Refrigeration and Air Conditioning PDF
Document Details
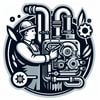
Uploaded by SmootherEpitaph1188
CFP des Moulins
Tags
Summary
This document provides an overview of refrigeration and air conditioning principles and methods. It discusses different types of refrigeration systems, including those using ice, and the role of refrigeration in various industries.
Full Transcript
# FOURTH PART ## AUXILIARY SERVICES ### REFRIGERATION AND AIR CONDITIONING #### PRINCIPLES AND METHODS ##### First Part The refrigeration and cold storage play an essential role in modern food storage methods so much so that refrigeration and food are considered inseparable. We should therefore r...
# FOURTH PART ## AUXILIARY SERVICES ### REFRIGERATION AND AIR CONDITIONING #### PRINCIPLES AND METHODS ##### First Part The refrigeration and cold storage play an essential role in modern food storage methods so much so that refrigeration and food are considered inseparable. We should therefore review the many applications of refrigeration other than food processing. * Mechanical refrigeration and freezing processes are used in mines to freeze quicksand and mud in order to continue excavation. They're also used in artificial ice rinks and for the preservation of furs in storage. * In many areas of research and experimental work, refrigeration is used to recreate cold atmospheric conditions for conducting tests under the conditions of use. * Mortuary refrigeration is adopted in hospitals to preserve bodies for identification or investigation purposes. * The fermentation of vinegar, the bottling of aerated water, the bakery industry and the treatment of wines are all assisted by refrigeration processes using a liquid refrigerant. * Controlled refrigeration allows maintaining a constant low temperature in steel annealing baths, in solutions used for mercerizing cotton and in the water jackets of rubber processing machinery. * Refrigeration helps extract wax from lubricating oils. Air cooling is extensively used in modern large hotels, cinemas, hospitals and shops where large amounts of clean and fresh conditioned air are needed for the comfort of people. Controlled fresh air is also needed in certain specialized chemical, engineering and manufacturing processes. Refrigeration also plays a role in brewing, chocolate manufacturing and storage, explosives manufacturing and margarine production. You can therefore notice that the advantages derived from refrigeration are so exceptional that the process has become an absolute necessity in modern civilization. Competition has prompted manufacturers to seek ways to improve equipment and has forced users to make every effort to achieve higher efficiency. #### PRINCIPLES OF REFRIGERATION In heat engines of the vapor, diesel and other types, the heat generated by fuel combustion is converted into useful work. In other words, heat units are converted into work units. In refrigeration processes, this conversion is reversed; mechanical energy is converted into heat to produce cold; a statement that may seem paradoxical at first. However, if the proper interpretations of heat, temperature and cold are understood there is no confusion. ##### HEAT At one time, heat was considered a thermal substance that permeated the spaces of all matter. This widespread belief persisted until the 19th century. A body was hot or cold depending on whether it contained an excess or a deficiency of calories. We now know that heat is neither a substance nor the property of such a substance, but a form of molecular energy, transferable. This energy is converted or transferred, but never destroyed—which constitutes a fundamental law of thermodynamics. Heat simply represents a measure of the kinetic energy possessed by the moving molecules that constitute a body or substance. The greater the heat present and the greater the degree of kinetic energy or molecular motion, and vice versa. If all the heat is removed from a body, the molecules are at rest and the energy is completely absent. Heat is divided into two types: sensible and latent. When a body is subject to an increase in sensible heat, then its new thermal condition is apparent to the senses, provided that the increase is sufficient to be detected. Sensible heat is measured by temperature. Latent heat, on the other hand, is needed to change the physical state of a substance without changing its temperature. It is called latent heat because its increase or decrease is not detected by the senses; it is hidden for all practical purposes. The latent heat (2257 kJ/kg) is needed to change water at 100 °C to steam at 100 °C. Similarly, an identical amount of latent heat is emitted when steam at 100°C condenses to water at 100 °C. The latent heat (335 kJ/kg) is also required to change ice at 0 °C to water at 0 °C, and the same amount of latent heat is emitted when the water is turned back into ice. The reason why heat or energy is needed to change the state of a substance from solid to liquid or from liquid to vapor is not difficult to understand. In