Clinical Hematology - Turgeon PDF
Document Details
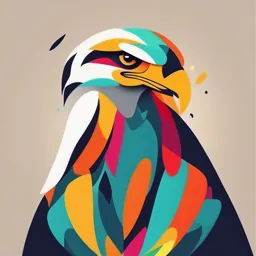
Uploaded by SubsidizedEternity
Institute of Health Technology, Dhaka
Tags
Summary
This document is a textbook on clinical hematology, specifically focusing on myeloproliferative neoplasms, case studies and diagnostic techniques. It discusses the various case studies in detail. It includes questions throughout the document.
Full Transcript
396 PART 4 Myeloproliferative Neoplasms Treatment Prognosis Treatment considerations in MDS must weigh the risk of therapy against the risk of problems associated with existing cytopenias as well as the likelihood and imminence of leukemic transformation. In patients with acute leukemia transforming...
396 PART 4 Myeloproliferative Neoplasms Treatment Prognosis Treatment considerations in MDS must weigh the risk of therapy against the risk of problems associated with existing cytopenias as well as the likelihood and imminence of leukemic transformation. In patients with acute leukemia transforming from MDS, the clinical responses to the standard therapy are poor. Although MDS is rare in children, these represent some of the most difficult dyscrasias to treat. Children treated for MDS respond poorly to conventional chemotherapy. Survival is generally good for patients with RA and RARS. The median survival time for all patients with MDS is approximately 2 years. MDS patients with multiple karyotypic anomalies have a shorter survival time (average, 8 months) than patients with single anomalies (average, 18 months) or those with a normal karyotype (average, 36 months). CASE STUDIES CASE 22.1 An elderly white woman with a history of anemia visited her primary care provider because of increasing shortness of breath, dizziness, and severe fatigue. She had no history of prior treatment with drugs or exposure to lead or other toxins. Her physician ordered a CBC. lines is characteristic as is the presence of abnormal metarubricytes and Pappenheimer bodies. Hyposegmentation of the neutrophils (Pelger-Huët anomaly) can be observed in myelodysplasia or acute leukemias. Karyotyping would further support the diagnosis. DIAGNOSIS: Refractory Anemia with Ring Sideroblasts Laboratory Data RBC 3.52 × 1012/L Hematocrit 37% Hemoglobin 12.4 g/dL WBC 8.4 × 109/L Platelets 275 × 109/L The peripheral blood smear demonstrated 3+ polychromatophilia, coarse basophilic stippling, occasional Pappenheimer bodies, and occasional hyposegmented neutrophils. Ten nucleated RBCs were seen per hundred leukocytes counted on the peripheral blood differential. Significant thrombocytopenia with occasional giant platelets were also noted. Follow-Up Laboratory Data Reticulocyte count 4.3% Bone Marrow Examination The bone marrow was hypercellular with erythroid hyperplasia. The RBC precursors exhibited an abnormal growth (dysplastic) pattern with nuclear:cytoplasmic asynchrony. Many binuclear rubricytes were observed. More than 15% of the metarubricytes were sideroblasts. Questions 1. What are sideroblasts? 2. What do sideroblasts represent? 3. What is the most probable diagnosis in this case? Discussion 1. Sideroblasts are erythrocytes with iron particles within the cytoplasm. 2. Sideroblasts represent defective heme synthesis and an overload of iron in developing erythroid precursors. 3. Sideroblasts are the hallmark of a myelodysplastic disorder. In this case, dyspoiesis of various blood cell CASE 22.2 A 26-year-old white man, with a history of colitis 20 years earlier, visited his family practitioner because of fatigue. He is taking medication for hypertension (Captopril) and carbonic anhydrase inhibitor for glaucoma. He states that he drinks 1 to 2 beers a day. He had a cold several weeks ago and has not felt well since then. He has no lifestyle risk factors. His physical examination revealed bruises in unusual locations (e.g., inside his thigh) with no report of any injury. He has scattered petechiae. Laboratory Data RBC 2.47 × 1012/L Hematocrit 24% Hemoglobin 7.7 g/dL WBC 4.4 × 109/L Platelets 10 × 109/L MCV 96 fL The peripheral blood smear exhibited macrocytic, hypochromic red blood cells. A few hyposegmented neutrophils were observed. Follow-Up Laboratory Data Reticulocyte count 0.3% Bone marrow examination revealed a hypercellular marrow with dysplasia of the hematopoietic cells. The Prussian blue stain was positive for iron. Questions 1. What is the cause of his elevated MCV? 2. What is the significance of the hypercellular bone marrow? 3. What could be the potential cause of this patient’s anemia? (continued) Turgeon_Chap22.indd 396 10/26/2010 12:19:02 PM CHAPTER 22 Myelodysplastic Syndromes and Myelodysplastic/Myeloproliferative Neoplasms 397 CASE STUDIES (continued) Discussion (Reprinted with permission from Anderson SC, Anderson’s, Atlas of Hematology, Philadelphia, PA: Wolters Kluwer Health, Lippincott Williams & Wilkins, 2003, Copyright 2003.) 1. With the presence of an increased MCV, it is unlikely that this patient has iron deficiency anemia. An elevated MCV could be caused by liver damage (e.g., alcohol abuse) or a vitamin deficiency. His history of colitis could be the cause. 2. A hypercellular bone marrow would rule out aplastic anemia as the cause of the patient’s anemia. In addition, the presence of iron in the bone marrow further confirms the absence of iron deficiency anemia. 3. The medications that are being taken by the patient are known to have the potential to cause bone marrow suppression. No other evidence supports a virally induced aplastic anemia or the presence of leukemia. DIAGNOSIS: MDS REVIEW QUESTIONS 1. Patients with some variety of MDS are at increased risk 6. The incidence of chromosomal abnormality in adults of developing A. acute lymphoblastic leukemia B. AML C. chronic lymphocytic leukemia D. chronic myelogenous leukemia 2. Which of the following agents has not been supported by scientific research as being associated with the development of secondary MDS? A. Alkylating agents B. Organic solvents C. Insecticides D. Both B and C 3. An increased incidence of MDSs is seen in A. males younger than 55 years of age B. females younger than 55 years of age C. males older than 55 years of age D. females older than 55 years of age 4. The most frequently involved chromosomes in adults with MDS are A. 1, 5, and 7 B. 3, 5, and 8 C. 5, 7, and 8 D. 8, 12, and 13 5. The most frequent chromosomal abnormalities in children with MDS include all of the following except A. trisomy 8 B. monosomy 7 C. deletion of long arm of chromosome 20 D. all of the above with MDS is A. 5% to 15% B. 15% to 25% C. 25% to 60% D. 40% to 90% 7. The karyotype associated with a high probability of transforming to AML is A. monosomy 5 B. monosomy 7 C. trisomy 11 D. both A and B 8. Patients with MDSs commonly suffer from _____ initially. A. a rash B. anemia C. visual disturbances D. vertigo Turgeon_Chap22.indd 397 Questions 9 through 11: Match the type of myelodysplastic syndrome with the appropriate description. 9. Refractory anemia (RA) 10. Refractory anemia with ring sideroblasts (RARS) 11. Refractory anemia with excess blasts, type 1 (RAEB-1) A. anemia, no blasts B. anemia, less than 1% blasts C. cytopenia(s), less than 5% blasts, no Auer rods 12. In young patients, the therapy of choice for MDSs involves A. vitamins B. allogeneic bone marrow transplantation C. cytotoxic drugs D. colony-stimulating growth factors 10/26/2010 12:19:02 PM 398 PART 4 Myeloproliferative Neoplasms BIBLIOGRAPHY Bennett JM, et al. Acute myeloid leukemia and other myelopathic disorders following treatment with alkylating agents, Hematol Pathol, 1(2):99–104, 1987. Borbenyi Z, et al. Factors influencing leukemic transformation in myelodysplastic syndrome, Orv Hetil, 131(23):1231–1236, 1239, 1240, 1990. Brent BJ, Nandedkar M. Vular and oral lesions in a 34-year old woman, Labmedicine, 36(10):644–646, 2005. Estey EH, et al. Karyotype is prognostically more important than the FAB system’s distinction between myelodysplastic syndrome and acute myelogenous leukemia, Hematol Pathol, 1(4):203–208, 1987. Heaney ML, Golde DW. Myelodysplasia, N Engl J Med, 340(21):1649– 1660, 1999. Leone G. Therapy-related leukemia and myelodysplasia: susceptibiity and incidence, Hemotologica, 92(10):1389–1398, 2007. List A, et al. Lenalidomide in the myelodysplastic syndrome with chromosome 5q deletion, N Eng J Med, 355(14):1456–1465, 2006. List A, et al. Efficacy of lenalidomide in the myelodysplastic syndromes, N Eng J Med, 352(6):549–557, 