CNS Pharmacology III - PDF
Document Details
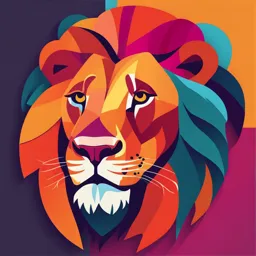
Uploaded by SurrealCatharsis
Ankara Medipol University
Zubeyir Elmazoglu
Tags
Summary
This document is a set of lecture notes covering CNS pharmacology and neurotransmission. The content explores neurotransmitters, their functions, and related processes in the central nervous system.
Full Transcript
PHARMACOLOGY III CNS PHARMACOLOGY Asst. Prof. Zubeyir Elmazoglu Department of Pharmacology, Faculty of Pharmacy Ankara Medipol University Ankara-2023 CNS The central nervous system (CNS) consists of the brain and spinal cord. Sensory information arrives to the CNS from the special senses and p...
PHARMACOLOGY III CNS PHARMACOLOGY Asst. Prof. Zubeyir Elmazoglu Department of Pharmacology, Faculty of Pharmacy Ankara Medipol University Ankara-2023 CNS The central nervous system (CNS) consists of the brain and spinal cord. Sensory information arrives to the CNS from the special senses and peripheral nerves and is integrated with memories and internal drive states to generate cognitive, emotional, and motor (behavioral) responses. This process occurs because of the complex interplay of neurotransmitters and neuromodulators acting on their receptors to excite or inhibit CNS neurons. In persons with brain disorders, structural or functional disturbances of CNS processing produce aberrant cognitive, emotional, or motor responses. Brain disorders are seen in association with a variety of disease processes, including degenerative, ischemic, and psychological disturbances. CNS Neurotransmitters are synthesized in neuronal cell bodies or terminals, and they are stored in neuronal vesicles until they are released into a synapse. The release of neurotransmitters is activated by membrane depolarization and calcium influx into the cell. Calcium evokes the interaction of storage vesicle proteins and membrane-docking proteins, leading to vesicle fusion with the membrane and exocytosis of the neurotransmitter After exocytosis, the neurotransmitter may activate presynaptic and postsynaptic receptors. A neurotransmitter’s action is then terminated either by its reuptake into the presynaptic neuron or by its degradation to inactive compounds, with degradation catalyzed by enzymes located on presynaptic and postsynaptic neuronal membranes or within the cytoplasm. Neurotransmitters can also diffuse from the synapse of their origin to affect neurons in the surrounding vicinity. In this way, different neurotransmitters released from different types of neurons form a chemical milieu The net influence of the chemical milieu on neurotransmission depends on the concentrations of the excitatory and inhibitory neurotransmitters acting at a particular synapse. NEURONAL TRANSMISSION Excitatory and Inhibitory Neurotransmission CNS neurotransmitters can evoke either an excitatory or an inhibitory synaptic membrane potential and trigger effects at presynaptic and postsynaptic sites on target neurons. If an excitatory postsynaptic membrane potential (EPSP) reaches firing threshold, an action potential is conducted along the dendritic and axonal membrane, evoking the release of a neurotransmitter from the nerve terminal An inhibitory postsynaptic membrane potential (IPSP) hyperpolarizes the neuronal membrane and inhibits the firing of action potentials. Depending on whether a presynaptic membrane potential is excitatory or inhibitory, it will increase or decrease the release of a neurotransmitter from the neuron. Presynaptic receptors, also called autoreceptors, can also be coupled with cyclic adenosine monophosphate (cAMP) or other second messengers that modulate neurotransmitter release EPSP VS IPSP EPSP VS IPSP Interaction of multiple neurotransmitters at a particular site in a neuronal tract enables the complex interplay of various neuronal systems and contributes to the wide range of functional expression exhibited in the CNS. example, inhibition of the release of an inhibitory neurotransmitter will actually increase neurotransmission in the target neuron. Similarly, drugs can act in complex ways to affect neurotransmission. For Ethanol (ethyl alcohol), for instance, can diminish the inhibitory influence of the cerebral cortex on certain human behaviors, thereby increasing drug-induced behaviors, a phenomenon called behavioral disinhibition. Ethanol and other CNS depressants, initially or at low doses, exert their effects on the smaller and more numerous inhibitory neurons, creating disinhibition via excitation owing to removal