Natural Variability: El Niño/Southern Oscillation (ENSO) PDF
Document Details
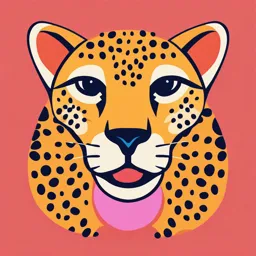
Uploaded by GreatestTigerEye
null
Tags
Summary
This document reviews El Niño/Southern Oscillation (ENSO), a major mode of natural variability in the Earth system. It examines the coupled interactions between atmosphere and ocean in the tropical Pacific, highlighting global implications and changes in trade winds, resulting changes in SST (sea surface temperature) patterns. The document also notes how El Niño and La Niña impact global weather and rainfall, with impacts on health and other phenomena.
Full Transcript
Natural Variability: El Niño/Southern Oscillation (ENSO) OCN-310 September 30, 2024 Summary Looked at the mean atmospheric and oceanic circulation patterns and what drives these Looked at the carbon system and geochemistry of Earth system Next step: look at modes of natural...
Natural Variability: El Niño/Southern Oscillation (ENSO) OCN-310 September 30, 2024 Summary Looked at the mean atmospheric and oceanic circulation patterns and what drives these Looked at the carbon system and geochemistry of Earth system Next step: look at modes of natural (internal) variability Why do this? Natural Variability Short-term Diurnal variability (usually minor) Annual variability (usually major) Long-term Orbital dynamics, Milankovitch Cycles Now focus on interannual to decadal El Nino/Southern Oscillation (ENSO) Pacific Decadal Oscillation (PDO) Others Coupled System Atmosphere drives the oceanic circulation via winds (and buoyancy, aka density changes) Ocean drives atmospheric circulation via sea surface temperature Coupled system leads to feedbacks positive feedback: self-enhancing negative feedback: self-damping What is normal? Average Sea Surface Temperatures (SST; oC) NOTE: Sea level can be a proxy for ocean circulation, just like winds can be estimated from High/Low pressure systems, ocean currents are associated with highs/lows in sea level Another NOTE: These values of sea level are very small (e.g., centimeters) and very large horizontal scale (e.g., basin-wide) Mean sea level These are differences from a mean sea level (in mm) Mean sea surface temperature East-west gradient in SST leads to east-west circulation in atmosphere à Walker Cell Subsurface temperature Heating at the surface and wind mixing: Surface, warm mixed layer Thermocline Cold deep Implications for the ocean Variability on interannual time-scales El Niño Original definition: Annual warming of water off of the Coast of Peru around Christmas. “El Niño” referred to the Christ child. Since early 1980ʼs new definition: Warm water occurring off Peru and reaching out to the dateline that tends to occur periodically every 2-5 years. Measured using Southern Oscillation Index Differences in pressure observed in Tahiti and Darwin, Australia El Niño/Southern Oscillation (ENSO) 3- to 5-year variability in tropical Pacific, leads to changes in atmospheric phenomena world-wide Key concepts: Coupled air-sea phenomenon in the Tropical Pacific Implications are global Driven by, or defined by, an adjustment of the mean circulation à Walker Cell Large-scale, equatorial waves propagate the change Normal ocean temperatures The big slosh of warm ocean temps to the eastern Pacific. Interannual Variability Examples Note, typically look at anomalies… Value minus average value for that season 1982-1983. The El Niño that “the rest of the world” finally noticed. El Niño Strong trade winds Westward currents, upwelling Cold east, warm west Convection, rising motion in west Weak trade winds Eastward currents, suppressed upwelling Warm west and east Enhanced convection, eastward displacement Key Points Local (equatorial Pacific) phenomenon with global implications Measured by sea level pressure difference (SOI) Coupled air-sea interaction critical Predictable several months in advance with limited skill Differences Not all El Niño events are the same Climate change may be causing this difference Example for 1997 La Niña El Niño sometimes referred to as “warm ENSO” or “warm event” Counter example of oscillation is referred to as La Niña, or “cold event” La Niña Strong trade winds Westward currents, upwelling Cold east, warm west Convection, rising motion in west Stronger trade winds Westward currents, enhanced upwelling Colder east, warmer west Enhanced convection Ways to monitor Pressure difference