2005. Malcovatti L, et al. Prognostic factors and life expectancy in myelodysplastic syndromes classified according to WHO criteria: a basis for clinical decision making, J Clin Oncol, 23(30):7594– 7603, 2005. Turgeon_Chap22.indd 398 Mufti GJ, et al. Diagnosis and classification of myelodysplastice syndrome: international working group on morphology of myelodysplastic syndrome (IWGM-MDS) consensus proposals for the definition and enumeration of myeloblasts and ring sideroblasts, Haematologica, 93(11):1712–1717, 2008. Nimer SD. Myelodysplastic syndromes, Blood, 111(10):4841–4851, May 2008. Rappaport ES, et al. Myelodysplastic syndrome: Identification in the routine hematology laboratory, South Med J, 80(8):969–974, 1987. Seo IS, Li CY. Myelodysplastic syndrome: Diagnostic implications of cytochemical and immunocytochemical studies, Mayo Clin Proc, 68(1):47–53, 1993. Sridhar K, et al. Relationship of differential gene expression profiles in CD34+ myelodysplastic syndrome marrow cells to disease subtype and progression, Blood, 114(23):4847–4858, 2009. Suciu S, et al. Results of chromosome studies and their relation to morphology, course, and prognosis in 120 patients with de novo myelodysplastic syndrome, Cancer Genet Cytogenet, 44(1):15–26, 1990. Turgeon ML. Myelodysplastic syndromes, Guthrie J, 61(4):149–159, 1992. 10/26/2010 12:19:02 PM PART FIVE Principles and Disorders of Hemostasis and Thrombosis CHAPTER 23 Principles of Hemostasis and Thrombosis OBJECTIVES Overview of hemostasis and thrombosis Describe the components of bleeding and clotting mechanisms. Blood vasculature: structure and function Describe and compare the histological features of the tissues of the arteries and veins. Name the blood vessels that constitute the microcirculation and compare their size and other features with those of arteries and veins. Define the term vasoconstriction. Explain how vasoconstriction participates in hemostasis. Describe the metabolic activity of the endothelium and its role in hemostasis. Outline the general process of hemostasis in small vessels that contributes to the maintenance of vascular integrity. The megakaryocytic cell series Define the term endoreduplication and relate this process to megakaryocytic development. List and explain the three functions of thrombopoietin or thrombopoietin-like cytokines. Describe the morphological features of the mature stages of development in the megakaryocyte series. Describe the process of formation of platelets from a megakaryocyte. List the ultrastructural components and cytoplasmic constituents of a mature platelet and describe the overall function of each. Explain the life span activities of a mature platelet. Explain the function of platelets in response to vascular damage. Define generally the terms platelet adhesion and platelet aggregation. Explain the events that take place during platelet adhesion, including the substances produced. Explain the events that take place during platelet aggregation. List substances that promote and substances that inhibit some aspect of platelet aggregation. Briefly describe the process of platelet plug consolidation and stabilization. Blood coagulation factors Explain the procedure for naming the coagulation factors. List the principal coagulation factors. Name the three groupings of coagulation factors and describe their similarities. Describe the individual functional characteristics of each of the coagulation factors. Name the four basic phases of blood coagulation. Describe the sequence of events in the extrinsic pathway. Describe the sequence of events in the intrinsic pathway. Describe the sequence of events in the coagulation pathway. Name and explain the principles of the laboratory tests that are used in assessing blood coagulation factors. Normal protective mechanisms against thrombosis Explain the effect of normal blood flow and the removal of substances from the circulation on protecting the body from thrombosis. Describe the activities of antithrombin III (AT-III) as a normal body defense mechanism. Name the two heparin-dependent thrombin inhibitors and describe their role as part of the natural anticoagulant system. Describe the functions of protein C and protein S. Explain the activities of the cellular proteases and the role of specific body cells in the production of coagulation factors and cofactors. Name and describe the assay techniques that can be used for the detection of fibrin split products. 399 Turgeon_Chap23.indd 399 10/15/2010 10:13:07 AM 400 PART 5 Principles and Disorders of Hemostasis and Thrombosis OVERVIEW OF HEMOSTASIS AND THROMBOSIS The maintenance of circulatory hemostasis is achieved through the process of balancing bleeding (hemorrhage) and clotting (thrombosis). Hemostasis, the arresting of bleeding, depends on several components. The four major components are the vascular system, platelets (thrombocytes), blood coagulation factors, and fibrinolysis and ultimate tissue repair. Three other, less important, components are the complement and kinin systems as well as serine protease inhibitors. Functionally, several processes are involved in hemostasis following injury to a small blood vessel: 1. Blood vessel spasm 2. Formation of a platelet plug 3. Contact among damaged blood vessel, blood platelet, and coagulation proteins 4. Development of a blood clot around the injury 5. Fibrinolytic removal of excess hemostatic material to reestablish vascular integrity vasa vasorum, small networks of blood vessels that supply nutrients to the tissues of the wall. Veins are larger and have a more irregular lumen than arteries. In comparison with arteries, veins are relatively thin-walled with a weaker middle coat. Elastin fibers are usually found only in larger veins, and there are fewer nerves distributed to the veins than to the arteries. Arterioles and Venules Arteries branch extensively to form a tree of ever-smaller vessels. Arterioles are the microscopic continuation of arteries that give off branches called metarterioles, which in turn join the capillaries. The walls become thinner as the arterioles approach the capillaries, with the wall of a very small arteriole consisting only of an endothelial lining and some smooth muscle surrounded by a small amount of connective tissue. The microscopically sized veins are referred to as venules. Venules connect the capillaries to the veins. Capillaries BLOOD VASCULATURE: STRUCTURE AND FUNCTION Arteries and Veins Arteries are the distributing vessels that leave the heart, and veins are the collecting vessels that return to the heart. Arteries have the thickest walls of the vascular system. Although variations in the size (Fig. 23.1A) and type of vessel exist, the tissue (Fig. 23.1B) in a vessel wall is divided into three coats or tunics. These coats are the tunica intima, tunica media, and tunica adventitia. The tunica intima forms the smooth glistening surface of endothelium that lines the lumen (inner tubular cavity) of all blood and lymphatic vessels and the heart. The simple squamous epithelium that lines these vessels is referred to as endothelium. The tunica intima consists of a single layer of endothelial cells thickened by a subendothelial connective tissue layer containing elastic fibers. The tunica media, the thickest coat, is composed of smooth muscle and elastic fibers. The tunica adventitia consists of fibrous connective tissue that contains autonomic nerve endings and the The capillaries, arterioles, and venules constitute the major vessels of the microcirculation. As a unit, the microcirculation functions as the link between the arterial and venous circulation. Blood passes from the arterial to the venous system via the capillaries. Capillaries are the thinnest walled and most numerous of the blood vessels. Sinusoids, which are specialized types of capillaries, are found in locations such as the bone marrow, spleen, and liver. Capillaries are small structures consisting of a supportive basement membrane to which a single layer of endothelium is tightly anchored. The basement membrane, immediately adjacent to the endothelium, is composed of a diffuse network of small fibers that support the endothelium and act as a barrier against particulate material that may gain access to the extravascular space. Collagen bands also offer structural support to the microvascular unit. Unlike the vessels of the arterial and venous systems, capillaries are composed of only one cell layer of simple squamous epithelium, which