of inhibitory neurotransmitters. At higher doses, larger excitatory neurons are inhibited, and profound depression of CNS activity can occur Pathways in the central nervous system. A shows parts of three relay neurons (blue) and two types of inhibitory pathways, recurrent and feed-forward. The inhibitory neurons are shown in gray. B shows the pathway responsible for presynaptic inhibition in which the axon of an inhibitory neuron (gray) synapses on the axon terminal of an excitatory fiber (blue). FAST VERSUS SLOW SIGNALS Neurotransmitters in the CNS can be characterized as slow or fast, depending on the receptors activated and the persistence of signal transduction pathways. The best examples of fast neurotransmitters are gammaaminobutyric acid (GABA) and glutamate acting at ligand-gated ion channels. Binding of these amino acid neurotransmitters directly to subunits of the ion channel protein directly initiates ion flow with a signal that lasts for only a few milliseconds. Examples of slow neurotransmitters are norepinephrine and serotonin acting at G protein–coupled receptors. These activated G protein–coupled receptor proteins initiate a slower, multistep process with alterations in second messengers and membrane effects that can last from many milliseconds to as long as a second. A slow (long-acting) signal can influence the overall tone of a neuron because it can modulate the signals of several other fast neurotransmitters acting on the same neuron. For this reason, slow neurotransmitters can also be called neuromodulators. NEUROTRANSMITTERS AND RECEPTORS NEUROTRANSMITTERS AND RECEPTORS NEUROTRANSMITTERS AND RECEPTORS The receptors can be divided into two basic groups: ionotropic receptors, also called ligand-gated ion channels, which are directly associated with ion channels, and metabotropic receptors, which are typical G protein coupled receptors The mechanisms of signal transduction for neurotransmitters in the CNS are similar to those for neurotransmitters in the autonomic nervous system. The activation of ionotropic receptors alters chloride, sodium, potassium, or calcium influx, thereby evoking excitatory or inhibitory membrane potentials. The linkage of metabotropic receptors with G proteins leads to activation or inhibition of adenylyl cyclase and alteration in the levels of intracellular cAMP, or activation of phospholipase C and the formation of inositol triphosphate and diacylglycerol. Metabotropic receptor activity can also modulate ion channel activity via second messengers (most notably calcium) that activate protein kinases responsible for the phosphorylation of ion channels. NEUROTRANSMITTERS AND RECEPTORS Acetylcholine Acetylcholine, synthesized from acetyl coenzyme A and choline, is degraded to acetate and choline by the enzyme acetylcholinesterase Acetylcholine receptors (also known as cholinergic receptors) consist of two main types: muscarinic receptors and nicotinic receptors. In the CNS, acetylcholine acts as an excitatory or inhibitory neurotransmitter in a number of neuronal tracts, including those that innervate the hippocampus, cerebral cortex, and basal ganglia. These tracts participate in memory, sensory processing, and motor coordination, respectively. NEUROTRANSMITTERS AND RECEPTORS Gamma amino butyric acid (GABA) The most ubiquitous inhibitory neurotransmitter in the brain and spinal cord is GABA, which is biosynthesized from glutamate, the most abundant excitatory amino acid. Its receptors are the ionotropic GABAA receptors and the metabotropic GABAB receptors. Most drugs affecting GABA neurotransmission primarily activate or inhibit the GABAA-chloride ion channel complex. This ion channel complex contains receptors for several types of drugs, including the benzodiazepines and barbiturates, general anesthetics, and alcohol . Drugs acting at the GABAB receptor are used to control spasticity . The functions of GABA include regulation of neuronal excitability throughout the CNS and motor coordination GABA RECEPTORS NEUROTRANSMITTERS AND RECEPTORS Glycine Glycine is a major inhibitory transmitter in the spinal cord. Its strychnine-sensitive receptors are coupled with the chloride ion channel, and activation of these receptors leads to membrane hyperpolarization. The inhibitory actions of glycine are potently antagonized by the alkaloid strychnine, a convulsant poison used as a rodenticide. Strychnine poisoning causes disinhibition of motoneurons, leading to convulsions and death through respiratory failure. Glycine also acts as a co-agonist