Colloq IV Script - Aurora Killi Part 2 - PDF
Document Details
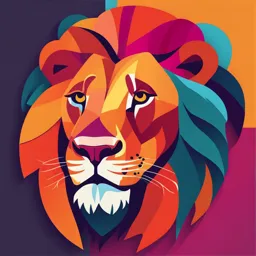
Uploaded by HugoverMedic
Rīgas Stradiņa universitāte
Aurora Killi
Tags
Summary
This document is a script for a lecture or presentation on the auditory and vestibular sensory systems. It covers topics such as sound transmission, coding of sound pitch/loudness. It also investigates Audiometry, Weber and Rinne tests and deafness. It's suitable for undergraduate-level biology or related course.
Full Transcript
Aurora Killi...
Aurora Killi 26.02.22 Auditory and vestibular sensory system Auditory sensory system. Functions of external and middle ear......................................................................................................... 2 Sound transmission in inner ear. Mechanism of activation of receptors............................................................................................ 7 Coding of the sound pitch, loudness, and localization....................................................................................................................... 12 Audiometry, Weber and Rinne test. Conduction and neural deafness............................................................................................. 14 Equilibrium sensory system. Receptors in semicircular canals; adequate stimuli and mechanism of activation............................. 17 Equilibrium sensory system. Receptors in vestibulum; adequate stimuli and mechanism of activation.......................................... 20 Vestibular reflexes. Vestibulo-ocular reflexes.................................................................................................................................... 22 1 Aurora Killi 26.02.22 Auditory sensory system. Functions of external and middle ear Auditory sensory system Sound is produced by vibrating object that causes compression and rarefication of air molecules Sound waves → the distance between two compression sites o Peak of wave → refers to the most compressed air molecule site o Bottom of wave → refers to the most rarified molecule site Characteristics of sound waves Sound waves physically are characterized by the frequency and amplitude Frequency → pitch o Frequency of a sound wave is related to the pitch of the sound o Low frequency waves are related to low pitch sound o High frequency waves are related to high pitch sound o Human ear can perceive sounds within the frequency range 16-20 000 Hz o This range is rather small compared to animals o Animals can hear sounds until 100 000 Hz o Whales and butterflies up to 500 000 Hz Amplitude → loudness o Describes pressure difference between the maximal and minimum point o Psychophysiological amplitude of the sound is related to the loudness o Low amplitude sounds are soft sounds o High amplitude sounds are loud sounds o Human ear can hear sounds in between 0-120 dB o Amplitude is expressed in logarithmic units of pressure against reference pressure o Pref = 20 uPa and is considered the threshold pressure Loudness of sounds The human ear can differentiate sounds in between 0-120 dB o 0 decibel is threshold of hearing o 120 dB sounds become uncomfortable to listen to o 10 dB → just audible sounds o 20 dB sound of rustling leaves 30-60 dB → quiet sounds o Quiet sounds usually are produced in the workplace o Beginning from 30 dB in the library o To 60 dB in a busy office 60-90 dB → moderate intensity sounds o Moderate intensity sounds are caused by traffic 90-110 dB → very loud sounds o Can be produced by the airplane or train if you are standing very close to the takeoff place > 110 db → uncomfortable o Sounds greater than 110 dB can be heard in rock concerts, sports competitions, fire alarm or ambulance alarm > 85 dB → damage to hair cells in organ of Corti o People subjected to such sound intensities regularly have to wear personal hearing aids to protect the hearing system 2 Aurora Killi 26.02.22 o Hair cells in the inner ear do not regenerate o It they are lost some part of hearing is lost as well Logarithmic scale Hearing level measured in dB uses logarithmic scale The difference of pressure exerted from 0 to 120 dB varies 1 million times The human ear is actually very sensitive to sound o At 120 dB the pressure variation in the external environment is about 0.01% o At 0 dB it is 1 millionth part of it Human hair cells can determine very slight pressure differences in the external environment Components of the hearing sensory system Like other sensory systems, the hearing sensory system consists of three major parts 1. Receptors → hair cells o Receptors for hearing system are located in the inner ear o Receptors are hair cells located in the organ of Corti o Hair cells generate receptor potential which further triggers neurotransmitter release on the cochlear nerve endings 2. Conductive part → hearing pathway o If threshold is reached, action potentials are generated o Through the cochlear nerve they are conducted to the cochlear nuclei in the brain stem o From the cochlear nuclei, impulses are conducted to the superior olivary nuclei of both sides. o From there they go to the inferior colliculi, and then to medial geniculate bodies 3. Highest centers → superior temporal gyrus o From the medial geniculate bodies, impulses go to the superior temporal gyrus in the cerebral cortex where the primary auditory area is located o This area is responsible for perception of sound Air and bone conduction Sound wave conduction to the organ of Corti can be done through air and bone conduction Air conduction Bone conduction 1. Sound waves are conducted to the external 1. In bone conduction, external and middle ear auditory canal conduction is not important 2. Vibrations from the external auditory canal are 2. The vibrating object