permits a more rapid rate of transport of materials between blood and tissue. Electron microscope examination of the FIGURE 23.1. Blood vasculature. A: Size of vessels. B: Tissue zones. A B Turgeon_Chap23.indd 400 10/15/2010 10:13:08 AM CHAPTER 23 Principles of Hemostasis and Thrombosis endothelium demonstrates organelles such as mitochondria, ribosomes, and endoplasmic reticulum. VASCULATURE PHYSIOLOGY The Role of Vasoconstriction in Hemostasis Vascular injury to a large or medium-size artery or vein requires rapid surgical intervention to prevent exsanguination. When a smaller vessel, such as an arteriole, venule, or capillary, is injured, contraction occurs to control bleeding. This contraction of the blood vessel wall is called vasoconstriction. Vasoconstriction is a short-lived reflex reaction of the smooth muscle in the vessel wall produced by the sympathetic branches of the autonomic nervous system. This narrowing, or stenosis, of the lumen of the blood vessel decreases the flow of blood in the injured vessel and surrounding vascular bed and may be sufficient to close severed capillaries. The Role of the Endothelium The endothelium contains connective tissues such as collagen and elastin. This connective tissue matrix regulates the permeability of the inner vessel wall and provides the principal stimulus to thrombosis following injury to a blood vessel. The endothelium is highly active metabolically and is involved in the clotting process by producing or storing clotting components (discussed in detail in a later section of this chapter). It is also rich with plasminogen activator, which, if appropriately stimulated, is released and activates plasminogen, which ensures rapid lysis of fibrin clots. Additionally, the endothelium elaborates prostacyclin, which is synthesized by the endothelium from prostaglandin precursors and strongly inhibits platelet aggregation and adhesion. TABLE 23.1 401 Minimal interactions leading to platelet activation or clot formation occur between the circulating blood and intact endothelial surfaces. However, disrupted endothelial cells release thromboplastic substances that can initiate coagulation. Collagen, in particular, initiates contact activation of factor XII, thereby initiating blood coagulation. The endothelium forms a biological interface between circulating blood elements and all the various tissues of the body. It is strategically situated to monitor systemic as well as locally generated stimuli and to adaptively alter its functional state. This adaptive process typically proceeds without notice, contributing to normal homeostasis. The presence of a unique organelle discovered by Weibel and Palade in 1964 turned out to be an important marker to identify authentic endothelial cells. This organelle, called Weibel-Palade body (WPB) represents the storage granule for von Willebrand factor, a molecule mediating platelet adhesion. A receptor for leukoyctes (P selectin) was found in WPB membrane. Secretion of these organelles provides a rapid method for activated endothelium to become adhesive for platelets and leukocytes. Nonadaptive changes in endothelial structure and function, provoked by pathophysiological stimuli, can result in localized, acute, and chronic alterations in the interactions of endothelium with the cellular and macromolecular components of circulating blood and of the blood vessel wall. These alterations can include Enhanced permeability to (and subsequent oxidative modification of) plasma lipoproteins Hyperadhesiveness for blood leukocytes Functional imbalances in local prothrombotic and antithrombotic factors, growth simulators and inhibitors, and vasoactive (dilator, constrictor) substances (Tables 23.1 and 23.2) Endothelial Prothrombotic-Antithrombotic Balancea Prothrombotic Antithrombotic Platelet-activating factor Prostacyclin Tissue factor Thrombomodulin von Willebrand factor Tissue plasminogen activator Plasminogen activator Urokinase Inhibitor-1 Heparin-like molecules Other coagulation factors Synthesis of factor V Binding of factors V, IXa, Xa Activation factor XII These various endothelial-associated factors and functions contribute to a dynamic physiological antagonism or “balance” that determines the status of local hemostatic/thrombotic activity. a Turgeon_Chap23.indd 401 10/15/2010 10:13:09 AM 402 TABLE PART 5 Principles and Disorders of Hemostasis and Thrombosis 23.2 Endothelial Vasoconstrictor-Vasodilator Balance Constrictor Dilator Endothelin-I Prostacyclin Angiotensin-II Nitric oxide Vasoconstrictor Other “EDRF-like” substances Prostaglandins These various endothelial-generated substances contribute to the local regulation of vascular tone through their effects on smooth muscle contractility. The endothelium is involved in the metabolism and clearance of molecules such as serotonin, angiotensin, and bradykinin that affect blood pressure regulation, the movement of fluid across the endothelium, and inflammation. With respect to blood coagulation, one of the basic characteristics of normal, intact endothelium is its nonreactivity with platelets and inability to initiate surface contact activation of clotting factor XII (Table 23.3). The Endothelins In 1985, a family of peptides, named the endothelins, was isolated and identified. The three members of the family—endothelin-1, endothelin-2, and endothelin-3—are produced in a variety of tissues, where they act as modulators of vasomotor tone, cell proliferation, and hormone production. Endothelin-1 is the only family member produced in endothelial cells and is also produced in vascular smooth muscle cells. It is not stored in secretory granules within endothelial cells. Stimuli such as hypoxia, ischemia, or shear stress induce the mRNA and synthesis and secretion of endothelin-1 within minutes. The half-life is approximately 4 to 7 minutes. Endothelin-2 is produced predominantly within the kidney and intestine, with smaller amounts produced in the myocardium, placenta, and uterus. The cells of origin are not clear. Endothelin-2 has no unique physiological functions as compared with endothelin-1. Endothelin-3, like endothelin-1, circulates in the plasma, but its source is not known. Endothelin-3 has been found in high concentrations in the brain and may regulate important functions, such as proliferation and development in neurons and astrocytes. It also is found throughout the gastrointestinal tract and in the lung and kidney. All three endothelins bind to two types of receptors (A and B) on the cells of many mammalian species, including humans. Endothelin-A receptors are expressed abundantly on vascular smooth muscle cells and cardiac myocytes. These receptors mediate the vasoconstrictor action of endothelin-1, although endothelin-B receptors may contribute Turgeon_Chap23.indd 402 TABLE 23.3 Endothelial Functions Angiogenesis Synthesis of stromal components Coagulation Vascular tone regulation Inflammation Special metabolic functionsa Immune responses Transporting molecules from the vascular lumen to the subendothelium, producing angiotensin-converting enzyme, and binding lipoproteins, high-density lipoproteins, and lowdensity lipoproteins. a to this action in some vascular beds. Type B receptors are expressed predominately on endothelial cells and to a much lesser extent on vascular smooth muscle cells. Endothelin-B receptors bind endothelin-1 and endothelin-3 with similar affinity. Endothelial Dysfunction Manifestations, collectively termed endothelial dysfunction, play an important role in the initiation, progression, and clinical complications of various forms of inflammatory and degenerative vascular diseases. Various stimuli of endothelial dysfunction have been identified, including immunoregulatory substances such as tumor necrosis factor (TNF) and interleukin-1 (IL-1), viral infection and transformation, bacterial toxins, and cholesterol and oxidatively modified lipoproteins. Disruption of the endothelium directly activates all four components of hemostasis. After this event, the following events take place: 1. Initially, rapid vasoconstriction for up to 30 minutes reduces blood flow and promotes contact activation of platelets and coagulation factors. 2. In the second phase, platelets adhere immediately to the exposed subendothelial connective tissue, particularly collagen. The aggregated platelets enhance sustained vasoconstriction by releasing thromboxane A2 and vasoactive amines, including serotonin and epinephrine. 