between east and west Pacific: Southern Oscillation Index (SOI) Sea surface temperature difference between east and west Pacific: NINO index Southern Oscillation Index Recall low pressure = high SST, so if SOI NEGATIVE: Pressure at Tahiti < Pressure at Darwin SST in eastern Pacific > SST in western Pacific (in anomalies) El-Nino event Different El Niño-Southern Oscillation Indices based on region of the Pacific Ocean: The Niño 3 Region is bounded by 90°W-150°W and 5°S- 5°N. The Niño 3.4 Region is bounded by 120°W- 170°W and 5°S- 5°N. Nino Index How known? Satellite In-situ buoy array (TAO) Implications Recognize this is complex: seasonal changes, local effects and differences in ENSO events make it hard to say what is “normal” However… El Nino impacts: rainfall La Nina impacts: rainfall El Nino impacts: health What’s happening now? Present Day Anomalies Last Year Forecast What about Hawaii? How will ENSO change? Summary ENSO is a major mode of natural variability and occurs on interannual time-scales Marked by changes in trade winds that in turn cause changes in SST patterns Warm, El Nino event has warmer temps in E. Pacific Cold, La Nina event has colder temps in E. Pacific It is a coupled air/sea phenomenon in the equatorial Pacific but has implications for global weather ENSO Review Tropical Pacific phenomenon, occurs periodically every 2 to 5 years Related to change in east-west temperature anomalies: El Nino/warm event: west cooler than normal, east warmer than normal La Nina/cold event: west warmer than normal, east colder than normal Coupled phenomenon, driven by change in trade winds Due to El Nino conditions, Hawaii’s drought is expected to continue well into next year, and possibly even into the 2024 dry season, according to the National Oceanographic and Atmospheric Administration. Low-frequency Variability OCN-310 October 2, 2024 East-West Walker Circulation Trade winds Cold east, warm west Rising motion and convection in west Weak trade winds Less warm in west and more warm in east Eastward displacement of convection Summary ENSO is a major mode of natural variability and occurs on interannual time-scales It is a coupled air/sea phenomenon in the equatorial Pacific but has implications for global weather ENSO responsible for changes in rainfall Under warming scenario, Pacific may enter a more El Nino-like state; with ENSO events becoming more extreme Lower-Frequencies ENSO represents the largest mode of inter-annual variability in the tropics. We now will look at other “low frequency modes”: 1. Pacific Decadal Oscillation (PDO) 2. North Atlantic Oscillation (NAO) 3. Arctic Oscillation (AO) 4. More? Pacific Decadal Oscillation (PDO) The PDO is similar to ENSO in the sense that it is an oscillation in Pacific Sea Surface Temperatures. The fundamental signature is SST in the Northern Pacific of opposite sign to those in the Equatorial Pacific The pattern shifts on a roughly 10- to 20 year time- scale PDO Positive Phase PDO Negative Phase Surface temperature (positive phase) Rainfall (positive phase) Rainfall (negative phase) PDO Implications The PDO primarily affects weather patterns and sea surface temperatures in the Pacific Northwest, Alaska, and northern Pacific Islands (Pacific North America pattern, or PNA) A positive phase of the PDO corresponds with a positive PNA pattern which is generally a ridge over western North America and a trough over eastern North America and the opposite is true of the negative phase of both. PDO warm phase/+PNA Warm sea surface temperature anomalies along the West Coast North America: ridge over the western and trough over eastern portion. PDO cold phase/-PNA The jet stream develops a trough along the West coast and a ridge develops over the eastern half the US. Deng et al., JGR 2013 South China Sea corals The relationship between the PDO and coral geochemistry may be related to the influence of the PDO on rainfall on Hainan Island. North Atlantic Oscillation "In Greenland, all winters are severe, yet they are not alike. The Danes have noticed that when the winter in Denmark was severe, as we perceive it, the winter in Greenland in its manner was mild, and conversely." Hans Egede Saabyes diary (1770-78) Arctic Oscillation positive phase negative phase Figures courtesy of J. Wallace, University of Washington Arctic Oscillation Negative phase: H over polar regions, L at lower latitudes Cold winter air to