each case, the heat increases the energy of the molecules and overcomes the intermolecular cohesive force that tends to prevent a change of state. ##### TEMPERATURE The temperature of a body or substance is fundamentally a measure of its heat level or its ability to affect the senses. It changes as the rate of molecular movement varies. It is an indication of the intensity of heat rather than the quantity of heat. It also determines the direction of the heat flow. If a body has a higher temperature or intensity of heat than another, heat flows from the hotter body to the colder body until they have the same temperature, even if the quantity of heat in the colder body is greater than the quantity of heat in the hotter body. The methods by which heat flows from one body to another are complex phenomena. Since you're already familiar with the terms radiation, convection and conduction, let's have a quick look at these concepts. * **Radiation** involves the transfer of heat from one body to another through air, without affecting the transfer medium. * **Conduction** occurs when heat flows from one body to another by means of a material medium or by physical contact. * **Convection** occurs when heat flows from one body to another through a fluid. It only occurs in expandable fluids, as it depends on the density difference that results when a part of a fluid increases in temperature. The heated portion of the fluid becomes less dense due to expansion and is displaced by a denser fluid located above the heated region. The heated portion emits its heat by radiation and by conduction to its surroundings in the new location. This method of heat transfer is known as convection. At absolute zero temperature, there is no heat and, as we've already mentioned, the molecules are at rest. In all calculations involving temperature, the value above absolute zero is always used and therefore the minus sign doesn't appear in lower readings. Absolute zero is approximately -273 °C below zero on the Celsius scale. ##### COLD The term cold is often used incorrectly. It's neither a substance nor a complete property in itself, but simply an absence or a reduction in heat. When we say "become colder", we mean heat is removed or escapes. ##### HEAT FLOW To produce refrigeration—that is, the removal of heat—there must be a heat flow from the substance to be cooled. This heat flow or heat transfer occurs only if there is a cooling medium to which the heat can flow at a lower temperature than that of the substance being cooled. The rate of heat flow depends on the temperature difference between the two substances, on the surface area of the substances to and from which the heat flow occurs and on the resistance to the flow of thermal insulation that may exist between the two substances. ## METHODS OF REFRIGERATION There are several ways to produce refrigeration, the most common being: * **Using ice** * **Using freezing mixtures** * **Using dry ice** * **Using volatile liquids** * **Using Ice** A limited degree of refrigeration is obtained placing the substance to be cooled near blocks of ice. Heat flows from the substance to the ice, thereby cooling the substance and causing the ice to melt. This method only allows for a moderate amount of cooling and it’s not long-term. The traditional ice-box is an example of this application. * **Freezing Mixtures** Freezing mixtures, or chemical refrigerators as they are sometimes called, operate on the principle of the absorption of latent heat when two solids chemically combine to form a liquid. In the case of ice and salt, this is the heat required to change ice into water without a change in temperature. Freezing mixtures have no commercial application but are widely used in laboratories where the production of low temperatures is needed quickly. Freezing mixtures consisting of a mixture of ice and an excess of a chemical salt operate as follows. The ice melts, absorbing the heat from its surroundings, and a salt solution forms which in turn dissolves more ice. Latent heat is required to change the ice into water and if the mixture is well isolated from its surroundings, this heat can only come from the mixture itself. The water formed dissolves more salt until an equilibrium is eventually reached where the concentration remains constant. And as long as the system is isolated from any external application of heat, a constant minimum temperature results. Some of the salts used are calcium chloride, sodium chloride, ammonium nitrate and ammonium sulfate. * **Dry Ice** This refrigerant is mainly used to preserve perishable goods in transit and for any other application where the melting of ordinary ice is unacceptable. The most common type of mobile ice cream cart is an example of this form of refrigeration. The dry ice manufacturing process (homogeneous carbon dioxide) is as follows: Dry and clean carbon dioxide is compressed to high pressure in a compressor. After it is purged from any traces of oil collected in the compressor, it passes into a condenser to reduce its temperature. It then