3. In the third phase, coagulation is initiated through both the intrinsic and extrinsic systems. 4. Finally, fibrinolysis occurs following the release of tissue plasminogen activators (t-PAs) from the vascular wall. Fibrinolytic removal of excess hemostatic material is necessary to reestablish vascular integrity. Maintenance of Vascular Integrity Vascular integrity or the resistance to vessel disruption requires three essential factors. These factors are circulating functional platelets, adrenocorticosteroids, and ascorbic acid. A lack of 10/15/2010 10:13:09 AM CHAPTER 23 Principles of Hemostasis and Thrombosis these factors produces fragility of the vessels, which makes them prone to disruption. Maintenance of vascular integrity through the hemostatic process depends on the events previously described. The importance of these reactions varies with vessel size (e.g., capillaries seal easily because of vasoconstriction). The integrity of arterioles and venules depends on vasoconstriction, the formation of a plug of fused platelets over the injury, and the formation of a fibrin clot. Arteries, because of their thick walls, are the most resistant to bleeding; however, hemorrhage from these vessels is the most dangerous. Vasoconstriction is of ultimate importance in damaged arteries. Veins, which contain 70% of the blood volume, may rupture with a slight increase in hydrostatic pressure. 403 Megakaryoblast Promegakaryocyte THE MEGAKARYOCYTIC CELL SERIES Mature platelets (thrombocytes) (Fig. 23.2), metabolically active cell fragments, are the second critical component in the maintenance of hemostasis. These anuclear cells circulate in the peripheral blood after being produced from the cytoplasm of bone marrow megakaryocytes, the largest cells found in the bone marrow. Megakaryocyte General Characteristics of Megakaryocytic Development Bone marrow megakaryocytes Figure 23.3 are derived from pluripotential stem cells. The sequence of development from megakaryocytes to platelets is thought to progress from the proliferation of progenitors to polyploidization, that is, nuclear endoreduplication, and finally to cytoplasmic maturation and the formation of platelets. There appears to be a complex relationship between the circulating platelet mass and the number, ploidy, and size of megakaryocytes, but the sensing mechanisms that regulate platelet production have not yet been identified. Recently, the gene for the human mpl protein was cloned and found to be expressed selectively in megakaryocytic cells. It was then found that antisense oligonucleotides that block the synthesis of human mpl protein inhibit the formation of megakaryocyte colonies but not of erythroid or granulocyte-macrophage colonies in vitro. This orphan receptor of unknown function might be the receptor for thrombopoietin. The protein may act synergistically with other growth factors during the proliferation state. It is not known whether further hormonal stimulation is needed for cytoplasmic maturation or platelet release. Megakaryocytopoiesis proceeds initially through a phase characterized by mitotic division of a progenitor cell, followed by a wave of nuclear endoreduplication. Endoreduplication is the process in which chromosomal material (DNA) and the other events of mitosis occur without subsequent division of the cytoplasmic membrane into identical daughter cells. Recognizable megakaryocytes have ploidy values of 4n, 8n, 16n, and 32n. The maturation of megakaryocytes from immature, largely non–DNA-synthesizing cells to morphologically iden- Turgeon_Chap23.indd 403 Platelets FIGURE 23.2. Normal megakaryocytic series. (Reprinted with permission from Anderson SC. Anderson’s Atlas of Hematology, Philadelphia, PA: Wolters Kluwer Health/Lippincott Williams & Wilkins, Copyright 2003.) tifiable megakaryocytes involves processes such as the appearance of cytoplasmic organelles, the acquisition of membrane antigens and glycoproteins, and the release of platelets. Thrombopoietin, the hormone thought to stimulate the production and maturation of megakaryocytes, which in turn produce platelets, has recently been purified and cloned. Thrombopoietin activity results from several different cytokines: erythropoietin, IL-3, and granulocyte-macrophage colony-stimulating factor (GM-CSF). These substances have been shown to be able to increase megakaryocyte size, maturational stage, and ploidy. The Developmental Sequence of Platelets Early Development Two classes of progenitors have been identified: the burst-forming-unit megakaryocyte (BFU-M) and the colony-forming-unit megakaryocyte (CFU-M). The BFU-M 10/15/2010 10:13:10 AM 404 PART 5 Principles and Disorders of Hemostasis and Thrombosis FIGURE 23.3. Granular megakaryocyte from an MGG-stained normal bone marrow smear. (Reprinted with permission from Mills SE. Histology for Pathologists, 3rd ed, Philadelphia, PA: Lippincott Williams & Wilkins, 2007.) is the most primitive progenitor cell committed to megakaryocyte lineage. The next stage of megakaryocyte development is a small, mononuclear marrow cell (Fig. 23.4) that expresses platelet-specific phenotypic markers but is not morphologically identifiable as a megakaryocyte. These transitional cells represent 5% of marrow megakaryocyte elements. Some transitional immature megakaryocyte cells may be capable of cellular division, but most are nonproliferating while actively undergoing endomitosis. Megakaryocytes The final stage of megakaryocyte development is the morphologically identifiable megakaryocyte (Fig. 23.5). These cells are readily recognizable in the marrow because of their large size and lobulated nuclei. These cells are polyploid (Table 23.4). Megakaryocytes are the largest bone marrow cells, ranging up to 160 mm in size. The nuclear-cytoplasmic (N:C) ratio can be as high as 1:12. Nucleoli are no longer visible. A FIGURE 23.5. Megakaryocyte. distinctive feature of the megakaryocyte is that it is multilobular, not multinucleated. The fully mature lobes of the megakaryocyte shed platelets from the cytoplasm on completion of maturation. Platelet formation begins with the initial appearance of a pink color in the basophilic cytoplasm of the megakaryocyte and increased granularity. Mature Platelets Platelets have an average diameter of 2 to 4 mm, with younger platelets being larger than older ones. In contrast to megakaryocytes, platelets have no nucleus. The cytoplasm is light blue, with evenly dispersed, fine red-purple granules. TABLE 23.4 Developmental Characteristics of Mature Megakaryocytic Cells Size Megakaryocyte Platelet 30–160 µm 2–4 µm Nuclear-cytoplasmic 1:1–1:12 ratio Nucleus Shape Lobulated (two or more lobes) (Anuclear) Chromatin color Blue-purple — Chromatin clumping Granular — Nucleoli Not visible — Color Pinkish blue Light-blue fragments Shape Occasional pseudopods Cytoplasm Irregular border FIGURE 23.4. Megakaryoblast. (Reprinted with permission from Anderson SC. Anderson’s Atlas of Hematology, Philadelphia, PA: Wolters Kluwer Health/Lippincott Williams & Wilkins, Copyright 2003.) Turgeon_Chap23.indd 404 Amount Abundant Granules Abundant near the Scattered borders of the cytoplasm 10/15/2010 10:13:16 AM CHAPTER 23 Principles of Hemostasis and Thrombosis An inactive or unstimulated platelet circulates as a thin, smooth-surfaced disc. This discoid shape is maintained by the microtubular cytoskeleton beneath the cytoplasmic membrane. Platelets circulate at the center of the flowing bloodstream through endothelium-lined blood vessels without interacting with other platelets or with the vessel wall. Platelets are extremely sensitive cells and may respond to minimal stimulation by forming pseudopods that spontaneously retract. Stronger stimulation causes platelets to become sticky without losing their discoid shape; however, changes in shape to an irregular sphere with spiny pseudopods will occur with additional stimulation. This alteration in cellular shape is triggered by an increase in the level of cytoplasmic calcium. Such changes in shape accompanied by internal cellular contractions can result in the release of many of the internal organelles. A loss of viability is associated with this change to a spiny sphere. Cellular Ultrastructure of a Mature Platelet Examination of a platelet with an electron microscope reveals a variety of structures. These structures are fundamental to the functioning of the platelet. The Glycocalyx Ultrastructure examination of the platelet (Fig. 23.6) reveals that the cellular membrane is surrounded