is in direct contact with conducted through the tympanic membrane to the skin and vibrates the bones of the skull the middle ear 3. Through the temporal bone, sound waves are 3. Ossicle chain in the middle ear conducts conducted to the fluid in the inner ear sounds waves to the oval window which 4. Sound waves reach the hair cells in the organ contacts with the fluid in the inner ear of Corti through the fluid 4. Through the fluid, sound waves are travels to 5. The middle ear is an important amplifier of the Corti organ hair cells that sense that sound sound → bone conduction usually transmits waves coming weaker sound from the same source than air 5. We perceive most sounds through air conduction conduction 3 Aurora Killi 26.02.22 Functions of the external ear Sounds are firstly conducted through the external ear Function Explanation Collection of sound waves Collection of sound waves from the external environment is done by auricula Auricula is also responsible for localization of sound source (especially is sound source is vertically displaced) Transmission of sound waves Sound waves from auricula are transmitted through the external auditory canal to the tympanic membrane Resonance function Auricula resonates to sound waves of 5000 Hz External auditory canal resonates to sound waves of 3000 Hz Sound waves are amplified in the external auditory canal on the way to the tympanic membrane Defense function Mostly related to glands in the wall of the outer third of the external auditory canal Desquamated epithelial cells and gland secretions make ear wax Ear wax moves slowly outwards from the external auditory canal Gland secretions are a little acidic → prevents bacterial growth, water, and insect entrance in external auditory canal Over-secretion of ear wax → obstruction o Good functions of ear wax are gone o Sound waves cannot penetrate as easy and reach the tympanic membrane Regulates humidity and Blood flow to the external auditory canal and tympanic temperature of the tympanic membrane keeps normal temperature of the tympanic membrane membrane and humidifies it This enables it vibration when sound waves come to the membrane Ossicle chain 1. When sound waves reach the tympanic membrane, it starts to vibrate and causes vibration of the ossicle chain 2. The ossicle chain consists of Malleus Incus Stapes (footplate of stapes is connected to the oval window) 3. Vibration of the ossicle chain, pushes the stapes footplate into the oval window, creating vibration which is transmitted through the fluid in the inner ear Functions of the middle ear Function Mechanism Sound transmission Sound transmission is done by the ossicle chain Ossicle chain joins the tympanic membrane and oval window membrane Amplification Difference in tympanic and oval window membrane surface area Done by two mechanisms o Causes the most amplification in the middle ear Total amplification → 22x 4 Aurora Killi 26.02.22 (22 * 1.3 = 22) o The tympanic membrane is connected to malleus followed by incus and stapes whose footplate contacts with the oval window membrane o Tympanic membrane surface area → 55 mm2 o Oval window membrane surface area → 3.2 mm2 o Surface area of tympanic membrane is 17x greater than oval window → sound waves amplified 17 times o Small vibrations of the tympanic membrane → great pressure rise in middle ear which is exerted against the oval window Lever system o Second amplification mechanism o There are two levers o Incoming lever of malleus o Outgoing lever of incus o Malleus lever is 1.3x > than incus lever o If the tympanic membrane moves, it moves the lever system against fulcrum (joining point of malleus and incus) o This exerts 1.3 times greater pressure on the oval window than the pressure exerted on the tympanic membrane o Ossicle chain can amplify sound waves 1.3 times o Total amplification in middle ear → 22x (17 * 1.3) Ossicle chain immobility In case the ossicle chain is not mobile anymore we lose 1.3x amplification This amplification loss will cause sound produced by speech to be perceived as whispering Person loses 20-40 dB amplification of the sound through the middle ear Stapes is substituted with an artificial one to prevent this amplification loss Defense function Majorly provided by two muscles located in the middle air that are activated by too loud sounds Impulses sent to the brainstem through the cochlear nerve are sent back to these muscles if the sound is too loud M. tensor tympani o Connected to the tympanic membrane o Innervated by the V cranial nerve (trigeminal nerve) o Contraction → moves tympanic membrane inwards into the middle ear M. stapedius o Connected to the footplate of stapes o Innervated by the VII cranial nerve (facial nerve, mandibular branch) o Contraction → moves stapes footplate out of the oval window and inwards into the middle ear If these muscles work simultaneously → ossicle chain becomes stiffer and cannot vibrate as much This system protects the inner ear from loud sound conduction Equilibration of pressure Tuba auditiva connects the middle ear and the nasal cavity Partial defense function It equilibrates pressure between atmospheric air and middle ear If Pat changes → tympanic membrane bulge inwards or outwards depending on where pressure is greater 5 Aurora Killi 26.02.22 To prevent this, tuba auditiva equilibrates pressure and enables vibration of the tympanic membrane Tuba auditiva is normally closed → opens due to yawning and swallowing The quick change in altitude causes the tympanic membrane to bulge inward into the middle ear and sometimes even rupture To prevent this, we should swallow saliva to open tuba auditiva multiple times and equilibrate pressure 6 Aurora Killi 26.02.22 Sound transmission in inner ear. Mechanism of activation of receptors Internal