liquefies and is suddenly expanded through nozzles in a chamber. The rapid expansion of carbon dioxide results in snowflakes that are compacted into blocks or sheets for later use. * **Volatile Liquids** The most common method to commercially produce cold is by vaporizing liquids with extremely low boiling points. The operation depends on the fact that all liquids, when they evaporate, absorb latent heat from their surroundings and thereby produce a cooling effect. Boiling water is a simple illustration of this; refer to Figures 1 to 4. The heat applied to water increases its temperature and eventually causes it to boil at 100°C at normal atmospheric pressure. Further application of heat does not increase its temperature, just causes it to evaporate. In other words, to evaporate, the water must absorb heat from an external source, which is then cooled. The boiling point of different liquids varies widely, even if they are at the same pressure. This pressure factor is important because the boiling point depends on the pressure - the higher the pressure, the higher the boiling point and vice versa. If the boiling point of a liquid is given without any reference to the pressure, it is always assumed that the pressure is normal atmospheric pressure, that is 101.3 kPa. The relationship between pressure and boiling point applies to refrigeration. By selecting a liquid with a low boiling point, it absorbs the heat necessary to vaporize it from the substance which is to be cooled. The degree of cold required depends on the pressure applied to the liquid to vaporize it. The pressure can be modified by modifying the pressure and therefore the temperature at which the liquid vaporizes. ##### REFRIGERATION PROCESS A common refrigerant is anhydrous (water-free) pure ammonia. An excellent and clear representation of the process in a refrigerator is achieved by considering a quantity of this substance during the transfer from the liquid to the vapor state. For example, suppose we have an open container filled with liquid ammonia, as shown in Figure 5. At atmospheric pressure and temperature, it boils and its temperature is -34 °C simply because the boiling point of liquid ammonia at atmospheric pressure is -34 °C. Since this temperature is significantly lower than that of the surrounding air, heat flows to the ammonia and it continues to boil until it has completely evaporated. However, as the boiling process is taking place, the air immediately surrounding the container is cooled as heat flows to the ammonia. When all of the ammonia has evaporated, boiling ceases, heat flow stops, and the cooling effect ends. Obviously, using liquid ammonia in this manner to produce refrigeration commercially is not at all economical. First, an unlimited supply of liquid ammonia would be necessary; second, there is no control over the degree of cooling around the container—the closer you are, the colder it gets; and lastly, there is no control over the degree of cold produced in a specific area. ![img1] When heat is added to water at atmospheric pressure at sea level, the water boils and its temperature remains at 100°C. ![img2] If the vapor pressure above the water is increased, the boiling point temperature increases. For example, at a pressure of 350 kPa, the boiling point temperature is 138.88 °C. ![img3] When water is heated at the summit of a high mountain, it boils at a temperature lower than 100°C because the pressure on the water is less than the pressure at sea level. A decrease in pressure signifies a decrease in the boiling point temperature. ![img4] When the steam from a boiler fills a radiator, heat flows from the steam to the surrounding air. If the steam in the radiator is at atmospheric pressure, it condenses and the temperature of the steam and the water is 101.3 °C. In other words, the boiling point temperature and the condensation point temperature are the same for a given pressure. A certain improvement is achieved by placing the container in a closed and insulated box and taking steps to vent the ammonia vapor to the atmosphere, as shown in Figure 6. The item to be kept cold is placed in the box and since only the inside of the box and the item inside are cooled, much less ammonia is driven off by boiling. ![img6] It may be that the item in the box doesn’t need to be cooled to a temperature as low as -34 °C, which is the boiling point of ammonia. By restricting the flow of ammonia vapor to the atmosphere, it is possible to build up pressure in the container and thereby increase the boiling point of ammonia. For example, if the pressure in the container is increased to 163.4 kPa, the boiling point is -23.3 °C. The resulting decrease in the temperature difference between the inside and the outside of the container means that less heat flows to the container to be absorbed by the ammonia and consequently less ammonia evaporates. By restricting the flow of steam to the atmosphere, as shown in Figure 7, the refrigeration temperature is raised and the rate of evaporation reduced. ![img7] As we have mentioned, this