externally by a fluffy coat or glycocalyx. This glycocalyx is unique among the cellular components of the blood. It is composed of 405 plasma proteins and carbohydrate molecules that are related to the coagulation, complement, and fibrinolytic systems. The glycoprotein receptors of the glycocalyx mediate the membrane contact reactions of platelet adherence, change of cellular shape, internal contraction, and aggregation. Cytoplasmic Membrane Adjacent to the glycocalyx is the cytoplasmic membrane whose chemical composition and physical structure are described in Chapter 3. Extending through the plasma membrane and into the interior of the platelet is an open canalicular or surface-connecting system. It is this system that forms the invaginated, sponge-like portion of the cell that provides an expanded reactive surface to which plasma clotting factors are selectively adsorbed. Contact activation of the membrane phospholipids also generates procoagulant activity and arachidonic acid to the blood-clotting process. The cytoplasmic membrane and open canalicular membrane system articulate with the dense tubular system that is not surface connected. Although the canaliculi penetrate the cytoplasm in a random manner, they are generally in close proximity to granules and other organelles. Therefore, products released by the granules or cytoplasm can be transported to the exterior environment through the canaliculi. In addition to the movement of extracellular materials against the concentration gradient through the canaliculi, phagocytosis is also likely to occur through these channels. Additionally, the channels of the open canalicular system and dense tubular system appear to constitute the calcium-regulating mechanism of the cell. Microfilaments and Microtubules Directly beneath the cell membrane is a series of submembrane filaments and microtubules that form the cellular cytoskeleton. In addition to providing the structure for maintaining the circulating discoid shape of the cell, the cytoskeleton also maintains the position of the organelles. A secondary system of microfilaments is functional in internal organization and secretion of blood coagulation products, such as fibrinogen. The microfilaments interact with the dense tubular system in sequestering calcium, which initially causes centralization of internal organelles. These subcellular and cytoplasmic filaments make up the contractile system (sol gel zone) of the platelet. FIGURE 23.6. Platelet with its inventory of granular content. ADP, adenosine diphosphate; ATP, adenosine triphosphate; FV, factor V; GPIb, platelet surface glycoprotein Ib; PDGF, platelet-derived growth factor; PF4, platelet factor 4; TGF-b, transforming growth factor-b; vWf, von Willebrand factor. (Reprinted with permission from Koopman WJ, Moreland LW. Arthritis and Allied Conditions: A Textbook of Rheumatology, 15th ed, Philadelphia, PA: Lippincott Williams & Wilkins, 2005.) Turgeon_Chap23.indd 405 Granules Three different types of storage granules related to hemostasis are present in the mature platelet. These granules are alpha granules, dense or delta granules, and lysosomes. The alpha granules are the most abundant. Alpha granules contain heparin-neutralizing platelet factor 4 (PF 4), beta-thromboglobulin, platelet-derived growth factor, platelet fibrinogen, fibronectin, von Willebrand factor (vWF), and thrombospondin. Dense bodies, named because of their appearance when viewed by electron microscopy, contain serotonin, adenosine diphosphate (ADP), adenosine triphosphate (ATP), and calcium. Lysosomes, the third type of granule, store hydrolase 10/15/2010 10:13:28 AM 406 PART 5 Principles and Disorders of Hemostasis and Thrombosis enzymes. Extrusion of the contents of these storage granules requires internal, cellular contraction. Secretions from the granules are released into the open canalicular system. Other Cytoplasmic Constituents In addition to containing substantial quantities of the contractile proteins, including actomyosin (thrombosthenin), myosin, and filamin, the cytoplasm of the platelet contains glycogen and enzymes of the glycolytic and hexose pathways. Energy for metabolic activities and cellular contraction is derived from aerobic metabolism in the mitochondria and anaerobic glycolysis–utilizing glycogen stores. The platelet is a very high-energy cell with a metabolic rate 10 times that of an erythrocyte. Based on energy availability and endogenous constituents, the platelet is effectively equipped to fulfill the role of protecting the body against vascular trauma. Platelet Kinetics, Life Span, and Normal Values An average megakaryocyte produces about 1,000 to 2,000 platelets. Marrow transit time, or the maturation period of the megakaryocyte, is approximately 5 days. It is believed that platelets initially enter the spleen, where they remain for 2 days. Following this period, platelets are in either the circulating blood or the active splenic pool. At all times, approximately two thirds of the total number of platelets are in the systemic circulation, while the remaining one third exist as a pool of platelets in the spleen that freely exchange with the general circulation. A normal person has an average of 250 × 109/L (range, 150 × 109/L to 450 × 109/L) platelets in the systemic circulation. Platelet turnover or effective thrombopoiesis averages 350 × 109/L ± 4.3 × 109/L/day. The life span of a mature platelet is 9.0 days ± 1 day. At the end of their life span, platelets are phagocytized by the liver and spleen and other tissues of the mononuclear phagocytic system. PLATELET FUNCTION IN HEMOSTASIS Platelets normally move freely through the lumen of blood vessels as components of the circulatory system. Maintenance of normal vascular integrity involves nourishment of the endothelium by some platelet constituents or the actual incorporation of platelets into the vessel wall. This process requires less than 10% of the platelets normally in the circulating blood. For hemostasis to occur, platelets not only must be present in normal quantities but also must function properly. This section discusses the hemostatic functions of platelets, including platelet adherence and aggregation. Overall Functions of Platelets Following damage to the endothelium of a blood vessel, a series of events occur, including adhesion to the injured vessel, shape change, aggregation, and secretion. Each structural and functional change is accompanied by a series Turgeon_Chap23.indd 406 of biochemical reactions that occur during the process of platelet activation. The platelet plasma membrane is the focus of interactions between extracellular and intracellular environments. Agonists that lead to platelet activation are varied and include a nucleotide (ADP), lipids (thromboxane A2, platelet-activating factor), a structural protein (collagen), and a proteolytic enzyme (thrombin). One of the distinct activities associated with platelet activity in response to vascular damage is the continued maintenance of vascular integrity by the rapid adherence of platelets to exposed endothelium. In addition, platelets spread, become activated, and form large aggregates. Formation of a platelet plug initially arrests bleeding. The adherence and aggregation of platelets at the sites of vascular damage allow for the release of molecules involved in hemostasis and wound healing and provide a membrane surface for the assembly of coagulation enzymes that lead to fibrin formation. Vascular healing is promoted by stimulating the migration and proliferation of endothelial cells and medial smooth muscle cells through the release of the mitogen, platelet-derived growth factor. Platelet Adhesion If vascular injury exposes the endothelial surface and underlying collagen (Fig. 23.7), platelets adhere to the subendothelial collagen fibers, spread pseudopods along the surface, and clump together (aggregate). Platelet adhesion to subendothelial connective tissues, especially collagen, occurs within 1 to 2 minutes after a break in the endothelium. Epinephrine and serotonin promote vasoconstriction. ADP increases the adhesiveness of platelets. Considerable evidence indicates that the adhesion and aggregation of platelets are mediated by the binding of large soluble macromolecules to distinct glycoprotein receptors anchored in the platelet membrane. This increase in adhesiveness causes circulating platelets to adhere to those already attached to the collagen. The result is a cohesive platelet