central US and Europe Positive phase: Lower over Arctic, higher pressure at mid. lat. Storm tracks further north è wetter in Alaska, Scotland, Scandinavia; drier in Mediterranean and W US; colder in Greenland and Newfoundland Low-frequency Variability Earth’s climate varies on many different timescales: Really low-frequency changes from orbital variations Decadal changes from large-scale ocean/atmosphere “modes” Interannual changes from ENSO All this with annual (seasonal) changes, higher frequency changes, daily changes Next step: what can we learn from historical records? Climate during the Phanerozoic OCN-310 October 4, 2024 Review Learned about the formation of the Earth System Next focused on the current “mean” state and the roles of the atmosphere and ocean More recently looked at natural modes of variability in the Earth System: Daily (rotation) Seasonal/annual (orbit/tilt) Interannual (ocean/atm coupling; ENSO) Decadal (ocean/atm coupling; PDO, NAO, etc.) Very long periods (orbital dynamics) Now we look at past climate states and see what we can explain Geologic Time Scale Recall Earth’s age is believed to be 4.5 billion years The evolution of the Earth since that time is divided into different periods: Largest division = EON (e.g., Archean, Phanerozoic) Next largest = ERA (e.g., Mesozoic, Cenozoic) Next largest = PERIOD (e.g., Devonian, Jurassic) Smallest division = EPOCH (e.g., Miocene, Pleistocene) The first geologic time scale was proposed in 1913 by the British geologist Arthur Holmes. This was soon after the discovery of radioactivity, and using it, Holmes estimated that the Earth was about 4.5 billion years old. Names given to these periods were usually derive from geographic regions where the rocks from that time were first studied (e.g., Pennsylvanian) No absolute dating at that time, so the timing was done relatively Once radiometric dating was discovered, the times were put in place and are continually being checked and refined oterozoic Eon 1. Proterozoic Eon From 2,500 – 570 mya Atmosphere enriches with oxygen due to bacteria—ozone layer forms Supercontinent Rodinia forms around 800 mya First multi-cell fossils – simple Stromatolites (algae) Eukaryotic cell fossils Extensive glaciations during “Snowball Earth” hanerozoic Eon 2. Phanerozoic Eon – “visible life” Three Eras spanning from 540 mya to present Paleozoic – “ancient life” Mesozoic – “middle life” Cenozoic – “recent life” Greatest diversity of land and ocean organisms Fossil record indicates complex organisms thrive Several mass extinctions 2.1 Paleozoic Era – “Cambrian Explosion” Spans 570 – 245 mya 7 periods of Paleozoic At the beginning of the Cambrian, the fossil record goes through an exponential increase in diversity and complexity 2.1 Paleozoic (cont’d) 3 distinct “ages” Age of invertebrates (shells) Age of fishes (vertebrates), Age of amphibians Massive swamps resulting in coal deposits of today Supercontinents Laurasia, Gondwanaland, and eventually Pangea form Disastrous extinction at the end of the Permian wiping out ~90% of all marine & ~70% of all land organisms 2.2 Mesozoic Era Mesozoic has 3 Periods from 245 – 66 mya Triassic Jurassic Cretaceous Rise of dinosaurs Pangea begins to break apart and form the current continents Warmer climates dominate 2.2 Mesozoic (cont’d) Reptiles rise, dinosaurs dominate, and shelled eggs help to protect offspring The most famous mass extinction occurs at the end of the cretaceous (K/T boundary) Main theory is asteroid impact changed climate so drastically that majority of animals failed to adapt 2.3 Cenozoic Era 2 Periods spanning from 66 mya to present day Tertiary Quaternary 7 Epochs are contained within the two periods, including Pleistocene and Holocene We are now in the Holocene 2.3 Cenozoic (cont’d) Cenozoic represents less than 2% of Earth’s history Mammals rise and become the dominant organism on land Angiosperms (flowers) become the dominant plant life on land Ice age advances occur during Pleistocene Many organisms go extinct with ice age climate changes Phanerozoic Eon Last 540-700 million years Coming out of “snowball” Earth Glacial/interglacial periods Continents “move” à Plate Tectonics Plate Tectonics (defs) Plate tectonics is a unifying theory in geology that explains a wide range of geologic phenomena. Tectonics: dealing with structural features of the Earth (e.g., mountains, ocean basins). Plate Tectonics: The