type of arrangement for commercial refrigeration requires an unlimited supply of ammonia. However, the process requires that the vapor be collected and returned to its liquid form by an external source. It can then be reused repeatedly. Beyond economy in converting ammonia vapor back to liquid, other factors require that it not escape freely and continuously to the atmosphere. In particular, since the vapor is penetrating, it has a strong, unpleasant odor and is very dangerous. There are two methods for recovering ammonia vapor, the compression system and the absorption system. Each system depends on the fact that the process of transforming a liquid into a gas by the application of heat is reversible. That is, the gas is returned to a liquid by the removal of heat. ![img5] When heat is added to liquid ammonia at atmospheric pressure at sea level, the ammonia boils and its temperature is -34°C instead of 100°C as is the case with water. ## COMPRESSION VAPOR SYSTEMS In the compression system for recovering ammonia, its compression temperature must be slightly higher than the temperature of the available refrigerant, typically water. This is achieved mechanically by increasing the vapor pressure using a compressor, as shown in Figure 8. As the cold vapor is compressed, its temperature increases as a result of the work done on it. ![img8] This increased temperature allows the refrigerant (water) to remove the heat and, by maintaining the pressure, the ammonia gas condenses back into a liquid. This condensed ammonia evaporates again when it returns to the container in the insulated box and the cycle continues. The simple example given above uses ammonia as a refrigerant and a reciprocating compressor to increase the pressure. This ammonia compression system is common in industrial refrigeration plants; it’s covered in the next lecture. There are numerous other refrigerants frequently used (see the notes further down) and the pressure change can be achieved by reciprocating, centrifugal or jet compressors. In each case, the vapor compression refrigeration cycle follows a diagram very similar to that shown in Figure 9. The four main components of the equipment are the expansion valve, the evaporator, the compressor and the condenser. * **Rotary Compressors** are used when the pressure ratio is low, such as in small household refrigerators. They are also used in large low-pressure units or the supercharged units of a multi-stage system, similar to that shown in Figure 3 of Lecture 4 of this section. Diagrams (a) and (b) of Figure 10 illustrate the operation of two types of rotary compressors: (a) the sliding vane type uses a vane on a shaft with an eccentric and (b) the sliding vane type. Rotary compressors have high volumetric efficiency and are relatively vibration-free. Figure 11 shows the operation of the vapor compression refrigeration cycle using a rotary compressor, such as in a household refrigerator. Diagrams (a) and (b) illustrate the progressive steps of the cycle. * In (a), the system is off, the liquid refrigerant is contained in the evaporator, the capillary tube and the condenser. The evaporator temperature is lower than that of the condenser and contains most of the liquid. The remainder of the system is filled with refrigerant gas. The pressures in the system are balanced. * In (b), a quantity of heat enters the evaporator, resulting in the vaporizing of the refrigerant at low pressure and the rotary compressor is turned on. The compressor is of the sliding vane type, similar to that shown in Figure 9 (a), with a spring-loaded vane that separates the suction and the discharge ports. In position (b), the suction chamber between the piston and the vane is very small when the discharge chamber is completely closed. * In (c), the piston moves forward, expanding the suction chamber and reducing the discharge chamber. The compression of the gas opens the discharge valve and the gas starts to flow to the condenser. In (d), the process continues, more gas is discharged to the condenser. * In (e), the piston approaches the end of the compression and the gas in the condenser starts to condense. (Note that the diagrams show the rotor of the compressor during the progressive revolutions of the rotation; compression and discharge of the gas occur evenly over several rotations.) * In (f), the piston is at top dead center, discharge is complete, the discharge valve is closed and the cylinder is filled with low-pressure vapor. The next revolution compresses and discharges this vapor. ## CENTRIFUGAL COMPRESSORS The pressure build-up in the condenser is now sufficient to cause a flow of liquid refrigerant from the condenser through the capillary tube to the evaporator. The capillary tube restricts flow sufficiently to keep the condenser pressure above that of the evaporator and high enough to allow condensation with air as the refrigerant. As the liquid refrigerant flows through the capillary tube, there is a pressure drop and some of the liquid flashes to vapor. This flashing in the evaporator further expands