ear Stapes footplate beating on the oval window transmits sound waves into the cochlea Cochlea is spiral shaped and makes 2.5 turns around Cochlea has bone walls, and inside the bone there is a membranous cochlea that divides cochlea into 3 parts o Scala vestibuli where the sound waves travel from the footplate of stapes o Scala tympani where it returns back o Scala media which is related with the membranous cochlea Scala vestibuli & scala tympani Scala media Perilymph Endolymph Filled a fluid called perilymph Contains fluid called Perilymph is similar to extracellular fluid endolymph Rich in Na+ ions Rich in K+ ions Poor in K+ ions Greater [K+] concentration than intracellular fluid Secretion of endolymph Endolymph secretion depends on stria vascularis located in the lateral wall of scala media. Stria vascularis is able to concentrate K ions in the endolymph by several mechanisms 1. Hair cells release K+ ions into the perilymph where they are further transported to the spiral ligament 1. Fibrocytes accumulate K+ for transfer to intermediate cells 2. In the spiral ligament, fibrocytes take up K+ ions by two important 2. K+ channels in intermediate cell mechanisms generate endocochlear potential o Na+/K+ pump → pumps K into the cell 3. Marginal cell transfer from K+ from o Na+/K+/2Cl- symport → Na+ gradient is used to drive this intrastrial fluid to endolymph + 3. From fibrocytes, K are transported into intermediate cells through gap junctions 4. Intermediate cells secrete them into the intrastrial fluid via K+ channels 5. Marginal cells take them up via o Na+/K+ pump o Na+/K+/2Cl- symport 6. K+ ions diffuse out of the marginal cells → endolymph in high quantity 7. This potential of +80 mV and the little higher [K+] in endolymph → important for K+ influx into hair cells when sound waves come along Genetic diseases and drugs Genetic diseases o Defective K+ transporters → decreased K+ secretion in endolymph o This decreases electrical and chemical gradient for K+ influx into hair cells → deafness o Barter´s syndrome → defective Na+/K+/2Cl- symport in the ear causing deafness Loop diuretics o Blocks Na+/K+/2Cl- symporter o If person uses loop diuretics for longer time → part of hearing lost 7 Aurora Killi 26.02.22 o Hearing will regain back when the use of these drugs is stopped Antibiotics and anti-cancer treatment o Some antibiotic treatment and anti-cancer treatment can decrease K+ secretion in endolymph thus causing deafness Transport of endolymph The bony labyrinth is the bony outer wall of the inner ear and consists of o Vestibulum o Semicircular canals (superior, horizontal, posterior) o Cochlea At one end of each semicircular canal is ampulla Inside the bony labyrinth is the membranous labyrinth which is filled with endolymph The space between the bony and membranous labyrinth is filled with perilymph Endolymph in the membranous labyrinth comes from both o Hearing system (cochlea) o Vestibular system (semicircular canals and vestibulum) Endolymph from the membranous labyrinth is collected and transported via the endolymphatic duct into the enlargement called endolymphatic sac The endolymphatic sac lies below dura mater, and excess endolymph is able to absorb in its blood vessels Meniere´s disease If there is decreased endolymph removal from the inner ear, Meniere´s disease develops Causes of Meniere´s disease Viral and bacterial infection Autoimmune reactions Genetic causes of mechanisms related to reabsorption of endolymph Consequences Recurring vertigo o From vestibular system o Dizziness and feeling of spinning despite the person do not move Sensorineural hearing loss o From hearing system o Accumulation of endolymph in the membranous cochlea leads to the damage of hair cells Tinnitus o Threatening damage of outer hair cells cause their contraction which leads to tinnitus or ringing in the ear o The contraction and relaxation of external hair cells causes vibration of the endolymph o These vibrations are perceived by the inner hair cells, despite that there is no sound in the external environment Feeling of fullness in the ear o Due to increased pressure in the membranous labyrinth, experiences feeling of fullness in the ear Treatment We can use drugs to decrease K+ secretion (since reabsorption is decreased) Loop diuretic furosemide blocks the Na+/K+/2Cl- symport → decreased K+ transport to endolymph 8 Aurora Killi 26.02.22 We can also prescribe drugs that decreases o Dizziness o Nausea o Anxiety level by increasing blood flow to the brain to the endolymph might reabsorb quicker Sound transmission through the inner ear 1. When sound waves are transmitted through the middle ear, the foot of stapes starts vibrating perilymph 2. The acoustic inertia of fluid is lower than air → amplification in the middle air is important to transmit sound from air into fluid 3. When perilymph in scala vestibuli starts vibrating → pressure wave is transmitted along scala vestibuli to helicotrema, and then it travels back into the scala tympani 4. On the way back it vibrates the basilar membrane and then it disappears through the round window back into the middle ear Hair cells Organ of Corti is located on the basilar membrane of scala media It consists of four rows of hair cells o Outer hair cells of three rows o Inner hair cells of one row Each hair cells have stereocilia which projects outwards Stereocilia are organized in increasing length to one side of the hair cell Stereocilia are joined by tip links which enables coordinated movement of them Inner hair cells Important for sensory function Less numerous Most of afferent nerve fibers begin from the inner hair cells These cells are responsible for o Sound perception o Signal transduction Inner hair cells stereocilia are not connected to tectorial membrane o Their