process that involves the interaction of moving crustal plates and results in major structural features of the Earth. Early Theory: Contracting Earth First proposed by Giordano Bruno (16th century) who compared the process to the drying of an apple. Lord Kelvin (19th century) suggested that contraction was due to cooling of the Earth. Contracting Earth Shrinking resulted in a reduction in the Earth’s diameter while the circumference remained unchanged due to folding and buckling of the crust (diastrophism). Contracting Earth (problems) Fossils are preserved in rocks that represent organisms that could not withstand the early temperatures. Initial temperatures required for the amount of contraction were too high to be realistic. Other variations… 1. Extrusion of molten rock from within the Earth The amount of extrusive rock present is not enough to explain the crustal shortening that would be needed. 2. Chemical shrinkage (1920s) Decay of elements within the Earth to Helium which then escapes to the atmosphere. Combination of elements within the earth to form denser elements. Continental Drift Frances Bacon (1620), while reviewing the first maps of the coastlines of Africa and South America, noted that the outlines of the continents appear as if they could fit together. Frances Placet (1668) later suggest that the continents were actually fixed together as suggested by their outlines (pulled apart by biblical flood). Alfred Wegener (1912) published “The Origin of the Continents and Oceans” and became the “father of continental drift” [Note this “jigsaw” fit of continental margins is best when the outline is the edges of the continental shelves.] Evidence 1: The presence of fossils only over small areas of now separate continents (how did they get from continent to continent?). Evidence 2: Various climate zones. Evidence 3: Glacial deposits Evidence 4: “Cratons”, or building blocks of continents, line up Evidence 5: “regular” distribution of mountain ranges. Wegener concluded: The continents were once joined. Therefore, they must have moved apart over time. Contracting Earth theory was not consistent with the facts. The pushing of the continents by gravitational forces that derived from the sun and the moon (similar to tides) causes “continental drift” Not widely believed Went against long-held belief He had predicted the North America and Europe were moving away from each other at the rate of 250 cm/year……an impossible rate. The mechanism that Wegener proposed was impossible and easily demonstrated to be so. It was not until the 1950’s that evidence from the geological record of the Earth’s magnetic field began to strongly suggest exactly such movement. 1960’s: Glomar Challenger The ship took core samples of ocean floor rocks as it crossed the Atlantic Ocean A map was produced from this data showing the rock types and ages From this map a new theory was developed to explain Wegener’s evidence: Plate Tectonics Ocean floor rock ages Ocean floor rocks get older symmetrically away from ridges Theory: sea floor splits apart and rocks move away from each other Process: seafloor spreading Starting point: Pangea “snowball Earth” ~750 million years ago leads to supercontinent Pannotia Supercontinent Pangea formed ~350 million years ago (late Paleozoic) and began to break apart ~175 million years ago The very late Proterozoic is also the time of the Varangerian ice age. Signs of glaciation have been found on almost every continent. large creatures suddenly appeared on the scene, The mid Ordovician is the time of the Great Diversification, when sea-floor-dwelling filter feeders exploded into the greatest diversification of all time (more). Shortly after, round about the late Ordovician, the plants invaded the land. It was also one of the coldest times in Earth history. The climate was much warmer than today, and stayed so until the K-T extinction that killed off (most of) the dinosaurs. The early Jurassic was the start of the dinosaurs’ heyday – shortly after the Triassic extinction had killed off most of the competition. Primitive mammals found a niche for themselves too, but it was a pretty insignificant one. The Cretaceous climate was still balmy, right up to the poles. Dinosaurs and palm trees lived in both polar regions. Sea level was 100-200 meters higher than today, which created plenty of shallow seas as the continental margins were flooded. They provided