the liquid to vapor with a consequential cooling effect on the surrounding areas. A centrifugal compressor is illustrated in Figure 12. Figure 13 illustrates the progressive steps in the operation of a vapor compression refrigeration cycle using a centrifugal compressor. Here, compression is achieved by centrifugal force, using the same principle as a centrifugal fan. Here, the pressure difference obtained is lower than that required for positive displacement and refrigerants that require such a high degree of compression. The centrifugal compression systems are usually designed so the condenser, evaporator and the compressor are mounted on a single base. The vapor compression cycle using a centrifugal compressor from Figure 13 is described below. * In (a), the system is off, the liquid refrigerant is in the cooler, which can also be called the evaporator. The condenser is of the shell-and-tube type, with the cooling water flowing through the tubes. The cooler is also of the shell-and-tube type, with the brine flowing through the tubes. The brine is used to transport heat from the load to the evaporator and is called the secondary refrigerant of the system. * In (b), the brine circulation is switched on, the heat from the brine evaporates some of the liquid refrigerant and it flows towards the suction of the centrifugal compressor. * In (c), the compressor is switched on and draws refrigerant from the cooler at inlet suction pressure to the compressor. The compressed refrigerant vapor is discharged to the shell of the condenser. * In (d), the cooling water flow through the condenser tubes starts and the condensation of the refrigerant vapor begins. * In (e), the condensed liquid returns by gravity to the cooler shell for re-evaporation by the heat extracted from the circulating brine. The liquid level in the cooler is maintained by a float valve. The system is now in operation. ## JET REFRIGERATION A jet can be used to produce the pressure differential required for a compression system. Figure 15 shows its application in a water jet refrigeration system. Water jet vapor compression systems have been successfully employed in small and large capacities for air conditioning. Steam nozzles propel the suction vapor from the evaporator of a water jet expansion valve where the chilled water is vaporized and consequently cooled. The mixture of refrigerant vapor and the motive steam is compressed in the jet or supercharger. The condenser, which is of the surface or barometric type, requires an evacuator for initial venting and the removal of air due to leaks, and a condensate pump to return or discharge the condensate. ![img15] The jet in Figure 15 produces the pressure differential needed for the vapor compression refrigeration cycle. The jet removes refrigerant vapor from the evaporator chamber and controls the evaporator pressure. In this system, the fluid used to operate the jet is mixed with the refrigerant vapors and its design is very basic if these two fluids have exactly the same properties. Therefore, it is advantageous to use steam as the driving fluid of the jet and water as the refrigerant. Steam-driven water is supplied to the main jet and the air jets in the main condenser. The chilled water used for the circulation (the refrigerant) is cooled as it flows through the evaporator by its own partial evaporation under low pressure conditions. The condensate from the condenser (jet steam and chilled water vapor) is returned to feed the boiler. The drawbacks of the system are the difficulty of control and poor economy. The jet is inefficient as a compressor and therefore the condenser capacity must be large. However, the initial equipment cost is relatively low and the system is useful where the water and steam supply are sufficient and at low cost. ## AIR CYCLE REFRIGERATION We need to mention the use of air as refrigerant in compression refrigeration. Air is the only substance used in commercial refrigeration that remains in a gaseous state throughout the cycle. Air was used as a refrigerant in some of the first refrigeration machines. However, compared to the vapor compression cycle, the air cycle has a very low coefficient of performance and the high power requirements that follow have caused it to be removed from commercial use. There is one exception however—the air conditioning systems of modern aircraft where air cabin supercharging is used and the low weight of the machinery is a significant advantage. The air cycle operates by compressing and expanding the air charge. Expansion causes a drop in temperature and the cold air is discharged for direct cooling (as in an open system) or returned for recompression (as in a closed system). The expanding air can drive an air motor and thereby reduce the power required by the compressor. ## ABSORPTION VAPOR SYSTEMS As we had already mentioned in the development of simple refrigeration cycles, ammonia vapor is collected and re-converted for repeated use using a compression cycle or an absorption cycle. Examples of different types of compression systems