movement is rather dependent on the endolymph flow in the inner corner of scala media When stereocilia bend → depolarization and impulse transmission along afferent nerve fibers to the brain Outer hair cells Have very few afferent fibers, but most efferent fibers from superior olivary nuclei More numerous comes to outer hair cells o Large receptive fields o 100-150 hair cells send impulse to a single cochlear nerve fiber Outer hair cell stereocilia are connected to tectorial membrane o Their movement is dependent on interreaction with the tectorial membrane Motor protein prestin is located in the membrane of outer hair cells Depolarization 9 Aurora Killi 26.02.22 1. When outer hair cells depolarize due to stereocilia bending → prestin triggers hair cell contraction 2. Distance between tectorial and basilar membrane shortens 3. Endolymph flow to inner corner increases thus stimulating inner hair cells 4. In such a way, outer hair cells can amplify endolymph flow to stimulate inner hair cells Activation due to efferent fibers 1. Most of the efferent fibers that begin from superior olivary nuclei in the brain stem comes to the outer hair cells 2. Efferent fibers are activated due to loud sounds in which the superior olivary nucleus send impulses back to the outer hair cells to make them stiffer 3. The basilar and tectorial membrane complex becomes stiffer 4. This prevents high endolymph flow to the inner angle, thus protecting inner hair cells Frequency of hair cell contraction is not exactly the same as the sound frequency, but it tries to resemble the frequency rise and decrease Sound waves travel along the scala vestibuli and tympani When sound waves travel along scala vestibuli and tympani, basilar membrane moves up and down which also causes tectorial membrane movement Since both membranes move in a little bit different directions it causes deviation of stereocilia Basilar membrane moves up 1. Happens when stapes move out of the oval window (Pvestibuli < Ptympani) 2. Basilar membrane bulge upwards and pushes tectorial membrane up and a little bit out 3. Stereocilia bends towards the longest one 4. Bending towards the longest hair → activation of outer hair cells which stimulates their contraction 5. Contraction moves basilar and tectorial membrane closer to each other, stimulating endolymph flow in the inner angle → deviation of hair towards the longest of the inner hair cell (activation) Basilar membrane moves down 1. Happens when stapes moves into the oval window (Pvestibuli > Ptympani) 2. Basilar membrane bulges downwards and drags tectorial membrane little bit down and medially (inwards) 3. Stereocilia bends towards the shortest one → inactivation and relaxation of outer hair cells 4. Distance between the tectorial and basilar membrane increases → opposite endolymph flow bends inner hair cells stereocilia towards the shortest, decreasing their activity If stereocilia bends towards the longest one 1. Mechanosensitive K+ channels in the stereocilia open → K+ influx Stereocilia are connected by tip links that connected to provided by two mechanisms mechanosensitive K+ channels Chemical gradient o [K+] in endolymph → 150 mM/L 10 Aurora Killi 26.02.22 o [K+] in hair cell → 120 mM/L o K+ flows into the cell down the gradient Electrical gradient o Endolymph charge → +80 mV o Hair cell membrane potential → -70 mV o Potential difference of 150 mV drives K+ into the cell 2. Positive ion influx triggers depolarization 3. Voltage gated Ca2+channels open → Ca2+ influx 4. Neurotransmitter glutamate is released in the synapse with afferent nerve fibers 5. Glutamate triggers excitatory postsynaptic potential in the cochlear nerve 6. If threshold is reached → action potential generated and conducted to CNS If stereocilia bends towards the shortest one 1. Mechanosensitive K+ channels close → K+ influx ceases 2. K+ ions move out of the cell into the extracellular space, down the chemical gradient 3. Extracellular space is washed with perilymph 4. Ion outflux → hyperpolarization 5. Inhibition of neurotransmitter release → impulse generation is stopped Impulse conduction to the brain 1. Impulses are conducted to the cochlear nuclei through the cochlear nerve fibers 2. From the cochlear nuclei some impulse travel to Same side superior olivary nuclei Opposite side superior olivary nuclei 3. From olivary nuclei, impulses are conducted to the inferior colliculi which is responsible for head movement in the direction of sound 4. Through the medial geniculate bodies, impulses are conducted into the superior temporal gyrus where the primary auditory area is located 11 Aurora Killi 26.02.22 Coding of sound pitch, loudness, and localization Coding of sound parameters Parameter Coding Frequency Done by properties of cochlea Pitch o At the base of cochlea, the basilar membrane is narrow and stiff and vibrates maximally at high frequency sounds o At the apex of cochlea, the basilar membrane wide and lose and vibrates with greater amplitude at low frequency sounds High frequency sound → basilar membrane vibrates closer to the oval window and then sound wave disappears Medium frequency sound → wave travels forwards and basilar membrane vibrates in the middle of cochlea Low frequency sound → sound wave travels to the tip near helicotrema and basilar membrane vibrates with highest amplitude Different places of the basilar membrane send impulses through different nerve fibers into different places in primary auditory cortex o Low frequency sound → front part of primary auditory cortex o High frequency sound → posterior part of primary auditory cortex Amplitude High amplitude sound waves vibrate the basilar membrane with greater Loudness amplitude This triggers greater activity of hair cells → impulse frequency in afferent fibers