plenty of channels whereby warm water could be transported towards the poles. Land/Ocean/Ice Variability Discussed general circulation of the ocean and atmosphere; how would this change if the land/ocean masses were different? What are the implications for climate? Climate Change after Pangea When the continents separated and reformed themselves, it changed the flow of the oceanic currents and winds. Summary Phanerozoic Eon Paleozoic Mesozoic Era Era Cenozoic Era Tertiary Period Quaternary Period Tertiary/Quaternary Climate varies between cold and warm in regular cycles Tertiary was warmer à no glaciation over continents Changes into Pleistocene, temperatures reduced to allow spreading of ice sheets over the continents in four different occasions Summary Phanerozoic Eon Paleozoic Mesozoic Era Era Cenozoic Era Tertiary Period Quaternary Period Paleocene/Eocene Thermal Maximum (PETM) Period of “greenhouse Earth” Rapid rates of seafloor spreading suggests high rates of magma to the Earth surface via volcanoes Large influx of CO2 into the atmosphere (positive radiative forcing) Temperatures rise à outgoing longwave to match incoming shortwave Later, volcanic activity decreases, leading to cooling Next Look at sea level over the geologic record Explore how we’ve come to develop hypotheses about past climate (temperatures) à proxy data Focus on more recent times “Recent” Climate Variability OCN-310 October 7, 2024 Review Phanerozoic Eon Paleozoic Mesozoic Era Era Cenozoic Era Tertiary Period Quaternary Period Ice Ages Louis Agassiz, a Swiss-American scientist and physician, was the first to recognize evidence for an ice age Trained in medicine and natural history, he was the first to propose, in 1837, that earth had been subjected to a past ice age 1807 - 1873 Ice Ages Ice ages - times when the entire Earth experiences notably colder climatic conditions During an ice age The polar regions are cold There are large differences in temperature from the equator to the pole Large, continental-size glaciers or ice sheets can cover enormous regions of the earth Alternate periods known as “greenhouse” periods Review Phanerozoic Eon Paleozoic Mesozoic Era Era Cenozoic Era Tertiary Period Quaternary Period Ice ages Five main ice ages since the Earth was formed: 1. Precambrian (2) 2. Ordovician-Silurian 3. Carboniferous-Permian 4. Quaternary Definitions Ice ages: Periods during which the Northern Hemisphere had ice sheets. Very few such periods. Includes the Pleistocene and Holocene (now) Glacial Period: Times when more than just Greenland/Antarctica have ice sheets (e.g., Pleistocene) Interglacial Period: Timed when only Greenland/Antarctica have ice sheets (e.g., the Holocene) Phanerozoic Eon Paleozoic Mesozoic Era Era Cenozoic Era Tertiary Period Quaternary Period Pleistocene Glaciations Evidence Glacial deposits Oxygen Isotope Possible Explanation Milankovitch Theory Issues Climate Feedbacks (?) Pleistocene Glaciation Began about 1.6 million years ago There have been at least 5 glacial advances in past 500 thousand years Climate cooled 5-10ºC during glacial episodes, warming in between Last episode peaked 18,000 years ago, ice covering about 30% of the earthʼs surface North American Ice Cover Figure shows the extent of ice cover from 18,000 to 8000 years ago How to explain ice age variability in Pleistocene Epoch? Three main cycles in Earth/Sun/etc system (recall lecture on orbital dynamics): 1. Eccentricity: 100,000 years 2. Obliquity: 41,000 years 3. Precession: 19,000-23,000 years The correlation between eccentricity and global temperature was striking and supported Milankovich’s ideas. Effects of the Milankovitch Hypothesis The effect of the various cycles is to change the contrast between seasons Milder winters in high latitudes lead to climate warming, and greater snowfall Cooler summers would reduce snowmelt Combined, this might trigger ice formation, and lead to an ice age This can explain the alternating glacial-interglacial effects seen in the Pleistocene Other Factors Effecting Climate Composition of the earth’s atmosphere Variations in reflectivity of the earth’s surface GHG (natural and anthropogenic) Stable States Equilibrium states can be represented with the glacial state (longer periods) in a deeper valley than the interglacial state. Orbital forcing periodically and continually send the system from one valley to the other, back and forth. Explanation (?) Why is the 100k-year cycle so pronounced in the actual climate, when the solar forcing is so muted? There must be one or more positive feedbacks – to amplify the initial change. 