have been given. In many of them, the refrigerant used is not ammonia, however, the principle of operation remains the same—pressure is applied to the refrigerant vapor until its condensation temperature is greater than that of the refrigerant source. It's then free to condense into liquid, removing heat, and ready to be reused. Note that compression takes place on the refrigerant when it is in a gaseous or vapor state. The work requirement of a refrigeration cycle is reduced if compression is done on the refrigerant when it is in liquid form. The absorption cycle provides a method of achieving this. It is identical to the vapor compression refrigeration cycle, except the compressor is replaced by a separate absorption cycle where the secondary fluid circulates. In Figure 16, the refrigerant vapor leaving the evaporator is absorbed into the secondary fluid in the absorber, raised to condenser pressure by the pump, then separated from the secondary fluid by the application of heat in the generator. ![img16] The refrigerant vapor (now at a higher pressure) enters the condenser, the expansion valve and the evaporator in turn, as in the compression process. The secondary fluid circulates only in the absorption cycle. The generator operation in the absorption cycle requires a generous supply of heat; even though its work requirement is less than that of the compression cycle, its total energy requirement is greater. Therefore, the absorption cycle is applied where large amounts of heat at low cost are available. ![img17] Figure 17 illustrates a simple example of an absorption system using ammonia as the refrigerant. This system relies on the fact that anhydrous ammonia is readily absorbed by water. The ammonia gas from the container passes into an absorption chamber where it is absorbed by water. The strong water-ammonia solution that results is pumped to a generator. It is heated in a coil or by another suitable means, whereupon the ammonia gas is driven off under pressure. The gas passes into a condenser where it liquefies for subsequent additional use, and the remaining weak water/ammonia solution is returned to the absorber. The ammonia absorption system has significant commercial importance and, under specific conditions, is a cost-effective method of refrigeration, particularly where waste heat, such as exhaust steam, can be used to power the generator. ![img18] Figures 18 (a), (b), (c) and (d) illustrate the principle of operation and Figures 19 and 20 show the external look of an absorption system that uses water as the refrigerant and lithium bromide as the secondary fluid. The water absorption system operates at low absolute pressure, allowing water to evaporate at 4°C (saturation temperature which is equivalent to 6 mm Hg of absolute pressure). This cooling effect of the temperature is applied to the cooling water that circulates. This system is suitable for air conditioning applications where a cooling water temperature between 4°C and 7 °C is required. The entire system in the machine, including the evaporator, absorber, generator and condenser, must be maintained at a high vacuum to ensure efficient and economical operation. To achieve this by removing non-condensable gases, a purge unit consisting of a vacuum pump in the form of a jet is installed. ![img19] ![img20] ![img21] Figure 19 (a) shows a schematic of the water absorption system and (b) its external view. Figure 20 provides an isometric view of the unit. Notice that the condenser and the generator are grouped in one vessel, and the evaporator and the absorber are both located in separate vessels as well. These machines can produce between 25 and 1000 tons of refrigeration. <start_of_image> Schematic of a hermetic absorption chiller. All absorption units are provided with purge systems to remove non-condensable gases, but they are not designed to withstand leaks. The logical approach is to prevent leaks in the design, by incorporating the equipment with the least number of fittings possible. For example, by enclosing the unit entirely in a single housing, it is possible. The diagram illustrates the condenser, evaporator, absorber and the generator all enclosed in a single body. The pumps are built without external seals, like submersible pumps; they can therefore be considered hermetic. ## HERMETIC DESIGN OF A CENTRIFUGAL MACHINE The principle of hermetically sealing all of the equipment to simplify operation at pressures below atmospheric, is also applied to water-cooled compression systems that use a centrifugal compressor. Figure 22 shows a cross-section of a centrifugal chiller used in air conditioning applications. This machine uses Freon 113 as a refrigerant, and operates completely under vacuum. ![img22] ## REFRIGERANTS When dealing with a simple refrigeration cycle, ammonia was selected as the representative refrigerant. However, there are a number of other substances that are also suitable as refrigerants. The general requirements of a refrigerant are as follows. It must be able to withstand continuous changes from the