increases Hair cells in the organ of Corti have different sensitivity o Soft sounds only activate the cells with highest excitability o Loud sounds activate more cells with higher threshold and summation of potential in the cochlear nerve can increase impulse frequency to the brain Auditory cortex translates loudness of sounds from impulse frequency o If high frequency impulses come → loud sounds o If low frequency impulses come → low sounds Localization Localization of sound or sound source Done through two mechanisms → time delay and loudness delay Time delay o Ear closest to the sound source receives sound quicker than the opposite ear o This time delay allows us to differentiate which side the sound comes from Loudness delay o If sound comes from the side, it is perceived louder in the closest ear while softer in the opposite ear Differentiation of time delay and loudness delay is done by superior olivary nuclei Time delay Medial superior olivary nucleus determine time delay Receives excitatory input from both ears Impulses from one ear alone is not enough to excite it If sound source is closer ipsilateral side (left) 12 Aurora Killi 26.02.22 o Signals from the left ear reaches medial superior olivary nucleus before signals from the right ear o Ipsilateral medial superior olivary nuclei will not be activated until threshold o No signals sent from the ipsilateral medial superior olivary nucleus to the brain, indicating that the sound source is closer to the ipsilateral side If sound source is closer to contralateral side (right) o Impulses from the right and left ear will arrive in the medial superior olivary nucleus at the same time o Actional potential is generated in the contralateral medial superior olivary nucleus o Impulses are transmitted to the highest centers of brain indicating that the sound source is closer to the contralateral side Loudness delay Lateral superior olivary nucleus determine loudness difference Ipsilateral auditory pathway works excitatory on the lateral superior olivary nucleus Contralateral auditory pathway works inhibitory on opposite side lateral superior olivary nuclei Sound source on ipsilateral side (left) o Ipsilateral signals excite lateral superior olivary nucleus and impulses are sent to the brain o Contralateral signals take longer to reach the nucleus and cause inhibition of it o Until inhibition takes place → signals from the ipsilateral side are sent to the brain that the sound source is closer to the ipsilateral ear Sound source on contralateral side (right) o Signals from both sides arrive in the opposite side lateral superior olivary nucleus at the same time o Excitation and inhibition happen simultaneously o Lateral superior olivary nucleus does not to transmit impulse to the highest centers of the brain → indicating that sound source is closer to the opposite ear Vertical localization discrimination The form of auricula reflects sound waves into the external auditory canal Ear compares the direct wave and reflected wave to localize the sound source o Reflected wave has long delay → sound comes from bottom o Reflected wave has short delay → sound comes from front or above Vertical localization can be done with one ear 13 Aurora Killi 26.02.22 Audiometry, Weber and Rinne test. Conduction and neural deafness Isophones Sensitivity of hair cells in the range of frequencies is not the same Hair cells are most sensitive in the range of 1000-6000 Hz o If sound frequency decreases → threshold for hearing increases o If sound frequency > 6000 Hz → threshold for hearing increases The lines presented in the chart joins together with the subjectively the same intensity of sound which is perceived by the person 0 dB sound at 1000 Hz frequency causes the same subjective intensity as 65 dB sound at 40 Hz frequency Isophones are lines that join different sound frequencies at which the person subjectively perceives equal sound intensity Audiogram Testing hearing threshold → we express the intensities of sound waves that the person can hear against the zero isophone Hearing thresholds are normally represented in opposite 0 dB intensity in audiogram corresponds to zero phone of zero intensity line Threshold of hearing o 0 dB sound at 1000 Hz gives the same subjective intensity perception as 65 dB at 40 Hz o This is considered as the hearing threshold Audiometry test → determine hearing threshold of the person Hearing thresholds Normal hearing threshold should be < 20 dB Mild hearing loss: 20-40 dB Moderate hearing loss: 40-55 dB Moderately severe hearing loss: 55-70 dB Severe hearing loss: 70-90 dB Profound hearing loss: > 90 dB Air and bone conduction These curves generally show the air conduction curves o If sound waves are produced in air and transmitted through the outer and middle until the inner ear and hair cells Bone conduction is generally about 40 dB higher than the air conduction o No amplification of sound waves in the middle ear o We can use the same characteristics of hearing loss to characterize thresholds obtained in bone conduction Audiogram and age Threshold for hearing is differs with age → mostly higher frequencies are affected 20 years → hearing threshold < 20 dB 14 Aurora Killi 26.02.22 90 years → some people cannot hear 8000 Hz Audiometry tests Rinne´s test Compares air and bone conduction 1. A vibrating tuning fork is placed over the mastoid bone behind the ear so sound waves are transmitted through the bone and activates hair cells in organ of Corti 2. We ask the person to tell when he/she stops hearing 3. When the person stops hearing sounds the tuning forks is moved close to the external acoustic mellitus and ask the person again to tell when he/she stops hearing 4. Since air conduction usually is better than bone conduction, the person should still