1. Ice-Albedo Feedbacks 2. Evidence for Feedbacks affecting atmospheric CO2 on Glacial Time Scales 3. Cloud-Albedo Feedbacks 4. Changes in Terrestrial Biomass (negative feedback!) Ice-Albedo Feedback Feedback: Biological Pump Why should the biological pump respond to glacial changes? We know that sea level drops during glacial periods, could this lead to phosphorus fertilization? Feedback: Biological Pump Feedback: Iron Fertilization Glacial periods are believed to have been dry and dustier, as shown in ice cores (more dust in the core). Feedback: Reefs We know that sea level drops during glacial periods, could this lead to a coral reef feedback? Feedback: Reefs Feedbacks: Cloud Albedo The production of DMS by phytoplankton lead to cooling of climate via 2 mechanisms – sulfate aerosol and cloud seeding Feedbacks: Forest Cover Feedbacks: Forest Cover Difference in vegetation between the last glacial maximum and present day potential: (ignoring human impact on forests). Holocene Quaternary Period, last 1.6 million years, marks time of ~regular ice ages Explanation from orbital variability and feedbacks Now look at more recent history: the Holocene 18,000 years ago 32% of the land surface and 30% of the ocean surface were covered by glaciers. 10 million square kilometers of North America was covered by a continental ice sheet up to 3 km thick. The center of greatest ice thickness was near Hudson Bay. Isostatic subsidence depressed the land surface by approximately 380 meters. The region has rebounded by about 300 meters and continues to rise at 2 cm/yr. Holocene Start: LGM General warming over the Holocene following the Last Glacial Maximum (LGM) Key events Dramatic drop (and recovery) 12,000 to 10,000 years ago (Younger Dryas): example of “abrupt climate change” Key events Period of warming between 6,000 and 4,000 years ago (Holocene Maximum) Key events Recent warming “Medieval warm period” General warming over the Holocene following the Last Glacial Maximum (LGM) Dramatic drop (and recovery) 12,000 to 10,000 years ago (Younger Dryas): example of “abrupt climate change” Period of warming between 6,000 and 4,000 years ago (Holocene Maximum) Shorter warming during “medieval warm period” Mann et al., GRL, 1998 https://www.theatlantic.com/technology/archive/2013/03/were-screwed-11- 000-years-worth-of-climate-data-prove-it/273870/ Summary 1. For much of the Earth’s history, the climate was warmer than today. 2. We are presently living in a long-term “Icehouse” climate period, which is comprised of shorter-term glacial (e.g., 21,000 ybp) and interglacial (e.g., today) periods. There were four periods of Icehouse prior to the current one. 3. Last Glacial Maximum = 21,000 ybp (maximum North American continental glaciers, lower sea level exposed Bering land bridge allowing human migration from Asia to North America) 4. Younger-Dryas Event = 12,000 ybp (sudden drop in temperature and portions of N.H. reverted back to glacial conditions) Summary (cont’d) 5. Holocene Maximum = 5,000-6,000 ybp 6. Medieval Climate Optimum (Warm Period) = Warming during 1,000 A.D. – 1,300 A.D. in Europe and the high-latitudes of North Atlantic (N.H. warm and dry, Nordic people or Vikings colonized Iceland & Greenland) 7. Little Ice Age = Cooling during 1,400 A.D. – 1,900 A.D. 8. Over the last century, the earth’s surface temperature has increased by about 0.75°C (about 1.35°F). E. J. Judd et al., 2024. A 485-million-year history of Earth’s surface temperature, Science, 385(6715) DOI: 10.1126/science.adk3705 https://xkcd.com/1732/ https://www.youtube.com/watch?v=3mEIDcnwc-g Past Climate: Proxy Data OCN-310 October 9, 2024 Phanerozoic Eon Paleozoic Mesozoic Era Era Cenozoic Era Tertiary Period Quaternary Period From last time…https://xkcd.com/1732/ Time-scales of Climate Change Climate Signals We’ve looked at temperature records and variability over a variety of time-scales (years, decades, millennia, etc.) How do we get information on these time-scales? à Proxy data Climate Information Direct evidence Observations (e.g., tide gauges, thermometers, satellites, etc.) Historical documents Indirect evidence Proxy data Numerical models Test feedbacks Test forcing mechanisms Shen Kuo (1031 – 