength- to-weight ratio; it is very light compared to other composite materials. Aramid-reinforced composites also demonstrate excellent vibration-damping characteristics in addition to a high degree of shatter and fatigue resistance. A disadvantage of Aramid is that it stretches, which can cause problems when it is cut. Drilling Aramid can also be a problem if the drill bit grabs a fibre and pulls until it stretches to its breaking point. Carbon fibre Advantages of carbon materials are their high compressive strength and degree of stiffness. However, carbon fibre is cathodic, while aluminium and steel are anodic. Thus, carbon promotes galvanic corrosion when bonded to aluminium or steel, and special corrosion-control techniques are needed to prevent this occurrence. Carbon materials are kept separate from aluminium components when sealants and corrosion barriers, such as fibreglass, are placed at the interfaces between composites and metals. To further resist galvanic corrosion, anodise, prime and paint any aluminium surfaces prior to assembly with carbon material. Carbon-fibre composites are used to fabricate primary structural components such as ribs and wing skins. Even very large aircraft can be designed with a reduced number of reinforcing bulkheads, ribs and stringers thanks to the high strength and high rigidity of carbon-fibre composites. Carbon fibre is stronger in compressive strength than Kevlar®, but it is more brittle. Ceramic Fibre Ceramic fibres are used in high-temperature applications. This form of composite will retain most of its strength and flexibility at temperatures up to 1200 °C. For example, tiles on the space shuttle are made of a special ceramic composite that dissipates heat quickly. Some firewalls are also made of ceramic-fibre composites. The most common use of ceramic fibres in civilian aviation is in combination with a metal matrix for high-temperature applications. Fibre Science The selective placement of fibres needed to obtain the greatest amount of strength in various applications is known as fibre science. The strength and stiffness of a composite depends on the orientation of the plies to the load direction. A sheet-metal component will have the same strength no matter which direction it is loaded. For example, if a wing in flight bends upwards as well as twists, the part can be manufactured so one layer of fibres runs the length of the wing, reducing the bending tendency, and another layer runs at 45° and at 90° to limit the twisting. Each layer may have the major fibres running in a different direction. The strength of the fibres is parallel to the direction the threads run. This is how designers can customise fibre direction for the type of stress the part might encounter. In flight, the structure tends to bend and twist. The fibre layers are laid in a way that limits these forces, thereby customising a part to the type of stresses encountered. Composite Fabrics Fabric Orientation When working with composite fibres, it is important to understand the construction and orientation of the fabric because all design, manufacturing and repair work begins with the orientation of the fabric. Unlike in metallic structures, the strength of a composite structure relies on the proper placement and use of the reinforcing fibres. Some of the terms used to describe fibre orientation are warp, weft, selvage edge and bias. Warp The warp of threads in a section of fabric run the length of the fabric as it comes off the roll or bolt. Warp direction is designated as 0°. There are typically more threads woven into the warp direction than the fill direction, making the fibre stronger in the warp direction. Because warp is critical in fabricating or repairing composites, insertion of another colour or type of thread at periodic intervals identifies the warp direction. Weft/Fill Weft, or fill, threads of the fabric are those that run perpendicular (90°) to the warp fibres. The weft threads interweave with the warp threads to create the reinforcing cloth. Selvage Edge The selvage edge of the fabric is the tightly woven edge parallel to the warp direction, which prevents edges from unravelling. The selvage edge is removed before the fabric is utilised. The weave of the selvage edge is different from that of the body of the fabric and does not have the same strength characteristics as the rest of the fabric. Bias The bias is the fibre orientation that runs at a 45° angle (diagonal) to the warp threads. The bias allows for manipulation of the fabric to form contoured shapes. Fabrics can often be stretched along the bias but seldom along the warp or fill. Fabric Styles Fabrics used in composite construction are manufactured in several different styles: unidirectional bidirectional multidirectional. Component designers can use any or all of these fabric styles, depending on the strength and flexibility requirements of the component part. Unidirectional A unidirectional fibre orientation is one in which all of the major fibres run in one direction, giving the fabric the majority of its strength in a single direction. This type of fabric is not woven together, meaning there are no fill fibres. Occasionally, small cross threads are used to hold the major fibre bundles in place. However, the cross threads are not considered woven fibres. Bidirectional/Multi-directional Bidirectional or multi-directional fabric orientation calls for the fibres to run in two or more directions. Bidirectional fabrics are woven with the warp threads usually outnumbering the weft, so there is usually more strength in the warp direction than in the weft. Fabric Weaves Fabrics are woven together in a number of weaves and weights. Woven fabrics are more resistant to fibre breakout, delamination and damage than unidirectional materials. Because of the wide variety of uses and strength requirements, composite fabrics are available in many weaves. Some of them are: Plain weave Satin weave Twill weave. Plain Weave In this most simple weave pattern, warp and fill yarns are interlaced over and under each other in alternating fashion. The plain weave provides good stability, porosity and the least yarn slippage for a given yarn count. Satin Weave In the satin weave, the warp floats or skips over as many as 12 fill yarns before being woven in. A satin weave is notable for its smooth surface created by the relatively long warp