hear these sound waves going through the external, middle ear into the internal ear If air conduction > bone conduction → test is positive and indicates normal hearing If bone conduction > air conduction (due to obstruction of sound waves in the ear) → indicates conductive hearing loss Weber test 1. A vibrating tuning fork at the middle of the scalp 2. We ask the person in which ear he or she hears the sound best 3. Normally, there should not be a difference between the right and left side 4. If person hears sound better in one ear, it can indicate two things Conductive loss → in the ear that heard sound better o If the person hears sound better in the left ear, it can indicate conductive hearing loss in the left ear o In conductive hearing loss there are problems with sound wave conduction through the external and middle to the inner ear in the same side o If no environmental sounds or sounds of less intensity comes to the internal ear these hair cells become more sensitive, thus sound waves conducted through the bone causes greater activity in the same side Sensorineural loss → in the opposite ear o If the person hears sound better in the left ear, it can indicate sensorineural hearing loss of the right hear o The hearing loss is connected to the damage of hair cells Otoacoustic emission Test to determine hearing function Principle → when sound waves are transmitted to the hair cells they start contracting and emitting sound waves back A sensor is placed in the external ear, and it emits sound waves that are transmitted to the inner ear Hair cells respond to the sound waves by transmitting them backwards A microphone inside the sensor records the acoustic emissions and analyze them o If hair cells are healthy → emission present and sent back to the microphone o If hair cells are damaged → emission is lost and indicates sensorineural hearing loss 15 Aurora Killi 26.02.22 Deafness Conductive hearing loss Sensorineural hearing loss Sound waves does not reach inner ear Sound waves not processed correctly Sound waves are not conducted from the Inability to process sound waves that are external environment to hair cells in inner ear transmitted and sent to the cortex Causes Causes o Accumulation of ear wax in the outer o Damage of hair cells until the cerebral auditory canal cortex o Perforated tympanic membrane o It can be damage of hair cells caused by o Problems with ossicle chain due to loud sounds recurring inflammations in the middle ear o Problems with cochlear nerve of further the ossicle chains become immobile or conductive pathways to the cerebral there is excessive tissue growth around the cortex joints so sound waves is not properly o Damage of cerebral cortex itself transmitted Treatment of hearing loss External auditory devices → conductive hearing loss o These can be used to amplify the sound waves transmitted through the external auditory canal Cochlear implants → sensorineural hearing loss o Cochlear implants consist of two parts o The first is external part which receives sound waves and sends them to the internal part which is implanted in the persons skull. o The internal part consists of a receiver and stimulating electrode which is leading to the activation of cochlear nerve fibers directly Direct stimulation of cochlear nerve (sensorineural) o Most sensorineural loss problems are connected to damage of hair cells rather than nerve fibers innervating them o Direct stimulation of cochlear nerve from an electrode might help the person perceive sounds from the external environment 16 Aurora Killi 26.02.22 Equilibrium sensory system. Receptors in semicircular canals; adequate stimuli and mechanism of activation The bony labyrinth is the bony outer wall of the inner ear and consists of o Vestibulum o Semicircular canals (anterior, horizontal, posterior) o Cochlea At one end of each semicircular canal is ampulla Inside the bony labyrinth is the membranous labyrinth which is filled with endolymph The space between the bony and membranous labyrinth is filled with perilymph Vestibular sensory system The equilibrium sensory system consists of three parts Receptor → hair cells in the semicircular canals and vestibulum o Receptors are located in o Ampulla part of semicircular canals o Utricle and saccule of vestibulum (macula parts) o Receptor cells are hair cells similar to those in hearing system Conductive part → vestibular pathway o Begins from hair cells o Sends impulses to multiple subcortical centers on its way to highest center Centers → temporoparietal, somatosensory, and premotor cortex o The highest centers are responsible for conscious equilibrium sense and are located in the cerebral cortex o These centers are in the temporoparietal, somatosensory, and premotor cortex which are responsible for conscious sensation of equilibrium In the hearing sensory system there are only stereocilia Receptors Both in ampulla and macula Hair cells in the vestibular system have o Kinocilium → one true hair o Stereocilia → other hairs Hairs are placed in the direction of the longest hair Apical surface of hair cells is covered by a gelatinous mass called o Capula in semicircular canals o Otolithic membrane in vestibulum We have two types of hair cells Type I hair cells Type II hair cells Have a wide postsynaptic membrane of the Rather small area covered by afferent fibers vestibular nerve afferent fibers Great in convergence to a single nerve fiber Efferent nerve fibers synapse with the from multiple receptor cells postsynaptic membrane of the vestibular nerve These cells are more influenced by the efferent afferent fiber nerve fibers which are beginning from the brain stem 17 Aurora Killi 26.02.22 Mechanisms of activation of receptors Tips of hairs are connected with tip links that regulates mechanosensitive