liquid state to the gaseous state and vice versa, which are required by the refrigeration process. The operating pressures at which these take place must be suitable for the design and construction of the machine. Its properties, physical, thermodynamic and chemical, must be suitable for a cost-effective and safe design. The ideal refrigerant has a high latent heat, a low volumetric weight, a high critical temperature and a low boiling point. It’s cheap and readily available, non-toxic, non-flammable and non-corrosive, and allows for easy detection of leaks, and operates within reasonable pressure limits. No single refrigerant has all of these properties. The choice will therefore be based on those properties that are essential to the specific application. Refrigerants have been listed by the American Society of Heating, Refrigerating and Air Conditioning Engineers. Tables of properties are published in their data books and guides. Their standardized designation includes about 30 refrigerants, classified according to the following groups: <start_of_image>* **Halocarbon compounds (halogenated group)** * Fluorinated hydrocarbons (freons) * Chlorinated hydrocarbons (methyl chloride, methylene chloride) * **Organic Compounds** * Methane, ethane, propane, butane, and others. * **Inorganic Compounds** * Ammonia, water, air, carbon dioxide, nitrous oxide, sulfur dioxide. * **Azeotropes (fluid mixtures)** A standardized number is assigned to each refrigerant. For example, the refrigerant no. 717 is ammonia, no. 718 is water, no. 729 is air, no. 744 is carbon dioxide, no. 12 is dichlorodifluoromethane (CCl2F2) or Freon 12, no. 22 is monochlorodifluoromethane (CHCIF2) or Freon 22. Of these refrigerants, a small number are used in most applications. Ammonia, methyl chloride and carbon dioxide are the main refrigerants used in large and medium-sized refrigeration and cold storage facilities. Sulfur dioxide was previously used in smaller facilities, but has been replaced by Freon. The development of Freons has been of great importance to the refrigeration industry. The Freon series offers a refrigerant suitable for virtually any practical application. Table 1 shows the relative efficiencies of refrigerants. These values are based on operation in a typical refrigeration cycle, i.e., a cycle where evaporation and condensation occur at -15°C and 30°C respectively. They are sometimes called the ton figure (see Lecture Five of this section, under the heading Refrigeration Calculations). ## AMMONIA (NH3) Anhydrous ammonia is by far the most commonly used refrigerant. However, it is not suitable for small refrigerators like those often used for domestic purposes due to its penetrating odor which is unpleasant and irritating to sensitive membranes in particular, the eyes. A highly desirable characteristic of a refrigerant is a high latent heat of vaporization, as this is a measure of the refrigeration effect that can be obtained from it. Ammonia has a high latent heat of vaporization, namely 1370 kJ/kg at atmospheric pressure. Another desirable characteristic is high density. This permits the use of a small compressor that requires low power output. Keep in mind that the size of the compressor and its power output depend on the volume of refrigerant flowing through the system. Ammonia also possesses this second desirable characteristic, i.e., 1 kg of gas at atmospheric pressure occupies a volume of 1.138 m³ (density 0.879 kg/m³). Ammonia is very soluble in water. At normal ambient temperatures, water absorbs 900 times its own volume of ammonia gas. At normal ambient temperatures, liquid ammonia is very volatile and this facilitates purity tests. Simply pour a little liquid ammonia into a test tube and bring it to a boil. Any impurities remaining in the test tube are easily detected. At normal atmospheric temperatures, ammonia does not easily burn in air, but when heated, it forms a yellowish-green flame. However, when heated to over 870°C, it decomposes into its constituent gases, nitrogen and hydrogen. Under certain conditions, explosive mixtures form, and particularly if oil vapor is present. Therefore, ammonia should never come into contact with red-hot metal or an open flame. Pure ammonia in liquid or gaseous form does not attack metals. However, in the presence of water, ammonium hydroxide forms, which is very corrosive to copper, brass and, to some extent, steel. Therefore, it is essential that any ammonia present in a refrigeration system is completely anhydrous (dry, or free of water). *Refrigeration effect* – Refer to Table 1 and the section on refrigeration calculations in Lecture Five of this present section. ## CARBON DIOXIDE (CO2) Carbon dioxide is a well-known gas. It is a heavy, odorless and colorless gas that turns into a snow-like substance called dry ice at a pressure of 3450 kPa and 0°C. It exists in water to a certain extent. It is not dangerous to breathe, except in large quantities. Its only hazard therefore lies in the lack of oxygen. It does not irritate the membranes of the body, which is why it is