to the excitatory amino acid glutamate at N-methyl-d-aspartate (NMDA) receptors by binding to an allosteric strychnine-insensitive site. Taurine is a sulfur-containing amino acid postulated to act as a neurotransmitter or neuromodulator in the CNS. Taurine is believed to activate both strychnine-sensitive and strychnine-insensitive types of glycine-binding sites. NEUROTRANSMITTERS AND RECEPTORS Glutamate and Aspartate Glutamate and aspartate are acidic amino acids that function as excitatory neurotransmitters throughout the CNS. Their ionotropic receptors consist of three types that differ in their subunit composition and are named for drugs that show the most selectivity for each type: NMDA, AMPA (amino-3-hydroxy-5-methyl-4isoxazole propionate), and kainate receptors. These receptors are excitatory because their associated ion channels allow the flow of sodium or calcium ions into neurons, causing depolarization and firing of action potentials. Metabotropic glutamate receptors consist of two main classes that are either coupled to phospholipase C and intracellular calcium signaling or are negatively coupled to adenylyl cyclase and decrease cAMP. Glutamate and aspartate participate in the long-term potentiation needed for learning and memory and have a role in neuronal toxicity and apoptosis (cell death) evoked by trauma and ischemia. Antagonists of these excitatory amino acid receptors are used in the treatment of seizures and may find use in blocking the overexcitation of neurons that occurs after stroke and in other disorders NEUROTRANSMITTERS AND RECEPTORS Dopamine Dopamine is a major CNS neurotransmitter that binds to five types of dopamine receptors. The D1 and D5 receptors activate adenylyl cyclase, thereby increasing cAMP levels. In contrast, the D2, D3, and D4 receptors inhibit adenylyl cyclase and decrease cAMP levels. Levodopa, clozapine, and other drugs have effects on various steps in the synthesis and metabolism of dopamine. Dopamine is found in several neuronal tracts originating from the ventral tegmental area, plays a significant role in behavioral and drug reinforcement, and regulates emesis (vomiting), prolactin release, mood states, motor coordination, and olfaction. Its degradation by monoamine oxidase results in the formation of homovanillic acid, a metabolite that is subsequently excreted in the urine Inhibitors of monoamine oxidase increase dopamine levels in the brain and are used for the treatment of parkinsonism. NEUROTRANSMITTERS AND RECEPTORS Norepinephrine Norepinephrine, which is formed from dopamine, is degraded by monoamine oxidase and catechol-O- methyltransferase to a number of metabolites. The major metabolite excreted in the urine is 3-methoxy- 4-hydroxymandelic acid, or vanillylmandelic acid. The receptors for norepinephrine are α-adrenoceptors and β- adrenoceptors (also known as α and βadrenergic receptors) Norepinephrine is associated with several neuronal tracts projecting from the locus ceruleus in the medulla (brainstem) to the thalamus, cerebral cortex, cerebellum, and spinal cord, and it is found in tracts projecting from the midbrain to the hypothalamus. This ubiquitous neurotransmitter participates in the regulation of anxiety, cerebellar function, learning, memory, mood, sensory processing (including pain), and sleep. Drugs can alter norepinephrine neurotransmission by activating or blocking its receptors or by inhibiting its presynaptic neuronal uptake. NEUROTRANSMITTERS AND RECEPTORS Serotonin Serotonin, also known as 5-hydroxytryptamine (5-HT), is synthesized from tryptophan, is degraded to 5- hydroxyindoleacetic acid, and functions as both an excitatory and inhibitory neurotransmitter. Serotonin is found in neuronal tracts projecting from the raphe nuclei in the medulla to many other parts of the brain. These tracts are involved in emotional processing and pain processing and have an effect on appetite, mood, sleep, and hallucinations. Serotonin (5-HT) acts on more than a dozen types of receptors; all of them are metabotropic receptors, except for the 5-HT3 receptor, which is a ligand-gated ion channel protein. In most areas of the brain , serotonin has an inhibitory effect mediated by the 5-HT1A receptor, decreasing cAMP hyperpolarization. levels and increasing potassium conductance, causing membrane Drugs can affect serotonergic function by stimulating or blocking 5-HT receptors or by blocking serotonin reuptake NEUROTRANSMITTERS AND RECEPTORS Histamine Histamine is a neurotransmitter found in hypothalamic neurons that