K+ channel opening. Stereocilia and kinocilia movement is not by sound waves but due to position of the head If hairs bend towards longest hair If hairs bend towards shortest hair 1. Mechanosensitive K channels open → K influx 1. Mechanosensitive K+ channels close → K+ influx + + due to chemical and electrical gradient ceases while K+ outflux through K+ channels to 2. Depolarization of hair cell perilymph 3. Voltage gated Ca channels open → Ca influx 2. Hyperpolarization of hair cell 2+ 2+ 4. Neurotransmitter is released on the vestibular 3. Neurotransmitter release is inhibited, and nerve fibers and binds to postsynaptic vestibular nerve fibers are not activated membrane 4. Action potentials are not generated 5. This triggers excitatory postsynaptic potential 5. The mechanism of activation is the same as in generation the ear, however vestibular receptors are bent 6. If threshold is reached → action potential in not by sound waves, but by the position and afferent nerve fibers change of position of the head Receptors in semicircular canals Semicircular canals are located in three perpendicular planes Horizontal canal o Located about 30 degrees deviated from the horizontal plane o Stimulated by rotation of the head Anterior and posterior canals o Located 45 degrees deviated from the sagittal plane o Anterior canal responds to nodding of head o Posterior canal responds to head flexion to the side Our movements are usually not parallel to these three places The semicircular ducts are the part of the By moving → two or even all three canal receptor cells are membranous labyrinth that are contained in stimulated in different degrees the semicircular canals of the osseous Semicircular canals of the bony labyrinth are filled with labyrinth, concerned with rotational perilymph equilibrium. Like the other parts of the membranous labyrinth, the ducts contain The membranous canals or duct lies within the bony endolymph and are surrounded by perilymph labyrinth and are filled with endolymph Mechanism of activation of receptors in semicircular canals Receptors are located on crista ampullaris in the ampullar part of the membranous semicircular canals Hairs of these receptors are embedded in a gelatinous mass called cupula Cupula has the same density as endolymph → not deviated by the gravity These receptors are sensitive to rotational acceleration Starting rotation 1. When we rotate the head, the membranous and bony labyrinth rotates in the direction of the head movement 2. Endolymph has rather low inertia and will be displaced in the opposite direction of the movement 3. Endolymph will displace Capula to the opposite side of rotation as well which will bend stereocilia to the opposite side of head movement 4. In hair cells whose stereocilia bend towards kinocilium 18 Aurora Killi 26.02.22 Mechanosensitive K+ channels opening K+ influx Depolarization Neurotransmitter release Excitatory postsynaptic potential Action potential in the cochlear nerve Prolonged rotation 1. After about 15 seconds of constant speed rotation, endolymph accelerates and acquire the same rotational speed and direction as the membranous labyrinth 2. Capula is no longer deformed, and hair cells are not bent 3. Hair cells stop sending impulses to the CNS Stopping rotation 1. When head rotation has stopped, membranous labyrinth stands still where our head is 2. Endolymph does not stop right away due to low inertia 3. Capula is displaced in the direction of the previous rotation 4. Hair cells are bend in the opposite direction as before and this causes Mechanosensitive K+ channels close Hyperpolarization of hair cells Inhibition of neurotransmitter release No impulse conduction to the CNS Rotational acceleration in opposite ear The rotational acceleration is perceived differently in the opposite ear If we rotate to the left o Left ear → hair cells in left horizontal canal are activated and hairs are bent towards the longest one o Right (opposite) ear → hairs in the semicircular canal are bent towards the shortest one so they are inactivated. Impulses will not be sent to the CNS 19 Aurora Killi 26.02.22 Equilibrium sensory system. Receptors in vestibulum; adequate stimuli and mechanism of activation Receptors in maculae Located in the vestibulum and they perceive Gravity o We can sense if we are sitting with the head facing up or down o If our head is straight or not Linear acceleration o Movement forwards, backwards, sideways, up, and down o Receptors are located in two planes o Utricle receptors are located in the horizontal plane and sense linear acceleration in horizontal plane (forwards, backwards, sideways) o Saccule receptors are located in the vertical plane and sense linear acceleration in vertical plane (up, down) Stereocilia of receptors in each receptor area of macula are not directed in the same direction o In saccule → the kinocilium is located outwards from the midline o Stereocilia length increases outwards from the midline o At the end of longest stereocilium is the kinocilium o In utricle → the kinocilium is located on the midline o Hair cell length changes in opposite direction than in saccule o Stereocilia length increases towards the midline Otolithic membrane o Consist of otoliths or calcium carbonate crystals o Otolithic membrane is denser than surrounding endolymph and will bend the hairs on the receptors o Since the receptors in macula are located in opposite directions according to their hair length, some receptors will be activated while others inactivated o This membrane helps us to determine our head position (even if eyes are closed) Mechanism of activation of macula receptors The otolithic membrane can be displaced by linear acceleration If we move forwards → otolithic membrane with low inertia will displace