yarn floats. Twill Weave Twill weaves repeat on three or more warp and fill yarns. Twill weaves have a distinctive diagonal line on the surface of the fabric and come in many variations. The most common weaves used in advanced composite aircraft construction are the plain and satin weaves. Composite Bonding Matrix Systems The matrix is a bonding material that completely surrounds the fibre, giving it extra strength. The strength of a composite lies in the ability of the matrix to transfer stress to the reinforcing fibres. An advanced composite uses various manufacturing techniques and newer matrix formulas with newer reinforcing fabrics. Polyester resin is an example of an early matrix formula used with fibreglass for many non-structural applications such as fairings, spinners and trim. The old polyester/fibreglass formulas did not offer sufficient strength for fabricating primary structural members; it can be somewhat brittle. The newer matrix materials display remarkably improved stress-distributing characteristics, heat resistance, chemical resistance and durability. Most of the newer matrix formulas for aircraft are epoxy resins. Resin matrices are two-part systems consisting of a resin and a catalyst or hardener, which acts as a curing agent. The term resin often means both parts together, not just the resin. Resin Matrix Systems Resin matrix systems are a type of plastic. Some companies refer to composites as fibre-reinforced plastics. There are two general categories of plastics: thermoplastic thermosetting. By themselves, these resins do not have sufficient strength for use in structural applications, however, when used as a matrix and reinforced with other materials, they form the high strength, lightweight structural composites used today. Thermoplastic Resins Thermoplastic resins use heat to form the part into the desired shape, one that is not necessarily permanent. If a thermoplastic is heated a second time, it will ow to form another shape. Two types of transparent thermoplastic materials are used for aircraft wind shields and side windows: cellulose acetate and acrylic. Early aircraft used cellulose acetate plastic because of its transparency and light weight. However, it tends to shrink and turn yellow and therefore has almost completely been replaced. Acrylic plastics are identified by such trade names as Lucite or Plexiglas, or in Britain by the name Perspex. Acrylic is stiffer than cellulose acetate, is more transparent and, for all practical purposes, is colourless. Thermosetting Resins Thermosetting resins are usually liquids or low-melting-point solids in their initial form. When used to produce finished goods, they are cured by the use of a catalyst, heat or a combination of the two. Once cured, solid thermosetting resins cannot be converted back to their original liquid form. Unlike thermoplastic resins, cured thermosets will not melt and ow, but will soften when heated (and lose hardness). Once formed, they cannot be reshaped. At this time, two common structural airframe applications are: Polyester resin Epoxy resin. Polyester Resin Polyester resin, an early thermosetting matrix formula, is mainly used with fibreglass composites to create non-structural applications such as fairings, spinners and aircraft trim. While fibreglass possesses many virtues, its greatest limitation lies in its lack of structural rigidity. Polyester resins give fibreglass cohesiveness and rigidity. The actual cure of polyester resin occurs when a chemical reaction between the catalyst and accelerator generates heat within the resin. The less surface area there is for heat to escape, the more heat remains in the resin and the faster it cures. Therefore, when submitted to identical conditions, a thick layer of resin cures more rapidly than a thin layer. Warning: Never mix accelerators directly with catalysts as they will violently react with each other, and catch on fire or explode, if not diluted by the resin first. Epoxy Resins Most of the newer aircraft composite matrix formulas utilise epoxy resins, which are thermosetting plastic resins. Epoxy resin systems are well known for their outstanding adhesion, strength, and resistance to moisture and chemicals. Not all types of epoxy resin is suitable for every type of structure or repair. Make sure to use the resin called for in the manufacturer’s repair manual. Epoxy resins are two-part systems consisting of a base resin and a hardener – not a catalyst – for curing. Hardener is mixed in larger amounts than the catalyst used in the previously described polyester resin, which was an early thermosetting matrix formula. Adhesives (Bonding Agents) Resins come in different forms. Resins used for laminating are generally thinner to allow proper saturation of the reinforcing fibres. Others are used for bonding and are typically known as adhesives or bonding agents because they glue parts together. Adhesive resins and catalysts are available either in premixed quantities or in separate containers. One of the most unique forms of adhesive is the film adhesive. This type of adhesive pre-blends the resin and catalyst on a thin film of plastic. Refrigeration of the film is required to slow the cure rate (the rate of change to its permanent form) of the resin. If left out at room temperature, the resin and catalyst will start to cure. In the freezer, the curing process slows, lengthening the shelf life of the film. Adhesive films are often used to help bond patches to a repair area. Another form of adhesive is available in foam, which is primarily used to splice replacement honeycomb core segments to existing cores. When heat is applied to the adhesive, it foams up and expands into crevices. These types of foaming adhesives can also be used to permanently install fasteners. Pre-impregnated Materials Pre-impregnated fabrics, commonly known as ‘pre-pregs’, are fabrics that have the resin system already saturated into the fabric. Because many epoxy resins have high viscosity, it is often difficult to mix and work epoxy resins into the fabric to completely encapsulate the fibres. Fabrics are pre- impregnated with the proper amount and weight of a resin matrix to eliminate the mixing and application details, such as proper mix ratios and application procedures. Pre-preg materials offer convenience over raw fabrics in many ways: The pre-preg contains the proper amount of