mass that rapidly increases in size to form a platelet plug. The transformation of the platelet from a disc to a sphere with pseudopods produces surface membrane reorganization. Internal contraction of the platelet results in release of granular contents of the alpha and dense granules and the lysosomal contents. This process resembles the secretory activities of other cells. Platelets adhere at sites of mechanical vascular injury and then undergo activation and express functional glycoprotein IIb/IIIa receptors (also referred to as integrin alphaIIbbeta3) for circulating adhesive ligand proteins (primarily fibrinogen). These functional glycoprotein IIb/IIIa receptors mediate the recruitment of local platelets by forming fibrinogen bridges between platelets—a process called platelet cohesion. Although functional glycoprotein IIb/IIIa receptors bind with other circulating adhesive molecules in plasma (including vWF, fibronectin, vitronectin, and thrombospondin), fibrinogen is the predominant ligand because of its rela- 10/15/2010 10:13:30 AM CHAPTER 23 Principles of Hemostasis and Thrombosis 407 APTT Intrinsic XII Kininogen-Prekallikrein-XI IX-VIII:C PT Extrinsic VII Platelet surface phospholipids Ca2+ X V Prothrombin (II) Thrombin Disrupted tissue cell membranes Ca2+ Fibrin monomer Fibrinogen TT Fibrin polymers (H-bonds) XIII Fibrin polymers (covalent bonds) FIGURE 23.7. Coagulation mechanisms. Shaded areas (“platelet surface phospholipids”) enclose the intrinsic coagulation reactions that occur on the surface membranes of platelets. Dashed lines enclose the extrinsic coagulation reactions that occur on disrupted tissue cell phospholipoprotein membranes intruded into the circulation. aPTT, activated partial thromboplastin time; PT, prothrombin time; TT, thrombin time. tively high concentration. The peptide recognition sequence arginine-glycine-aspartic acid present in these different adhesive molecules mediates binding with expressed glycoprotein IIb/IIIa receptors. Glycoprotein IIb/IIIa is specific for platelets. Platelet recruitment depends almost exclusively on the final phase of glycoprotein IIb/IIIa–dependent platelet cohesion. Glycoprotein IIb/IIIa is the most abundant platelet membrane protein (with approximately 50,000 receptors per platelet). Platelet Aggregation Platelet aggregation is the gold standard test to determine platelet function. Platelet aggregation in vivo is a much more complex and dynamic process than previously thought. Over the last decade, it has become clear that platelet aggregation represents a multistep adhesion process involving distinct receptors and adhesive ligands, with the contribution of individual receptor-ligand interactions to the aggregation process dependent on the prevailing blood flow conditions. It is now believed that three distinct mechanisms can initiate platelet aggregation. A variety of agents are capable of producing in vitro platelet aggregation, an energy-dependent process. These agents include particulate material such as collagen, proteolytic enzymes such as thrombin, and biological amines such as epinephrine and serotonin. It is believed that bridges formed by fibrinogen in the presence of calcium produce a sticky surface on platelets. This results in aggregation. If these aggregates are reinforced by fibrin, they are referred to as a thrombus. Turgeon_Chap23.indd 407 Aggregation of platelets by at least one pathway can be blocked by substances such as prostaglandin E (PGE), adenosine, and nonsteroidal anti-inflammatory agents (e.g., aspirin). Aspirin, including aspirin-containing products such as AlkaSeltzer, induces a long-lasting functional defect in platelets (Fig. 23.8). It is clinically detectable as a prolongation of bleeding time. The mechanism of aspirin appears to be primarily, if not exclusively, the permanent inactivation of prostaglandin G/H synthase, which catalyzes the first step in the synthesis of the prostaglandins, the conversion of arachidonate to prostaglandin H2. Reduced formation of various eicosanoids (thromboxane A2, prostaglandin E2, and prostacyclin) in various tissues probably accounts for the variety of pharmacological effects of aspirin that form the basis of its therapeutic use and its toxicity. Because platelets lack the biosynthetic mechanisms needed to synthesize new protein, the defect induced by aspirin cannot be repaired during their life span (approximately 8 to 10 days). Therefore, after treatment with aspirin is stopped, cyclooxygenase activity recovers slowly, as a function of platelet turnover. This explains the apparent paradox of how a drug with a 20-minute half-life in the systemic circulation can be fully effective as an antiplatelet agent when administered once daily. Because of the permanent nature of aspirin-induced inactivation of platelet prostaglandin G/H synthase, the inhibitory effect of repeated daily doses less than 100 mg is cumulative. Daily administration of 30 to 50 mg of aspirin results in virtually complete suppression of platelet thromboxane biosynthesis after 7 to 10 days. These changes in platelet biochemistry are associated with maximal inhibition 10/15/2010 10:13:31 AM 408 PART 5 Principles and Disorders of Hemostasis and Thrombosis FIGURE 23.8. Mechanism of the antiplatelet action of aspirin. Aspirin acetylates the hydroxyl group of a serine residue at position 529 (Ser529) in the polypeptide chain of human platelet prostaglandin G/H synthase, resulting in the inactivation of cyclooxygenase catalytic activity. Aspirin-induced blockade of prostaglandin synthesis results in decreased biosynthesis of prostaglandin H2 and thromboxane A2. (Reprinted with permission from Patrono C. Aspirin as an antiplatelet drug, N Engl J Med, 330(18):1288, 1994. Copyright© 1994 Massachusetts Medical Society. All rights reserved.) of thromboxane-dependent platelet aggregation, and prolongation of the bleeding time accounts for the antithrombotic effects of aspirin. Qualitative platelet disorders can be attributed to adhesion, aggregation, or secretion defects. Release defects are the largest group of platelet function disorders. This condition is caused by abnormalities of signal transductase from membranes, abnormal internal metabolic pathways, or abnormal release mechanisms. Platelet Plug Consolidation and Stabilization The permanently anchored platelet plug requires additional consolidation and stabilization. Fibrinogen, under the influence of small amounts of thrombin, provides the basis for BOX 23.1 Platelet Plug Consolidation and Stabilization SUMMARY Vascular injury → exposes subendothelium and vasoconstriction → platelet adhesion → platelet aggregation → platelet plug formation → consolidation of platelets → fibrin stabilization Turgeon_Chap23.indd 408 this consolidation and stabilization. This process involves the precipitation of polymerized fibrin around each platelet. The result is a fibrin clot that produces an irreversible platelet plug (Box 23.1). Laboratory Assessment of Platelets A platelet count is a fundamental component in the evaluation of a patient. Examination of the peripheral blood smear for platelet number and morphology is critical because many clinical clues may be obtained from an evaluation of platelet quantity and morphology. Quantitative Determination of Platelets The circulating platelet count can be accurately determined in a blood sample using an electronic particle counter (see Chapter 27). Examination of a stained blood film provides a rapid estimate of platelet numbers. Normally, there are 8 to 20 platelets per 100× (oil) immersion field in a properly prepared smear (where the erythrocytes barely touch or just overlap). At least 10 different fields should be carefully examined for platelet estimation. The average number (e.g., 14) can be multiplied by a factor of 20,000 to arrive at an approximation of the quantitative platelet concentration. If an average number of 14 platelets is multiplied by 20,000, the approximate platelet concentration would be 280,000 or 280 × 109/L. Although the estimation of platelets from a blood smear does not replace an actual quantitative measurement, it should be done as a cross-check of the quantitative measurement. 