1095) mathematician, astronomer, meteorologist, geologist, zoologist, botanist, pharmacologist, agronomist, archaeologist, ethnographer, cartographer, encyclopedist, general, diplomat, hydraulic engineer, inventor, academy chancellor, finance minister, governmental state inspector, poet, and musician discovered the concept of true north in terms of magnetic declination towards the north pole with experimentation of suspended magnetic needles devised a geological hypothesis for land formation (geomorphology) based upon findings of inland marine fossils, knowledge of soil erosion, and the deposition of silt proposed a hypothesis of gradual climate change, after observing ancient petrified bamboos that were preserved underground in a dry northern habitat that would not support bamboo growth in his time. Paleo-climatology: The Study of Past Climates The study of past climates prior to the instrument record. Scientists use indirect evidence (data) during past time periods to determine the climate at that time period. These climate imprints are referred to as proxies. Tree Rings Sediment Pollen Cores Examples of Climate Proxies Stable Ice Cores Isotopes Coral Reefs Calibration How to convert observation, e.g., tree ring, into variable, e.g., temperature? “calibration-in-time” Determine the relationship is between the proxy values and direct observations during the recent period in which climate observations exist, and use that relationship to infer values in the more distant past. “calibration-in-space”. Measure the proxy across a wide spatial range of modern environments, where the controlling factors – such as temperature – are known. This technique is used when direct comparison with observational records is not possible. 13 Paleoclimate Instrumental Records Records Error bars for proxy data 1. Instruments: satellites Satellite measurements have been used to construct globally complete land and oceanic temperatures since 1979. Provides a spatially uniform perspective whereas weather observations are biased towards where people are located. Allows for measurements over hard- to-sample areas like the oceans and ice sheets. Source: NOAA Source: NOAA 2. Instruments: thermometers The earliest records of temperature measured by thermometers are from western Europe in the late 17th century and by the early 20th century records were being collected in almost all regions. Records from polar regions began in the 1940s. The National Climatic Data Center maintains a collection of temperature records from over 7,000 stations worldwide, about 1,000 go back to the 19th century. Temperature observations the first 2 weeks of July 1776 in Thomas Jefferson’s Weather Memorandum Book Yearly Temperature Change Since 1850 Data from thermometers http://commons.wikimedia.org/wiki/Image:Instrumental_Temperature_Record.png 3. Historical Documents New study that analyzes paintings going back to the 1500s to study the way atmospheric pollutants impact the climate. Specifically, researchers at Greece’s Academy of Athens say, they were able to use depictions of sunsets to measure something called aerosol optical depth (AOD), a measure of particles, or aerosols, in the atmosphere Historical Records of “Weather” Non-instrumental accounts Restricted to weird or extreme events Recorded in literature, birth and death records, art works and theater productions from those time periods. Instrumental accounts and records In the renaissance, science became enamored with measuring stuff First temperature, then wind, moisture, and pressure Historical documents contain past weather and climate information. Ship logs are particularly useful for accounts of sea ice, storms, and hurricanes. Farmers’ logs can include useful information such as planting or harvest dates and overall crop health. Personal diaries are another resource. “Little Climate Optimum” Occurred from about 800 to 1300 AD Records of civilizations abound in what are now harsh climatic regions Greenland- “Norse” colonies Wari Empire- Peru, South America Pueblo culture - Chaco Canyon, NM Anasazi culture- Mesa Verde, CO Causes problems in some coastal areas Sea level rises flooding places along the Mediterranean Sea “Little Ice-Age” Occurs from 1350 to 1850 AD Produces cooler weather and forces the termination of all of the cultures mentioned in association with Climatic optimum Ice becomes commonplace in places that do not currently freeze, e.g., Thames River- England 4. Tree rings (Dendrochronology) One way that trees grow is to add a layer of new wood between the old wood and the bark Trees generally produce one ring each year. Thick rings = good growing conditions Thin rings = stressed conditions Trees ring records can extend back ~1000’s years. Tree rings 25 26 Tree Rings 29 Example Record A several decade long North American drought in the late 16th century is apparent from tree ring records. 