hairs of hair cells in the opposite direction (backwards) If we stop movement → otolithic membrane does not stop immediately thus displacing the hair in the direction of the previous movement (forwards) In such a way we can determine the direction of movement o If hairs bend towards kinocilium → mechanosensitive K+ channels opens and depolarization triggers neurotransmitter release to activate the vestibular nerve that conducts impulses to the brain o If hairs bend towards shortest stereocilium → mechanosensitive K+ channels close, and hyperpolarization inhibits neurotransmitter release and impulse transmission to the brain Otoliths in semicircular canals Otoliths can separate from the otolithic membrane and get free This happens more often for older people Head movements causes free otoliths to float with the endolymph to the semicircular canals 20 Aurora Killi 26.02.22 Receptors in the semicircular canals are not normally sensitive to gravity force Otoliths displaces the hairs in crista ampullaris and activates these receptors Person becomes dizzy because the brain receives signals that the person is rotating To remove the dizziness, the person can perform certain movements of the head to move the otoliths (debris) out of the semicircular canals 21 Aurora Killi 26.02.22 Vestibular reflexes. Vestibulo-ocular reflexes Vestibular pathways 1. Receptors in semicircular canals and macula are activated due to K+ influx in hair cells 2. Neurotransmitter release activates vestibular nerve 3. Vestibular nerve conducts impulses from all vestibular receptors to the vestibular nuclei in the brain stem which further sends impulses to… Motor neurons of extremities and neck muscles o To adjust the tonus of skeletal muscles according to our equilibrium o Especially extensor muscle tone is increased to support the body against gravity Motor neurons of eye movement o To control eye movement Cerebellum o Centers of equilibrium control are mainly located in cerebellum o These centers precisely control our equilibrium unconsciously o Damage to cerebellum → unconscious equilibrium control is lost o Conscious equilibrium control is not very precise → greater deviation from vertical position will be needed for us to consciously sense it and regulate muscle tone Cerebral cortex o Through the thalamus, some impulses reach the cerebral cortex which provides o Conscious sensation of equilibrium o Conscious sensation of movement o Primary somatosensory cortex and postcentral gyrus o This is where the hand and face areas are located o Their stimulation leads to sensation of movement o Posterior parietal cortex and parieto-insular cortex o Spatial orientation of the body and movement of the body in the space o We can perceive where we are moving, which direction, and what we are trying to achieve with our body posture o Premotor cortex o This center together with the eye control centers keeps the eye and body movements coordinated Vestibulo-ocular reflex The vestibulo-ocular reflex is the connection between vestibular receptors and eye movements It is important to keep the eyesight on one object while the head moves In general, this reflex causes eye movement in the opposite direction to the head rotation This is achieved by the interplay between the vestibular system and two nerves that control eye movement o Abducens nerve (VI) → controls lateral rector muscles contraction o Oculomotor nerve (III) → controls medial rector muscles contraction Mechanism of reflex 1. When we turn our head to the left → left semicircular canal activates and send impulses to the vestibular nuclei in the left side 2. The left vestibular nucleus causes 22 Aurora Killi 26.02.22 Excitation of contralateral abducens nucleus → lateral rectus contraction in right eye Excitation of ipsilateral oculomotor nucleus → medial rectus contraction in left eye Inhibition of ipsilateral abducens nucleus → relaxation of lateral rectus for left eye, relaxation of medial rectus for the right eye 3. By rotation of head to the left side both eyes turn to the right For left eye (ipsilateral) o Contraction of medial rectus o Relaxation of lateral rectus For right eye (contralateral) o Contraction of lateral rectus o Relaxation of medial rectus 4. Both eyes turn in the opposite direction of rotation. The reflex is important to provide good vision while the head moves Nystagmus Through this reflex, the eyes fixate to an object while the head moves in a direction This reflex can only keep the eyesight on the object for a limited time o If head rotation persists → vestibular-ocular reflex is inhibited to correct the vision for the next object in the visual field o Eyes will therefore rapidly move to left to the next object and then slowly move to the right side while fixating the object o This is called nystagmus Physiological o When we are looking out of the window of a moving car o The vestibulo-ocular reflex slowly turns the eye in the opposite direction of the movement, then a rapid shift to the next object Clinically o To test the vestibular receptor, nuclei, and eye control nuclei function in concert with the stimulation of vestibular receptors we can perform the caloric test Caloric test We irrigate the external auditory canal with cold or warm water o If the person has a perforated tympanic membrane air can be used instead The temperature change in the external auditory canal triggers endolymph flow in horizontal semicircular canal Cold and warm water stimulates endolymph flow in opposite directions and thus nystagmus is observed in different directions o Cold water stimulates nystagmus to the opposite side o Warm water stimulates nystagmus to the same side If nystagmus is observed in the eyes in response to caloric stimulation → horizontal semicircular canal works nicely 23