project to all the major parts of the brain, including the cerebral cortex. It is involved in the regulation of the sleep-wake cycle, cardiovascular control, regulation of the hypothalamic-pituitary-adrenal axis, learning, and memory. Histamine acts on two types of receptors in the brain, H1 and H3, with action on a third type, H2, in the periphery. All histamine receptors are metabotropic, with H1 receptors coupled to the phospholipase C pathway and H3 receptors negatively coupled to adenylate cyclase. H3 receptors were originally discovered as auto receptors on histaminergic neurons but are now known to also inhibit the release of other neurotransmitters. CNS histamine receptors are not currently considered a major site of therapeutic drug action, but antagonism of H1 receptors is partly responsible for the drowsiness and sedation caused by some antihistamines. NEUROTRANSMITTERS AND RECEPTORS Neuropeptides A number of peptides function as slow neurotransmitters or neuromodulators in the CNS. neuropeptides are synthesized in neuronal cell bodies and are then transported to nerve terminals for release. Once released, they are metabolized by various peptidases, but they do not undergo presynaptic reuptake. Some of the neuropeptides are released as co-transmitters with other, nonpeptide neurotransmitters. The co-transmitter neuropeptides usually serve to amplify or prolong the effects of these other neurotransmitters One of the first classes of neuropeptides discovered are the endogenous opioid peptides (endorphins), which include met-enkephalin, dynorphin, and β-endorphin. The opioid peptides inhibit pain transmission in the spinal cord and midbrain, and, like morphine, act on opioid receptors. A second important group consists of tachykinins, which include neurokinins A and B, and substance P. Neurokinins modulate cardiovascular and behavioral responses to stress, and substance P participates in pain processing, emesis, and anxiety OTHERS Nitric oxide is a gas formed from arginine by calcium calmodulin– stimulated nitric oxide synthase in CNS neurons. Evidence suggests that nitric oxide acts as a retrograde neurotransmitter in that it is released by the postsynaptic neuron and diffuses to the presynaptic terminal, where it facilitates future neurotransmitter release by elevating levels of cyclic guanosine monophosphate (cGMP). Several recently discovered appetite-regulating peptides function as neuromodulators in the CNS. These include ghrelin, a peptide synthesized in the stomach and hypothalamus, and neuropeptide Y and orexin, contained in neurons in the lateral hypothalamus.All three peptides stimulate food intake and increase body weight in animal models. Conversely, leptin is a peptide hormone secreted from fat cells that acts on the brain to reduce food intake and increase peripheral energy expenditure OTHERS Purines that can serve as neurotransmitters include adenosine and adenosine triphosphate (ATP). Adenosine activates specific receptors identified as A1, A2, and A3 receptors. Activation of A1 and A3 receptors increases cAMP formation, whereas activation of A2 receptors inhibits cAMP formation. The role of adenosine as a central neurotransmitter is not clearly established, but inhibition of A2 receptors by methylxanthines (e.g., caffeine) causes CNS stimulation. ATP acts on both ionotropic receptors, called P2X receptors, and metabotropic P2Y receptors. Endocannabinoids NEURONAL SYSTEMS IN THE CENTRAL NERVOUS SYSTEM Cognitive Processing Cognitive processing occurs in prefrontal cortical structures, where sensory information is integrated with past experience and interpreted in a manner that can result in thoughts and behavioral action. The neuronal systems involved in cognitive processing include association fibers that arise from areas throughout the brain and converge on the anteromedial frontal, orbital frontal, and cingulate areas of the prefrontal cortex. Cognitive processing uses memory and is influenced by emotions. At the same time, emotions are largely derived from past experience and cognition. Delirium is a general term that refers to disorders of cognitive processing, and one of the manifestations of schizophrenia is impaired cognitive processing. Drugs that affect cognitive processing include antipsychotics, CNS stimulants, hallucinogens, and sedative-hypnotics NEURONAL SYSTEMS IN THE CENTRAL NERVOUS SYSTEM Memory Memory is the ability to recall events and integrate them into cognitive processing, emotional processing, and ongoing motor activities. One