10/15/2010 10:13:32 AM CHAPTER 23 Principles of Hemostasis and Thrombosis BOX 23.2 Laboratory Assessment of Platelet Function Peripheral blood smear Platelet count Template bleeding time Petechiometer Platelet aggregation Adenosine diphosphate (ADP) Epinephrine Collagen Ristocetin Arachidonate Thrombin Platelet lumiaggregation (release) Platelet antibodies (IgM and IgG) Platelet membrane glycoproteins (flow cytometry) Platelet factor IV (Beta)-thromboglobulin Thromboxanes Qualitative Assessment of Platelets If a platelet count is normal but a patient has a suggestive bleeding history, an assessment of platelet function should be conducted. Methods of evaluation (Box 23.2) include bleeding time, aggregating agents, and lumiaggregation. Bleeding Time With and Without Aspirin The bleeding time test (see Chapter 26) is an in vivo measurement of platelet adhesion and aggregation on locally injured vascular subendothelium. This test provides an estimate of the integrity of the platelet plug and thereby measures the interaction between the capillaries and platelets. Platelet adhesiveness is the process of the sticking of platelets to the vessel wall, whereas platelet aggregation is the sticking or clumping of platelets to each other. The bleeding time reflects these aspects of platelet function. As the platelet count drops below 100 × 109/L, the bleeding time increases progressively from a normal of 3 to 8 minutes to more than 30 minutes. A prolonged bleeding time in a patient with a platelet count greater than 100 × 109/L indicates either impaired platelet function or a defect of subendothelial factor. Results between 8 and 11 minutes are usually not clinically significant. The antiplatelet effects of aspirin are owing to the inhibition of thromboxane A2 synthesis, a potent mediator of platelet aggregation and vasoconstriction. The onset of the effect of aspirin is rapid. Platelet inhibition is measurable within 60 minutes. The effects of aspirin last for the duration of the life span of the platelet, approximately 7 to 10 days. Turgeon_Chap23.indd 409 409 With borderline results, the aspirin tolerance test is often useful and is repeated 2 hours after aspirin challenge. Clot Retraction The contractile abilities of platelets also result in the contraction of formed clots. Clot retraction reflects the number and quality of platelets, fibrinogen concentration, fibrinolytic activity, and packed red cell volume. Because the fibrin clot enmeshes the cellular elements of the blood, primarily erythrocytes, the degree of clot retraction is limited to the extent that fibrin contracts by the volume of erythrocytes (hematocrit). Therefore, the smaller the hematocrit, the greater the degree of clot retraction. The degree of clot retraction is directly proportional to the number of platelets and inversely proportional to the hematocrit and the level of the blood coagulation factor fibrinogen. When clot dissolution (fibrinolysis) is very active, the fibrin clot may be dissolved almost as quickly as it is formed, and clot retraction is impaired. Platelet Aggregation Most platelet aggregation procedures (see Chapter 27) are based on some variation of Born method. Agents such as ADP, collagen, epinephrine, snake venom, thrombin, and ristocetin can be used to aggregate platelets. The principle of the test is that platelet-rich plasma is treated with a known aggregating agent. If aggregated, cloudiness or turbidity can be measured using a spectrophotometer. Depending on the type of aggregating agent used, a curve that can be used to assess platelet function is obtained. In vivo, platelets participate in primary hemostasis by first adhering and then aggregating at the site of an injured blood vessel. Platelet aggregation is a contributing factor to subacute stent thrombosis. Patients undergoing a stent procedure are monitored to assess the effect of using aspirin and clopidogrel, a prodrug whose active metabolite selectively inhibits ADP-dependent platelet aggregation. In vitro, platelet aggregation assays use various platelet activators to identify abnormal platelet function and to monitor antiplatelet drug therapy. ADP, collagen, epinephrine, ristocetin, and arachidonic acid are reagents commonly used to induce platelet aggregation. The platelet aggregation procedure is performed on a turbidimetric aggregometer as first described by Born. Changes in aggregation are recorded as platelet-rich plasma and aggregating reagents are stirred together in a cuvette. The aggregometer serves as a standardized spectrophotometer. As aggregation proceeds, more light passes through the sample. Epinephrine is usually used in two doses, as is ADP. A monophasic curve is elicited with ADP. A biphasic curve is usually elicited with epinephrine. Ristocetin and arachidonic acid also usually induce a monophasic curve. Lumiaggregation is an extension of aggregation. For more than 20 years, ristocetin cofactor (RCo) assay (which measures vWF) mediated agglutination of platelets in the presence of the antibiotic, ristocetin, and has been the 10/15/2010 10:13:34 AM 410 PART 5 Principles and Disorders of Hemostasis and Thrombosis most commonly used assay for the measurement of the functional activity of vWF. Recently, a collagen-binding enzymelinked immunosorbent assay (ELISA) has been introduced as an alternative procedure. Circulating plasma vWF antigen is a marker of generalized endothelial dysfunction. Platelet Adhesion Platelet adhesion in vivo occurs as platelets attach either to a damaged vessel wall or to each other. Methods of in vitro analysis rely on the adherence of platelets to glass surfaces. The amount of adherence of platelets in a blood sample to a glass surface can be measured by counting the number of platelets before and after exposure to glass beads. The reliability of this methodology has been questioned; therefore, use of the method is not universal. Antiplatelet Antibody Assays Antibodies against platelets may appear in the plasma of patients in certain clinical conditions, although it may be difficult to demonstrate these antibodies in cases of immune thrombocytopenia. Available techniques can include complement fixation methods, lysis of chromium 51–labeled platelets, assays of platelet-bound immunoglobulins, and competitive inhibition assays. BLOOD COAGULATION FACTORS Bleeding from small blood vessels may be stopped by vasoconstriction and the formation of a platelet plug, but the formation of a clot (thrombus) usually occurs as part of the normal process of hemostasis. The soluble blood coagulation factors are critical components in the formation of a thrombus. Hepatic cells are the principal site of the synthesis of coagulation factors. However, other cells, such as endothelial cells, also play an important role in the normal process of hemostasis and thrombosis. Classically, the coagulation factors have been described as reacting in a cascading sequence. Modifications of this sequence are now known to occur as the blood factors interact to form the final insoluble gelatinous thrombus. Basic Concepts of Blood Coagulation Blood coagulation is a sequential process of chemical reactions involving plasma proteins, phospholipids, and calcium ions. Most of the circulating factors (Table 23.5) that participate in the coagulation process are designated by Roman numerals. The activated form of an enzymatic factor appears as a Roman numeral followed by the suffix -a, whereas the inactive enzymatic factors, zymogens, are indicated by the Roman numeral alone. For example, factor II, prothrombin, is designated as factor II; however, in the active state, it is IIa, thrombin. Nonenzymatic factors have no such designations. The Roman numeral designation does not indicate the sequence of reactions in the clotting process. For example, factor X precedes factor II in the coagulation pathway. Turgeon_Chap23.indd 410 Common Characteristics of Coagulation Factors Proteins that are clotting factors have four characteristics in common. These characteristics are as follows: 1. A deficiency of the factor generally produces a bleeding tendency disorder with the exception of factor XII, prekallikrein (Fletcher factor), and high–molecularweight kininogen (HMWK; Fitzgerald factor). 2. The physical and chemical characteristics of the factor are known. 3. The synthesis of the factor is independent of other proteins. 