5. Pollen Pollen grains are especially well preserved in sediment layers, like at the bottom of a lake or ocean. All flowering plants produce pollen grains. Each kind of pollen has a distinct shape so we can identify the type of plant. Pollen grains are preserved in sediment layers in the bottom of ponds, lakes and oceans. Analyzing the pollen grains in each sediment layer tells us what kinds of plants were growing. The kind of plant is a clue to what the climate was like. 6. Ice Cores The Ice Core Record Ice sheets contain a record of hundreds of thousands of years of past climate, trapped in the ancient snow. http://tvl1.geo.uc.edu/ice/Image/pretty/green.html Ice Cores As snow and ice accumulate in polar glaciers a paleoclimate record accumulates of the environmental conditions of the time of formation. Ice cores can analyzed using stable isotope approaches for water or air bubbles within the ice as a record of past atmospheric gas concentrations. Vostok Station, Antarctica Side Note on Ice Cores First used in stratigraphic sense: If present, cold If thicker, colder (more snow) If thinned, warmer (less snow) Starting the 1980’s, isotopic analysis was used Dust, gas and chemicals also examined Ice cores typically go back around 500,000 years Limited to areas with ice… Ice accumulates year after year in distinctive layers Light and dark bands distinguish between winter and summer snowfall Changes in atmospheric gases The graph shows changes in carbon dioxide levels over the last 400,000 years Changes in temperature Temperature change for the past 150,000 years from an Antarctic ice core based on a hydrogen isotope proxy. 7. Varved Lake Sediments Complement tree ring records; most common in cold-temperature environments Occur in deeper parts of lakes that do not support bottom- dwelling organisms that would obliterate annual layers with their activity Layers act as proxy of precipitation amount Lake Core Sampling 8. Coral Reefs Corals are composed of calcium carbonate. This carbonate contains isotopes of oxygen that can be used to determine the water temperature when and where the corals grew. Corals Texture of calcite (CaCO3) incorporated in skeletons varies; lighter parts during periods of rapid growth in summer and darker layers during winter Limited to tropical climates Corals as climate archives 49 9. Marine Sediments 50 51 Long cores drilled by specially equipped ships Marine Sediments Isotopes in shells of foraminifera can reveal temperature, salinity, and ice volume Granular debris from land can indicate icebergs breaking off of continental ice sheets, suggesting cold climates 10. Other Methods Speleothems (caves) Fossilized plants (vegetation types) and leaf size and shape. 55 Sea Level https://www.scirp.org/journal/paperinformation.aspx?paperid=10089 2 Glacial Pair Photography On the left is a photograph of Qori Kalis Glacier taken in July 1978, and on the right, a photograph taken from the same vantage in July 2004 Both photographs taken by Lonnie G. Thompson, Byrd Polar Research Center, the Ohio State University Muir Glacier On the left is a photograph of Muir Glacier taken on August 13, 1941, by glaciologist William O. Field; on the right, a photograph taken from the same vantage on August 31, 2004, by geologist Bruce F. Molnia of the United States Geological Survey (USGS). Notes Keep in mind temporal resolution (e.g., year to year vs decades) Location is also important (e.g., tropics vs high latitudes) Relationship between proxy variable (e.g., temperature) and measurement (e.g., tree ring) may have changed over time Composite CPS and EIV NH land and land plus ocean temperature reconstructions and estimated 95% confidence intervals. ©2008 by National Academy of Sciences Michael E. Mann et al. PNAS 2008;105:36:13252-13257 Phanerozoic Eon Paleozoic Mesozoic Era Era Cenozoic Era Tertiary Period Quaternary Period https://interactive.carbonbrief.org/how-proxy-data-reveals-climate-of-earths-distant-past/