form of memory, called procedural memory, is used to recall a set of practiced motor actions (e.g., riding a bicycle or typing on a keyboard), and it involves the interaction of limbic structures, the cerebellum, and the basal ganglia. Another form of memory, called declarative memory, involves thoughts and associations that may be used to determine future actions. Declarative memory involves neuronal tracts in the hippocampus, amygdala, thalamus, and neocortex. Dementia is a term used to describe a number of memory disorders, including Alzheimer disease. The involvement of the basal ganglia in procedural memory may explain why some patients with Parkinson disease have difficulties with practiced motor actions. Drugs that affect memory include the cholinesterase inhibitors and CNS depressants such as benzodiazepines. NEURONAL SYSTEMS IN THE CENTRAL NERVOUS SYSTEM Emotional Processing Emotional processing is responsible for the generation of emotions such as anger, anxiety, fear, happiness, love, and sadness. These emotions represent the conscious perception of neuronal activity originating in the limbic system, including the hypothalamus, amygdala, septum, hippocampus, and mammillary bodies, as well as the cingulate and entorhinal portions of the frontal lobe cortex. Emotions contribute to a state of mental preparedness for anticipated future activities. Disorders in which emotional processing is defective include anxiety states, mood disorders, and schizophrenia. Drugs that affect emotional processing in the limbic system include anxiolytic (antianxiety) drugs, antidepressants, antipsychotics, CNS stimulants, opioids, and all drugs that produce drug dependence. Hence, most CNS drugs have some effect on emotional processing. NEURONAL SYSTEMS IN THE CENTRAL NERVOUS SYSTEM Sensory Processing Sensory processing involves neuronal tracts that perceive external stimuli and transmit that information to the brain. These include the sensory systems responsible for vision, hearing, olfaction, touch, and pain. The spinothalamic tracts relay touch and pain sensations to the thalamus, which projects this information to the cortex. The brainstem region known as the reticular formation plays a significant role in filtering sensory information before it is relayed to the thalamus and hypothalamus and eventually to the cortex. The reticular formation includes the locus ceruleus and raphe nuclei, whose neurons release norepinephrine and serotonin, respectively, and play an important role in determining the level of consciousness, sleep, and wakefulness. The cortex of the parietal and occipital lobes and part of the temporal lobe is involved in the recognition and integration of sensory perceptions. Disorders in which sensory processing is defective include sleep disorders, chronic pain syndromes, and disorders of the special senses such as blindness, deafness, and taste and olfactory dysfunction. Among the drugs that affect sensory processing are antidepressants, hallucinogens, local and general anesthetics, opioid analgesics, and sedative-hypnotics NEURONAL SYSTEMS IN THE CENTRAL NERVOUS SYSTEM Motor Processing Motor processing refers to the neuronal activity that enables body movement. The structures involved in motor processing include the cerebellum, the motor strip of the frontal lobe cortex, the basal ganglia, and the suprasegmental nuclei that are found in the brainstem and are involved in the control of posture (e.g., the vestibular nuclei). Disturbances in motor processing occur in Parkinson disease, Huntington disease, and a variety of degenerative and demyelinating neuron disorders. Drugs that affect motor processing include antiparkinsonian drugs, CNS stimulants, muscle relaxants, antispastic drugs, and sedative-hypnotics. NEURONAL SYSTEMS IN THE CENTRAL NERVOUS SYSTEM Autonomic Processing Autonomic processing involves areas of the brain that integrate the activities of the peripheral autonomic nervous system. These areas include the hypothalamus and portions of the brainstem, such as the vasomotor center and the cranial nuclei of parasympathetic nerves. Disorders of autonomic processing include orthostatic hypotension and postural tachycardia syndrome. Some CNS drugs alter autonomic processing by affecting the actions of hypothalamic and brainstem nuclei, and others have a direct effect on peripheral autonomic neurotransmission. The drugs that affect autonomic processing include antidepressants, antiparkinsonian drugs, antipsychotics, and drugs used to treat Alzheimer disease.