matrix. The reinforcing fibres are completely encapsulated with the matrix. During hand lay-up, if a resin system has a high viscosity or is very thick, it is sometimes difficult to get the resin into and around each individual fibre to produce the strongest cure. This is not a problem with pre-preg fabrics. Pre-preg fabrics eliminate the need to manually weigh and mix components. In hand lay-up, the resin and curing agent must be properly weighed. In many cases, pre-preg materials produce a stronger component or repair. Pre-pregs were invented for use by aircraft manufacturers to reduce the problems associated with completely wetting the fabric with resin. They also save time and reduce the problems associated with weighing and mixing the resins. Pre-preg fabrics also have disadvantages when working in a maintenance facility. Some of the disadvantages of working with pre-pregs are: Many pre-pregs must be stored in a freezer. This requirement must be met. If some pre-pregs are allowed to remain at room temperature for even a few hours, the resins/catalysts start their chemical reaction and begin to cure. Pre-preg fabrics usually have a limited shelf life even if kept in the freezer. Pre-preg material is much more expensive than raw fabric that can be impregnated with the same type of resin system. This is especially true if the material exceeds its shelf life and must be discarded. Moisture, dust or other contaminants will compromise bond durability. Warning: Bags containing pre-preg material should be opened only in a controlled environment and not opened until the material has thawed to room temperature. Fillers Fillers, also known as thixotropic agents, are materials added to resins to control viscosity and weight, to reduce pot life and cured strength, and to make application of the resin easier. Fillers increase the volume of the resin, making it less dense and less susceptible to cracking as well as lowering its weight. Most fillers are inert and will not react chemically with the resin. Micro balloons, chopped bres and flox are common types of fillers used in composite construction. Micro balloons are small spheres manufactured from plastic or glass. Plastic micro balloons must be mixed with a compatible resin system that will not dissolve the plastic. The advantages to using micro balloons are that they provide greater concentrations of resin in the edges and corners of the structure; they are less dense, which reduces the overall weight; and they provide lower stress concentrations throughout the structure. However, micro balloons do not add strength to the composite structure. Flox is the fuzzy fibre taken from the fabric strands. Both chopped bres and flox may be used when added strength is desired. If a hole in a composite structure needs to be filled, a mixture of resin and flox will provide more strength than pure resin. Using pure epoxy resin produces brittle and heavy plugs. Resin and Catalyst Terminology Shelf Life This is the time span that a product will remain useful. It should be listed on the label. Temperature during storage will affect the shelf life. Pot Life and Gel Time Pot life and gel time are strictly related to the activity of the catalyst. They are governed by both the proportion of the catalyst and the ambient temperature. For a given proportion of catalyst, the higher the air temperature, the shorter the pot life and gel time of the resin. Hardening Time Hardening time can vary greatly depending on the size and thickness of the moulding and the proportion of resin present. It will also again be affected by the air temperature. Maturing Time The further period of time over which the moulding will continue to gain hardness and, eventually, complete stability is called the maturing time. When fully matured, the moulding will achieve its maximum strength, hardness, chemical resistance and stability. Mix Ratio A slightly improper mix ratio can make a tremendous amount of difference in the strength of the final composite. Because the mixing procedures are so important, they are always included with the resin containers. The resin manufacturer outlines the correct mixing procedures and this may be displayed on the container. Manufacturers often produce pre-measured matrix packages. The advantage of using pre-packaged resin systems is that they eliminate the weighing process and therefore remove the possibility of a mixing ratio error. The resin and catalyst are divided into separate containers that are attached at one end. When they are ready for use, the partition which separates the resin from the catalyst is broken to allow the two to mix. Still within the package, the resin/catalyst combination is mixed together by squeezing and kneading the package to thoroughly blend the mixture. When completely mixed, the package is cut with scissors and the resin dispensed. Disposable cartridges that store, mix and apply two-component materials are also available and convenient to use. They are available in many sizes and can be tailored to specific uses. Like the pre- measured packages, cartridges also eliminate mixing-ratio errors. To use epoxy cartridges, the seal that separates the two components must be broken with a plunger. The materials are then mixed together by moving the plunger in a twisting and up-and-down motion to thoroughly mix the resin and catalyst. The label describes how many strokes are required to give a thorough mix. A needle or syringe may then be installed onto the end of the cartridge, and the resin dispensed. Be sure to check the cartridge part number, shelf-life expiration date and any special instructions. Disposing of Resins Dispose of cured polyester resin/epoxy products in accordance with local regulations. Time-expired polyester resin systems are disposed by mixing them with the appropriate catalyst and then disposing of the cured product in accordance with local regulations. Dispose of time-expired two-part epoxy resin systems by mixing the two components together and disposing of the cured product in accordance with local regulations. Do not dispose of unmixed, uncured polyester resin/epoxy in general waste bins. Do not dispose of catalyst-soaked rags in general waste bins as rags could spontaneously combust. Contact local hazardous-material-collection agencies for collection and disposal of large quantities of uncured product, accelerators and catalysts.