often used as a refrigerant in hospitals and at sea. Carbon dioxide is non-flammable and does not support combustion. It has no effect on copper, copper alloys, iron or steel. It is very slightly soluble in water, forming carbonic acid (H2CO3). The solution has a very acidic taste. The solubility of CO2 in water depends on the temperature of the water—the higher the temperature, the less gas dissolves and vice versa. The density of carbon dioxide is high. Therefore, its refrigeration effect per given volume is also high. In fact, a refrigeration machine using carbon dioxide as refrigerant can use a compressor cylinder that is only one-fifth the displacement required to achieve the same amount of cooling with ammonia. Because of its very low boiling point, carbon dioxide must be compressed to very high pressures. Consequently, the compressor cylinder, the condenser and the connecting pipework are much stronger than for ammonia. However, it is not used in the majority of refrigeration systems since it is much more expensive to produce than ammonia. ## SULFUR DIOXIDE (SO2) Sulfur dioxide is a colorless vapor with a pungent and obnoxious odor. It is a very stable vapor used in smaller refrigerators, but has been widely replaced by Freon. It is not flammable, so there is no risk of explosion if it is used as a refrigerant. In its pure state, it is not corrosive to copper, copper alloys, zinc, iron or steel. However, in the presence of water, sulfuric acid forms, which attacks copper, zinc and iron. Therefore, its water content must be kept below 0.3 % by volume. A refrigeration machine that uses sulfur dioxide requires three times greater compressor cylinder displacement than one that uses ammonia as a refrigerant to achieve the same amount of cooling. It is heavier than air and so if a leak occurs, it forms a cloud that hugs the ground. ## ETHYL CHLORIDE (C2H5Cl) This vapor is very stable under normal atmospheric conditions and is therefore often used as a refrigerant in small household refrigerators. In its liquid state, it is colorless, highly volatile and has a sweet taste. Inhaling enough ethyl chloride can lead to unconsciousness and death. Its odor is pungent and resembles that of ether. An air/ethyl chloride mixture containing between 5% and 14% of ethyl chloride, when exposed to a spark, will ignite explosively. Mixtures containing proportions outside those percentages are non-flammable ## METHYL CHLORIDE (CH3Cl) Methyl chloride is light per unit volume. The compressor cylinders that use it as a refrigerant are therefore large compared to those that use ammonia or sulfur dioxide. In fact, they have to be twice as large as those that use sulfur dioxide and seven times as large as those that use ammonia, to produce a similar amount of refrigeration. Sulfur dioxide is not corrosive to copper, copper alloys, iron or steel, a characteristic that makes it very attractive as a refrigerant. Methyl chloride is a colorless vapor with an agreeable odor that resembles chloroform. It is easily liquefied when compressed and cooled. It is a very stable product, which makes it practical for smaller refrigerators. Its boiling point at atmospheric pressure is -23°C. Small quantities of methyl chloride mixed with air have little effect on the lungs or eyes, but when the percentage rises above 10 % by volume, the mixture that results causes unconsciousness and death by suffocation. Although this tendency to cause unconsciousness is not a drawback, it makes the product less safe than sulfur dioxide, which is immediately recognizable by its odor. Methyl chloride is soluble in water in the ratio of three volumes of gas to one volume of water under normal atmospheric conditions. The presence of water in methyl chloride is evident by the formation of ice on the expansion valve. Methyl chloride is flammable, but it does not burn easily. It is explosive only when mixed with air in specific proportions and exposed to a spark or an open flame. The explosive range is between 10% and 15% by volume of methyl chloride in air. Copper, copper alloys, iron and steel are all resistant to attack by methyl chloride. Rubber, however, is soluble in both liquid and gaseous forms of methyl chloride and should not be used for packing or other applications. Asbestos that is specially treated or lead is more satisfactory. Compressor cylinders for methyl chloride have to be smaller than those for sulfur dioxide to provide the same amount of refrigeration. They have to be larger than those for ammonia. ## METHYLENE CHLORIDE (CH2Cl2) Methylene chloride, sometimes called dichloromethane, is a colorless liquid under normal atmospheric conditions. It has a sweet, agreeable odor that resembles chloroform. Its boiling point is 40°C and its latent heat of vaporization is 316 kJ/kg. It does not form explosive mixtures with air; in vapor form, it extinguishes combustion. It does not react with mild steel, copper, aluminum, lead or tin, though it reacts slightly with brass at 80°C. ## FREON The group of hydrocarbons used as refrigerants, including ethane