4. The factor can be assayed in the laboratory. To develop an understanding of the theory of coagulation and the underlying principles of related laboratory procedures, it is helpful to compare the characteristics (Table 23.6) of various coagulation factors. Three groups of factors exist: the fibrinogen group, the prothrombin group, and the contact group. The fibrinogen group consists of factors I, V, VIII, and XIII. These factors are consumed during the process of coagulation. Factors V and VIII are known to decrease during blood storage in vitro. These factors are known to increase during pregnancy, in the presence of conditions of inflammation, and subsequent to the use of oral contraceptive drugs. The prothrombin group consists of factors II, VII, IX, and X. All these factors are dependent on vitamin K during their synthesis. Vitamin K is available to the body through dietary sources and intestinal bacterial production. This group is inhibited by warfarin. This group is considered to be stable and remains well preserved in stored plasma. The contact group consists of factors XI, XII, prekallikrein (Fletcher factor), and HMWK (Fitzgerald factor). These factors are involved in the intrinsic coagulation pathway. They are moderately stable and are not consumed during coagulation. Characteristics of Individual Factors Each of the individual coagulation factors has some unique characteristics. These characteristics include the following: Factor I (Fibrinogen) Fibrinogen is a large, stable globulin protein (molecular weight, 341,000). It is the precursor of fibrin, which forms the resulting clot. When fibrinogen is exposed to thrombin, two peptides split from the fibrinogen molecule, leaving a fibrin monomer. These monomers aggregate together to form the final polymerized fibrin clot product. Fibrinogenthrombin → fibrin monomers → fibrin clot. Factor II (Prothrombin) Prothrombin is a stable protein (molecular weight, 63,000). In the presence of ionized calcium, prothrombin is converted to thrombin by the enzymatic action of thromboplastin from both extrinsic and intrinsic sources. Prothrombin has a half-life of almost 3 days with 70% consumption during clotting. 10/15/2010 10:13:34 AM CHAPTER 23 Principles of Hemostasis and Thrombosis TABLE 23.5 411 Proteins in Blood Coagulation Factor Name Alternate Terms Coagulation Factors I Fibrinogen II Prothrombin V Proaccelerin Labile factor, Ac globulin VII Proconvertin Stabile factor, SPCA VIII AHF AHG, antihemophilic factor A IX PTC Christmas factor, antihemophilic factor B X Stuart factor Stuart-Prower factor XI Plasma thromboplastin antecedent PTA, antihemophilic factor C XII Hageman factor Glass or contact factor XIII Fibrin-stabilizing factor FSF Others Prekallikrein Fletcher factor HMW kininogen HMW kininogen, Fitzgerald factor vWF Factor VIII–related antigen Fibronectin Antithrombin III Heparin cofactor II Protein C Protein S SPCA, serum prothrombin conversion accelerator; AHF, antihemophilic factor; AHG, antihemophilic globulin; PTC, plasma thromboplastin component; PTA, plasma thromboplastin antecedent, FSF, fibrin-stabilizing factor; HMW, high–molecular-weight kininogen; vWF, von Willebrand factor. 2+ Prothrombin + Ca extrinsic or intrinsic thromboplastin → thrombin Factor IIa (Thrombin) Thrombin (molecular weight, 40,000) is the activated form of prothrombin, which is normally found as an inert precursor in the circulation. This proteolytic enzyme, which interacts with fibrinogen, is also a potent platelet-aggregating substance. A large quantity of thrombin is consumed during the process of converting fibrinogen to fibrin. A unit of thrombin will coagulate 1 mL of a standard fibrinogen solution in 15 seconds at 28°C. Fibrinogenthrombin → fibrin monomer + peptides Tissue Thromboplastin (Formerly Factor III) Tissue thromboplastin is the term given to any nonplasma substance containing lipoprotein complex from tissues. These tissues can be from the brain, lung, vascular endothelium, liver, placenta, or kidneys; these tissue types are capable of converting prothrombin to thrombin. Turgeon_Chap23.indd 411 Ionized Calcium (Formerly Factor IV) The term ionized calcium has replaced the term factor IV. Ionized calcium is necessary for the activation of thromboplastin and for the conversion of prothrombin to thrombin. Ionized calcium is the physiologically active form of calcium in the human body, and only small amounts are needed for blood coagulation. A calcium deficiency would not be expressed as a coagulation dysfunction, except in cases of massive transfusion. Factor V (Proaccelerin) Factor V is an extremely labile globulin protein. It deteriorates rapidly, having a half-life of 16 hours. Factor V is consumed in the clotting process and is essential to the later stages of thromboplastin formation. Factor VII (Proconvertin) Factor VII, a beta-globulin, is not an essential component of the intrinsic thromboplastin-generating mechanism. It is not destroyed or consumed in clotting and is found in both plasma and serum, even in serum left at room temperature for up to 3 days. The action of factor VII is the activation 10/15/2010 10:13:36 AM 412 PART 5 Principles and Disorders of Hemostasis and Thrombosis TABLE 23.6 Characteristics of Coagulation Factors Group Characteristic Ia IIb IIIc Molecular weight High Low ? Plasma Present Present Present Serum Absent Present, except II Present Absorption (BaSO4) No Yes None or partial Destruction Thrombin, plasmin Stability Factors V, VIII unstable Heat stable Stable Increase Inflammation, pregnancy, stress and fear, oral contraceptives Pregnancy, oral contraceptives Decrease Oral anticoagulants Group I: fibrinogen group (factors I, V, VIII, XIII) b Group II: prothrombin group (factors II, VII, IX, X) c Group III: contact group (factor XI, XII, Fletcher factor, Fitzgerald factor) a of tissue thromboplastin and the acceleration of the production of thrombin from prothrombin. This factor is reduced by vitamin K antagonists. Factor VIII (Antihemophilic Factor) This factor, an acute-phase reactant, is consumed during the clotting process and is not found in serum. Factor VIII is extremely labile, with a 50% loss within 12 hours at 4°C in vitro and a similar 50% loss in vivo within 8 to 12 hours after transfusion. In addition, factor VIII can be falsely decreased in the presence of lupus anticoagulant (LA). Factor VIII can be subdivided into various functional components. The total molecule, consisting of both a high–molecular-weight fraction and a low–molecular-weight fraction, is described by the nomenclature VIII/vWF. Factor VIII/vWF consists of two major moieties. The high–molecular-weight moiety consists of the vWF, VIIIR:RCo, and VIIIR:Ag components. The low–molecular-weight moiety consists of the VIII:C and VIIIC:Ag components. Factor VIII:C has procoagulant activity as measured by clotting assay techniques. Factor VIII/vWF multimers form ionic bonds with factor VIII:C and transport VIII:C in the circulation. Factor VIIIC:Ag is a procoagulant antigen as measured by immunological techniques using antibodies for factor VIII:C. Factor VIIIR:Ag is a related factor VIII antigen that has been identified using immunological techniques employing heterologous antibodies to VIII/vWF. Factor VIIIR:RCo demonstrates ristocetin cofactor activity, which is required for the aggregation of human platelets induced by the antibiotic ristocetin. Turgeon_Chap23.indd 412 Factor VIII/vWF is factor VIII-vWF. Endothelial cells are known to synthesize and secrete VIII/vWF multimers. Factor IX (Plasma Thromboplastin Component) Factor IX is a stable protein factor that is neither consumed during clotting nor destroyed by aging at 4°C for 2 weeks. It is an essential component of the intrinsic thromboplastingenerating system, where it influences the amount rather than the rate of thromboplastin formation. Factor X (Stuart Factor) This alpha-globulin is a relatively stable factor that is not consumed during clotting. Together with factor V, factor X in the presence of calcium ions forms the final common pathway through which the products of both the extrinsic and intrinsic thromboplastin-generating systems merge to form the ultimate thromboplastin that converts prothrombin to thrombin. The activity of factor X appears to be related to factor VII. Factor XI (Plasma Thromboplastin Antecedent) Factor XI, a beta-globulin, can be found in serum because it is only partially consumed during the clotting process. This factor is essential to the intrinsic thromboplastin-generating mechanism. Factor XII (Hageman Factor) Factor XII is a stable factor that is not consumed during the coagulation process. Adsorption of factor XII and kininogen (with bound prekallikrein and factor XI) to negatively charged surfaces such as glass or subendothelium (collagen) exposed by blood vessel injury initiates the intrinsic 10/15/2010 10:13:38 AM